Climate Change Exacerbated Dalbergia sissoo Dieback Under Water Stress and Ceratocystis fimbriata Infection
Asif Javed Muhammad1,2*, Izhar Ul Haq1, Nauman Ghafoor1 and Fazal Ur Rehman1
1Department of Forestry and Range Management, University of Agriculture Faisalabad, Faisalabad Pakistan
2Centre for Advanced Studies, Agriculture Food Security (CAS - AFS), University of Agriculture Faisalabad, Faisalabad Pakistan
3Pakistan Forest Institute, Peshawar, Khyber Pakhtun Khwa (KPK), Pakistan
4Alpha Genomic Laboratories, Islamabad Pakistan
Submission: February 25, 2023; Published: March 17, 2023
*Corresponding author: Asif Javed Muhammad, Department of Forestry and Range Management, Centre for Advanced Studies, Agriculture Food Security (CAS - AFS), University of Agriculture Faisalabad, Faisalabad Pakistan Email: azurefromheavens@gmail.com
How to cite this article: Asif Javed Muhammad, Izhar Ul Haq, Nauman Ghafoor and Fazal Ur Rehman. Climate Change Exacerbated Dalbergia sissoo Dieback Under Water Stress and Ceratocystis fimbriata Infection. Agri Res & Tech: Open Access J. 2023; 27 (4): 556376. DOI: 10.19080/ARTOAJ.2023.27.556376
Abstract
Dalbergia sissoo is a native tree species of the Indo-Pak and is one of the most important timber-producing species used in the agroforestry, fuelwood, and furniture industries. Historically widespread mortality of shisham due to dieback was observed during an El Niño cycle characterized by low rains and high temperatures. This is the first report that has successfully established the role of water deficit in shisham dieback under Ceratocystis fimbriata infection. Seedlings were either predisposed to water deficit for two weeks before fungal inoculation or subjected to both stresses i.e., biotic, and abiotic at the same time. The imposition of water deficit and the availability of water at the time of inoculation was determined by the rate of plant development, lesion growth, physiological parameters such as (stomatal conductivity, transpiration rate, photosynthesis rate, water potential, water use efficiency), chlorophyll contents, carotenoids, and oxidative enzymes such as POD, CAT, and H2O2. Water-stressed plants showed dieback symptoms and more susceptibility to fungal infection than well-watered plants clearly demonstrating water deficit is the primary cause of shisham dieback. Inoculated plants responded to stress by decreasing stomatal conductance, transpiration rate, and photosynthetic activity due to low water potential. The growth rate was also affected due to low chlorophyll and carotenoid content. D. sissoo reacts to stress by increased production of CAT and POD, enzymes. This study emphasizes the importance of exploring the interaction of drought x disease in shisham and the primary physiological responses involved in plant growth.
Keywords: Dalbergia sissoo; Climate Change; Drought, Ceratocystis fimbriata, Physiological & Biochemical Responses, Photosynthetic rate, Xylem cavitation & embolism
Background
Climate change is resulting in the frequency and intensity of climatic anomalies such as drought and flooding (Choat et al. [1], McDowell et al. [2]), and tree mortality associated with intense droughts are becoming more prevalent in drought-prone ecosystems [1-3]. Drought causing widespread tree dieback has a pronounced impact on the composition, function, and ecosystem services, making forest sustainability a significant concern under warming and dry climatic conditions [3-5]. Information regarding important physiological processes and tree response to long-term high temperatures and water deficit conditions is valuable in determining the tree growth and decline risk under future dry and warm climatic conditions [6,7]. Long-distance water transport in the xylem is critical for physiological functioning because it governs leaf gas exchange [8,9]. Upward water flow in xylem conduits is mostly drawn by transpiration-generated negative pressure and, therefore, remains stable, and increasing xylem tension during drought causes cavitation, thus compromising hydraulic network stability [10,11]. Embolism spreading throughout the xylem will significantly lower hydraulic transport efficiency and result in serious hydraulic dysfunction, which has been identified as a primary physiological mechanism leading to drought-induced tree death [12]. As a result, a detailed understanding of the patterns of tree growth in response to extreme drought, as well as the underlying xylem hydraulic processes, would provide a scientific framework for designing rational forest management approaches in water-stressed areas under climate change.
The Dalbergia genus is a pantropical group of approximately 250 species and a diverse group in Asia, Central and South America, Africa, and Madagascar (Kile [13], Hartvig [14]) Dalbergia sissoo is one of Pakistan's most significant and widely planted tree species. It is locally known as “shisham or talli” and is renowned for its high-quality wood, colour, tensile strength, texture, grain, and other physical-mechanical properties [14]. It offers a very important raw material for a wide range of wood-based industries, especially furniture, and housing. Wood is also preferred for heating and cooking due to its high calorific value. No other species of wood, except teak, are so extensively planted in India and Pakistan.
Although the species is native to Pakistan, serious challenges are speculated in planting, establishment, and forest productivity under changing climate scenarios [15,16] as hot and prolonged summers and low water availability are becoming more frequent in Pakistan. Plants are typically sedentary and adapted to cope continuously with different forms of biotic and abiotic stresses [17]. Climate change includes several factors such as drought, flooding, and high temperature that have an interconnected effect on plant health. It is therefore essential to understand these factors as products of plant health interactions to forecast global change [18]. Droughts are occurring both at a high rate and in severity, therefore, reducing the time needed for plants to react to such anomalies. Plant reactions to water deprivation have therefore been commonly considered and found to be dynamic, interactive, and subject to time, duration, and strength of stress [19,20]. Plant reactions to water deficiency cause physiological, biochemical, and molecular reactions [21]. These mechanisms were not studied in D. sissoo and how they interact with both biotic and abiotic factors such as fungal pathogens and water deficit. Pathogen outbreaks change plant physiological processes such as primary and secondary metabolism, as well as the induction of the defence mechanism, affecting plant growth and development [22]. Similarly, the outcome of plant-pathogenic interactions can be affected by the history of abiotic stress predisposition that plants have accumulated during their life cycle. Water scarcity can affect plants’ physiological status by altering their susceptibility to abiotic factors and favoring pathogen infection [23]. Abiotic stress predisposition to disease in plants is well known [20,24] and the challenge of ever-changing climate change means that a deeper understanding of these mechanisms is extremely important for disease management [24].
Ceratocystis fimbriata, a member of the Ceratocystis genus, is known to infect a wide variety of tropical and subtropical tree species [13,25-27]. However, very little is known about the interactive role of C. fimbriata and water deficit in relation to the incidence of shisham dieback in Pakistan. Secondly, different responses both physiological and biochemical that are triggered by D. sissoo seedlings under these stresses are not known. Given the socioeconomic significance of D. sissoo and the urgent need for research into tree response to multiple stresses, the role of drought stress, including simultaneous and water deficit predisposition in D. sissoo under Ceratocystis fimbriata and its implications on growth, disease, biochemical and physiological changes, was investigated.
Material and methods
Climate data on annual precipitation (mm) and temperature (oC) for Pakistan was downloaded from https://climateknowledgeportal.worldbank.org/download-data for a period ranging between 1981 and 2018 (Figure 1).
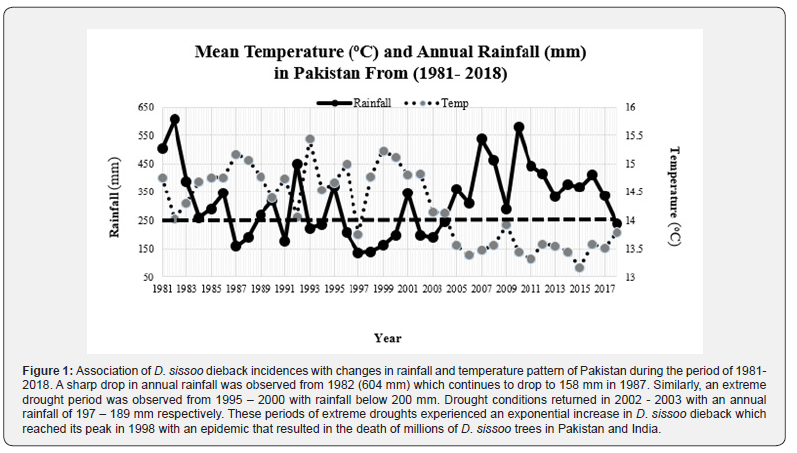
Plant Materials
The open-pollinated seeds of D. sissoo were randomly collected from different mother trees along with the natural range of the Himalayan Khyber Pakhtunkhwa (KPK) province of Pakistan. The area is considered a center of origin of D. sissoo. The mountain range forms narrow valleys with a subtropical environment. The area is mainly fed with freshwater received mainly from rain or from melting snow. Floods are not known due to hilly topography. Locally, the climate is subtropical, with high rainfall, temperatures, and humidity. The soil is sandy loam with good drainage and EC<0.5 dS m-1.
D. sissoo seedlings were raised from the pods by soaking in water overnight and planted into pots containing sandy loam soil at Nursery Farm of Forestry, University of Agriculture Faisalabad, Punjab. Seedlings were watered regularly till the age of 6 months.
Ceratocystis fimbriata culture
The Tree Pathology Laboratory at the Centre for Advanced Studies, Food Security Agriculture (CAS-AFS), Faisalabad University of Agriculture, Faisalabad, Pakistan, provided a reference culture of Ceratocystis fimbriata GenBank accession #MG971390. The pure culture was incubated at 25°C on 2 percent malt extract agar (MEA) comprising 20 g L-1 malt and 20 g L-1 agar (Biolab, Midrand, South Africa).
Pathogen inoculation
C. fimbriata pure culture was utilized for seedling inoculation. For inoculation, mycelial plugs (about 5 mm in diameter) were taken from the borders of 1-week-old cultures. Before inoculation, the stem surface was disinfected with 70% ethanol. A shallow "I" incision was performed at the base of the stem (5 cm above the surface of the soil) using a sterile scalpel to remove the bark and expose the cambium. In each of the wound-facing incisions, a mycelial plug was introduced [28]. To avoid desiccation, the initial length of the incision was measured, and the cut site was sealed with parafilm.
Wounded stems from control plants were similarly handled, except that they were infected with sterile MEA plugs devoid of fungus. To confirm Koch's postulates, the fungus was re-isolated from each infected plant at the conclusion of the experiment. Wood tissues were taken from the lesions and immersed in 5% hypochlorite for 2 minutes before being soaked in 70% ethanol, rinsed in sterile distilled water, washed dry on sterile filter paper, and plated on MEA medium. Plants that have just been infected with MEA have also been isolated. After incubation at 25oC, the fungus was described based on morphological properties.
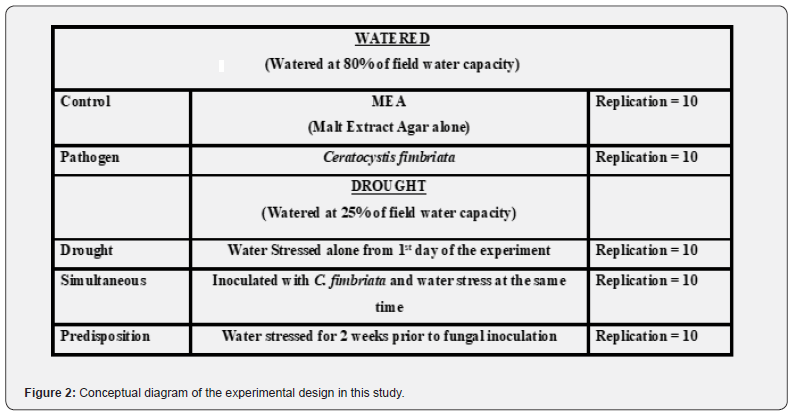
Experimental Design
The experiment was laid out in a completely randomized design with two levels i.e., water and drought. For drought, two levels i.e., simultaneous water deficit and fungal inoculation second predisposition to water deficit for two weeks before inoculation. Control treatments also contained two levels i.e., seedlings subjected to water deficit alone, secondly, seedlings watered and inoculated with MEA alone (Figure 2). Seedlings were watered at 80 percent field water capacity in the case of well-watered seedlings and those subjected to water deficit were maintained at 25 percent water field capacity. In the case of a drought predisposition, the seedlings were watered at 25 percent of the soil water field capacity for the first 14 days and inoculated with C. fimbriata after that seedlings were watered at 80 percent soil water field capacity. Each treatment is represented by 10 seedlings. Seedings were kept and maintained in a net house under natural environmental conditions.
Monitoring of Plants
Seedlings were monitored regularly, and observations of external symptoms including leaf color changes and wilting were recorded. However, data on fungal infection, plant growth, and physiological and biochemical traits were collected after two months of treatment.
Growth parameters
Plant height was measured in inches on the day of inoculation and again on the last day of the experiment. The plant's growth rate was calculated by subtracting the final and initial heights.
Monitoring infection
External signs such as foliar chlorosis and wilting were monitored on a regular basis. The growth of the stem lesions (mm) was calculated after 2 months. The final increment in lesion development was the difference between the initial and the final lesion growth.
Osmotic Potential and Water Potential
Fresh leaf tissues were sampled at 6:00 a.m. and kept at O°C for three to four days. Leaf tissues were thawed at room temperature for 30 to 45 minutes to obtain cell sap. The osmotic potential of the leaves was determined using an Osmometer and a 50 ml volume of cell sap [29]. The leaf water potential was calculated using a pressure chamber (Plant Moisture Stress, PMS Instruments) following the method of [30].
Pigment Determination
Photosynthetic pigments were extracted using cold acetone‐50 mM Tris buffer pH 7.8 (80:20 v:v) following the protocol of Sims & Gamon's [31]. The readings were taken at 470, 537, 647, and 663 nm wavelengths using PerkinElmer Spectrophotometer (Perkin Elmer). Concentrations of total chlorophyll, chlorophyll a (Chla), b (Chlb), and carotenoids were determined using Sims and Gamon's [31] formulae.
Antioxidant enzyme assays
Superoxide (SOD) was determined in a 2.0 ml reaction hermetically sealed tubes of reaction solution containing the disodium salt of 100 M Ethylenediamine tetraacetic acid (Na2 EDTA), 20 M -Nicotinamide adenine dinucleotide reduced (NADH), and 20 mM sodium phosphate buffer, pH 7.8 [32]. A chromatography syringe was used to introduce 100 µL of 25.2 mM epinephrine in 0.1N HCl into the process. The samples were incubated at 28 °C for 5 minutes while being shaken. Following that, the segments were removed, and a reading was taken at an absorbance of 480 nm in a PerkinElmer Spectrophotometer (Perkin Elmer). The blank was used under identical conditions, but with no plant tissue. With a molar absorption coefficient of 4.0 x 103 M-1, the amount of accumulated adrenochrome was utilized to compute the amount of superoxide anion generated [33,34]. To quantify hydrogen peroxide, leaf samples were weighed on a 0.0001 g precision balance, ground in liquid nitrogen, and homogenized in 2.0 mL of 50 mM potassium phosphate buffer, pH 6.5, containing 1 mM hydroxylamine, then centrifuged at 10,000 g for 15 minutes at 4 °C, and the supernatant was collected [35]. Aliquots of 100 µL supernatant were added to a reaction medium containing 250 M ferrous ammonium sulfate, 25 mM sulfuric acid, 250 M xylenol orange, and 100 mM sorbitol in a final volume of 2 mL, homogenized, and maintained in the dark for 30 minutes [36]. Absorbance was measured at 560 nm using a PerkinElmer Spectrophotometer (Perkin Elmer)., and H2O2 was quantified using a calibration curve with peroxide concentrations as a benchmark.
Photosynthetic rate, Transpiration Rate, Stomatal conductance, Water use efficiency
IRGA portable equipment was used to evaluate photosynthetic rate (μmol m-2 s-1), stomatal conductance (mmol m-2 s-1), water usage efficiency, and transpiration rate (mg H2O m-2 s-1) (Infra-Red Gas Analyzer, Li 6200, Licor). The measurements were obtained about midday on five randomly chosen leaves for each treatment.
Data Analysis
All parameters were submitted to a two-way analysis of variance (ANOVA), and significantly different treatment means were compared using Tukey's test, using the R package "agricolae" [37].
Result
Pathogenicity Trials
Stem lesions, foliar chlorosis, and wilting were not the same for all treatments. As expected, water-stressed plants wilted with severe leaf chlorosis, and more than 80 percent experienced mortality. Plants inoculated with MEA alone and watered appeared healthy. Similarly, plants watered and inoculated with C. fimbriata looked healthy and asymptomatic. Large-sized lesions occurred around the inoculation site of those plants that were water-stressed and inoculated concurrently or predisposed to water deficit before inoculation (Figure 3).
Generally, seedlings subjected to water and fungal stress showed a 30-60 percent mortality rate. A high mortality rate of 60 percent was observed in seedlings subjected to both stresses simultaneously, followed by predisposed seedlings with a 30 percent mortality rate. C. fimbriata was re-isolated from the lesions and was morphologically identified, thus confirming Koch's postulates. The lesion growth caused by C. fimbriata was significantly different (Figure 4). Maximum lesion growth (43.0 mm) was observed in seedlings that were inoculated after water limitation imposition than on plants inoculated simultaneously to water stress imposition (21.8 mm) respectively (Figure 4 & Table 1). Nonetheless, a very restricted lesion growth (6.6 mm) was observed in seedlings inoculated and watered (Figure 4 & Table 1).
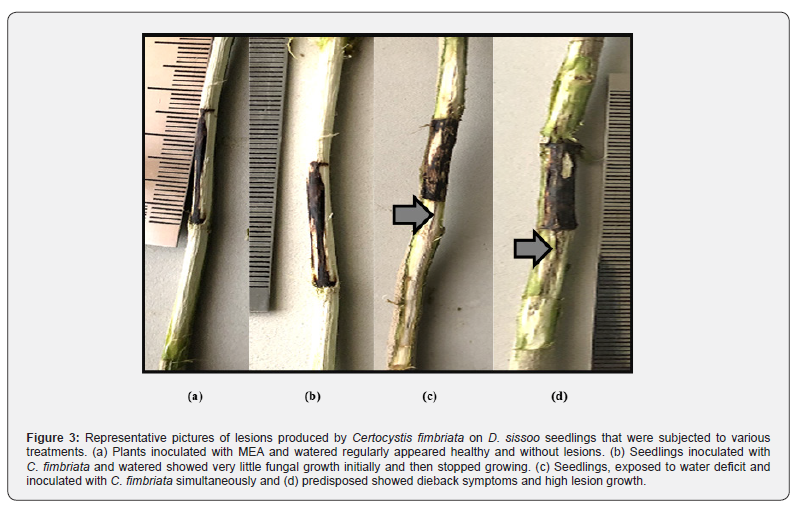
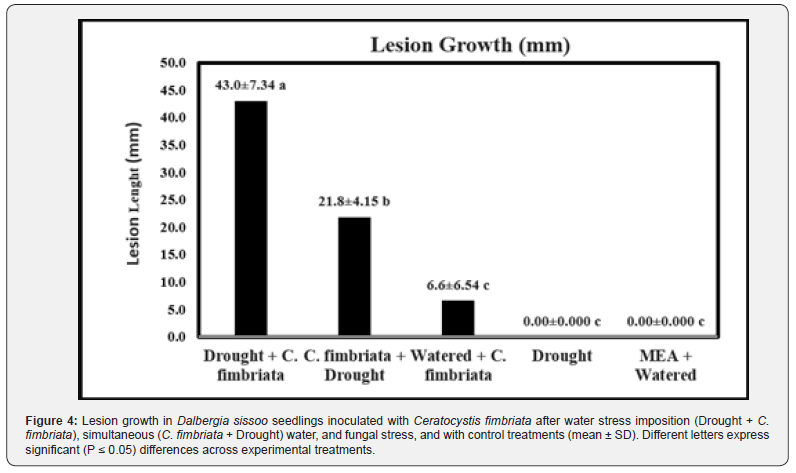
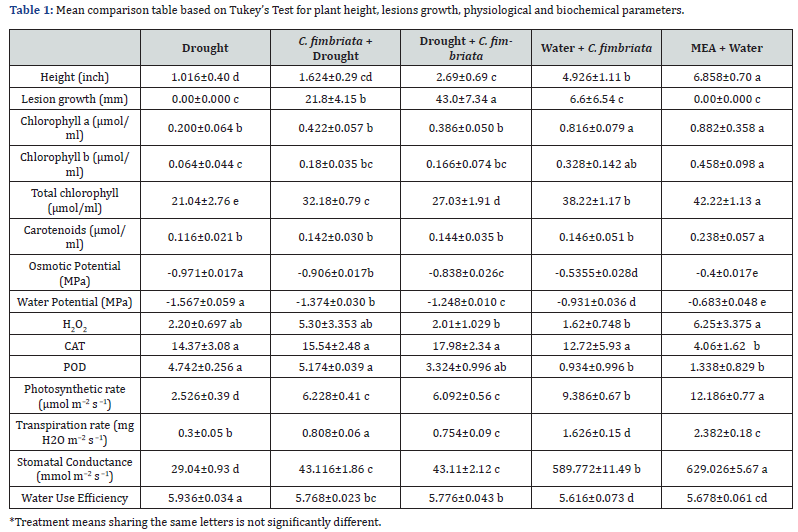
Morphophysiological parameters
Growth
Plant growth was negatively impacted under water deficit alone or when it was combined with fungal inoculation (Table 1). All well-watered seedlings resumed normal growth whether inoculated or not, while water-stressed and inoculated showed a significant reduction in plant growth (Table 1). Nevertheless, predisposed seedlings showed better growth than those that were continuously stressed and inoculated.
Photosynthetic pigments
The pigment concentration varied substantially depending on the drought treatment and the water condition at the time of inoculation (Figure 5a & Table 1). Control plants showed healthy green leaves and thus exhibited high chlorophyll content followed by those inoculated and watered. Chlorophyll content was negatively affected by drought alone in treatments combined with fungal inoculation. Lowered chlorophyll content caused low photosynthetic activity and mortality of the seedlings toward the end, both in drought alone and when combined with fungal inoculation. Similar effects were observed on Chlorophyll a and Chlorophyll b contents (Figure 5b, 5c & Table 1) which were mainly affected in seedlings with water retention alone or in a combination of both stresses. Concerning carotenoids, all treatments had a significant effect as compared to control plants (Figure 5d & Table 1).
Antioxidant enzyme assay
Water deficit alone or when combined with pathogen had significantly affected POD activity (Table 1). Maximum POD activity was observed in seedlings stressed and inoculated as compared to control or inoculated and watered (Table 1). POD activity ranged from 3.0 - 4.84 as compared to 1.338 in control plants. On the contrary, water and fungal stresses had a significantly different effect on CAT activity as compared to POD (Table 1). High CAT expression was observed in seedlings that were predisposed to water deficit, followed by simultaneous and water deficit alone respectively. Minimum CAT activity was observed in control seedlings indicating CAT has a significant role in protecting cellular functions under stress/s. Changes in H2O2 content were significant after water deficit and pathogen application. Water deficit alone or in combination with C. fimbriata had a significant effect on H2O2 (Table 1). Generally, treatments having high CAT expression showed low H2O2 content indicating both are negatively correlated and CAT has a significant role in protecting the cells from H2O2 damage.
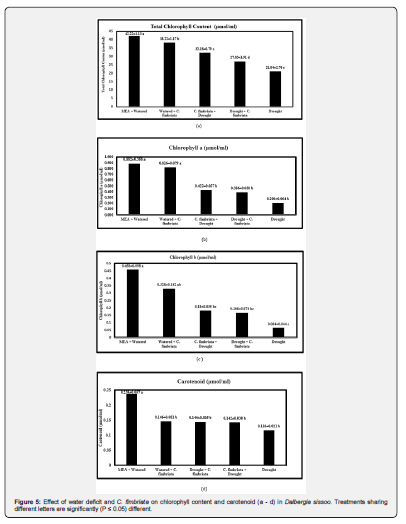
A maximum drop in water potential was observed in control seedlings that were deprived of water for two months. Seedlings inoculated after two weeks of water imposition showed significantly lower negative water potential as compared to plants inoculated simultaneously to water stress imposition (Table 1). Similarly, we found a statistically significant difference in stomatal conductance (Table 1). All treatments with water stress alone or in combination with fungal inoculation showed a significant drop in stomatal conductance as compared to those watered respectively (Table 1). With reduced stomatal conductance a minimum transpiration rate was observed in seedlings exposed to drought, predisposed, and simultaneous as compared to control seedlings respectively. The high photosynthetic rate was observed in seedlings with high water availability i.e. control and the lowest was observed for seedlings under constant drought stress. Similarly, low photosynthetic activity was observed in seedlings predisposed and inoculated with C. fimbriata, and high activity was observed in seedlings watered x inoculated simultaneously (Table 1). Drought stress seedlings exhibited the maximum amount of water use efficiency, followed by predisposed and seedlings inoculated and stressed simultaneously (Table 1), this was comparable to control and seedlings inoculated and watered regularly.
Discussion
This is the first study reporting the interaction between abiotic (water stress) and biotic factors (fungal inoculation) in Dalbergia sissoo susceptibility to dieback. The results indicated that changes in morphophysiological parameters were mainly regarded due to water deprivation in association with fungal inoculations. Furthermore, water-stressed plants showed susceptibility to fungi as compared to those which were watered normally. Lesion on plants inoculated after two weeks of water deprivation was larger than on those which were stressed and inoculated at the same time. However, a high mortality rate was observed in seedlings with simultaneous exposure to water and fungal stress. Seedlings inoculated and watered daily did not show dieback symptoms and lesion growth, thus indicating a positive association between drought and dieback. Diebacks and declines are diseases that are mainly caused by the predisposing effects of biotic and abiotic factors, resulting in the attack of secondary agents such as fungi [38]. Drought is possibly considered to be the most important predisposing factor followed by the secondary action of facultative parasites common to the natural environment.
A very low dieback incidence in shisham was reported in Abbassia plantation Mukhtar et al. [39] situated in the southernmost province of Punjab with a desert-like environment. The plantation water table is maintained by the canal running through the plantation ensuring a continuous supply of water to the rhizosphere. These findings were supported by a field study on shisham in Haryana, Karnal Forest Division, where a high rate of dieback was observed in coupes where irrigation was stopped as compared to those which were normally irrigated and did not show any dieback symptoms [40], thus affirming again the role of water stress in causing dieback in shisham. Abiotic variables have been shown to have a role in producing Neofusicoccum eucalyptorum sensitivity to Eucalyptus globulus dieback in many different tree species, including eucalypts [24]. The findings suggested that plants that were already water-stressed (predisposition) were more susceptible to N. eucalyptorum infection than non-stressed plants.
Shisham dieback reached its epidemic form in 1998 in Pakistan [39]. A look into the meteorological data strongly associated this epidemic with six years of extreme drought which started in 1995 and ended in 2001 (Figure 2). This period was also referred to as El Nino and it has negatively impacted tree species globally. The amount of rainfall received during these years was 370 mm in 1995 which reached to a low level of 197 mm in 2000 respectively (Figure 2). A survey carried out of different ecological zones of the Punjab, Pakistan, during 2005 - 2009, reported a very high dieback incidence in 2005 ranging from 6.6 to 50 percent and a follow-up survey of the same zones in 2009 revealed a marked reduction in the disease intensity that ranged from 2.2 to 8.3 percent respectively [39]. The severity and decline in dieback explained can be associated with a three years-long drought that started in 2003 and ended in 2005 (Figure 2) [39]. Another study on the cause of tree dieback suggested that extreme climatic fluctuation plays an important role in forest dieback [41]. With few exceptions, water stress has been implicated as a primary factor in all major diebacks. Extreme weather events such as flooding, heavy rains, drought, and wet/dry oscillations have been related to the onset of Eucalyptus diebacks in Australia (White 1968). Climatic fluctuations particularly those in rainfall were the primary cause of forest diebacks in Australia (White 1968). Extreme fluctuations in moisture such as waterlogging and flooding have also been reported to be responsible for a high incidence of dieback in shisham [42-46]. Generally, D. sissoo develops an extensive taproot system at an early age, allowing the trees to obtain moisture from the subsoil and thus flourish under semiarid conditions. The taproot may partially die or may grow to a greater diameter than the bole. However, sissoo has a more elaborate lateral root system that spreads for long distances from the tree. Waterlogging, flooding, semi-flooding, and drought may cause damage or mortality to the roots thus exposing them to secondary fungal pathogens. Intermittent droughts would increase the root decaying process in sissoo exacerbating the dieback. Waterlogging caused 73.1 percent of the disease, while high irrigation caused 13.8 percent of the disease, similarly, flooding, semi-flooding, degraded soil, uncared ploughing, high density, and moisture resulted in 27.4, 52.1, 27.6, 30.2, and 41.1 percent incidence, respectively [46].
Abiotic stress changes the physiological status of plants making them favorable to pathogens or diseases [23]. It has been observed that water stress on woody plants makes them more susceptible to fungi [47,48]. Similarly, the effect of a latent pathogen Diplodia sapinea was reported to be more aggressive under water stress to red pines, and its incidence in the Quercus robur [49]. Eucalyptus grandis seedlings when inoculated under water stress, reported the appearance of lesions and necrosis after one week of inoculation, and canker development was induced after three weeks of inoculation [50]. The present study results are in concurrence with that, as C. fimbriata showed more lesion growth under drought stress as compared to watered plants under infection. These findings established the idea that abiotic factors may be the primary cause of sissoo dieback which is followed by the attack by secondary agents such as facultative fungal agents. Availability of water or avoiding flooding may help sissoo withstand secondary attacks by fungi and resume normal growth by avoiding damage to its roots. Predisposition factors such as waterlogging and extended periods of drought would weaken its defense mechanism against later stress [51].
When plants were inoculated and stressed at the same time, there was a significant difference in morphophysiological status compared to plants that were predisposed and inoculated separately. The influence of water stress timing and shisham physiological status on pathogen infection susceptibility can be seen in the high lesion growth, plant growth rate, and biochemical response. Many previous studies have found that pathogens cause more damage to stressed weakened plants [47,48]. After the morphophysiological level, it was observed that water stress was the major factor that most impacted plant performance, with different degrees of intensity depending on the treatment applied. Drought alone and drought x pathogen stress significantly affect photosynthetic parameters. Stomatal conductance decreased in all the treatments except the well-watered sissoo plants. The reduced photosynthesis rate observed seemed to be due to reduced stomatal conductance or due to inhibition of metabolic phenomenon [52-55]. The earliest response of plants to drought stress is stomatal closure for drought-induced suppression, as the CO2 diffusion into the leaves decreases due to stomatal closure and thus results in decreased photosynthesis [52]. Under normal growth conditions, plants direct their energy to cellular maintenance, growth, defense, and reproduction. However, plants going under stressful conditions such as fungal attacks or water stress need to balance energy production and plant defense to survive under these stressful conditions [17,56]. Thus transferring energy resources from growth to defense results in a reduction of photosynthetic activity, which is a general consequence of pathogen attack [56]. Our results here support this trade-off strategy (low plant growth and decrease in photosynthetic activity, low chlorophyll pigments). Similarly, the effect of drought on the physiology of D. latifolia, and D. sissoo suggested that stomatal closure is the main cause of drought-induced suppression in photosynthesis [54]. The effect on the rate of photosynthesis in both species under drought stress was due to both stomatal and non-stomatal limitations and photo-inhibition might have played some role in this decline. Similarly, a physiological comparison between healthy and dieback-affected shisham trees revealed that diseased tree leaves were damaged due to some stress factors. Gas exchange attributes remarkably differed in pre- and post-drought inoculated seedlings compared to the well-watered seedlings. This showed that the photosynthesis rate decreased under water stress due to the stomatal closure. Stomatal closure could be occurred due to the direct effect of water deficit or due to blockage of the xylem caused by fungal mycelium.
There was a marked decrease in total chlorophyll content, chlorophyll a & b, and carotenoids in sissoo when subjected to drought and pathogen inoculation. A maximum decrease observed might be due to a lower rate of synthesis and rate of breakdown in plant systems [52,57]. The other reason could be excessive damage to chloroplast which might be responsible for the reduction in chlorophyll and carotenoids (Mitra 1998). It appears that drought alone or drought x pathogen inhibits the biosynthesis of chlorophyll and carotenoids that break down the pigments leading to the reduction in their content thereby affecting the photosynthetic activity.
Higher leaf water potential values may be associated with decreased photosynthesis, decreased stomatal conductivity, and transpiration to limit water loss, or with physical changes in pathogen-induced plant cell wall components [17]. The low water potential observed upon inoculation in predisposed shisham plants may be favorable for fungal colonization. Botryosphaeria dothidea colonization capacity increased with decreasing water potential because host stem lesions appeared only when plant water potential was low (-1.2 to -1.3 MPa) [59].
The study of plant-pathogen interactions is a dynamic process in which two species, the host, and the pathogen, compete to overcome each other's protective barriers. Plants can detect and activate defensive systems even when they are a significant distance away from the pathogen inoculation site [60,61]. The activation of ROS, which causes redox imbalance and oxidative stress and is poisonous to the attacker but may impede the normal cell function of host cells, is one of the first lines of defense against biotic and abiotic stress [62,63]. Similarly, fungal pathogens cause the release of antioxidant enzymes such as catalase and peroxidase as a pathogenicity mechanism [38]. Decreased H2O2 in plants inoculated during water deprivation was observed because of the development of antioxidant enzymes such as CAT by the ROS scavenge fungus, thus increasing its colonization success. Sherwood et al. [48] proposed that drought induces accumulation of hydrogen peroxide (H2O2) in Austrian pine (Pinus nigra) shoots which is toxic for fungi, but canker pathogen D. sapinea causes a substantial reduction in H2O2levels in water-deprived plants. The same phenomenon was observed during the in vitro application of H2O2, in which the fungus reacted to this high level of oxidative stress by increasing the development of catalase and peroxidase, leading to significant degradation of H2O2 [48].
The enhanced production of the CAT in the fungal and water-stressed plants showed the natural defense response of the plant. In plants the CAT is a major harmful oxygen species scavenging enzyme, it decomposes the H2O2 during oxidative stress and thus maintains the redox balance [49]. Peroxidase (POD) is frequently associated with plant defense mechanisms against pathogens. A very low level of POD was observed in normal plants as compared to those exposed to drought or predisposed inoculated seedlings. The main function of POD in plants is to extract H2O2from the tissues and contribute to the development of phenolic compounds, e.g., lignin [25,64]. POD is also responsible for the formation of intermolecular bonds during the organization of the cell wall at pathogen-infected sites [48,56]. Peroxidase often participates in the synthesis of ethylene, the concentration of which also increases in the pathogenesis phase and enhances its activity following a pathogen attack [25,65-70]. The tolerance of plants to ROS in response to drought stress involves the adaptation of several complex and manifold mechanisms, such as the enzymatic antioxidant system [19,57]. These include a substantial increase in the activity of these expressed enzymes shown in the argan tree (Argania spinose) under drought stress conditions, suggesting their function as an efficient antioxidant defense line against oxidative damage caused by ROS [57, 71-80]. Activation of ROS enzymes tends to be well associated with the degree and severity of stress. Drought stress increased the activity of antioxidants such as CAT and SOD [46]. Both enzymes are the most essential components of ROS protection systems. Most studies documented differential responses of antioxidant and antioxidant metabolites to drought stress in some plant species [25,29, 81-90].
Conclusion
Dalbergia sissoo is a tropical species that originated in the valleys of the Himalayan foothills of Indo - Pak subcontinent. These valleys are characterized by high rainfalls, riverine habitats, and low flooding. River Sindh and the water table in general are fed by fresh water from rain or melting snow. D. sissoo populations thus evolved under high water regimens, low flooding, and low salinity. D. sissoo was introduced to semi-arid regions of Pakistan which received very low rainfall annually. Moreover, D. sissoo was mainly planted along the roadsides, canal banks, farmer fields, or in plantations. These plantation sites heavily depend on rainfall for their water needs. Disease surveys reported high dieback incidences along the roadsides, and canal banks and the lowest on farmer fields. Dieback incidence was severe on canal sides used for transportation that cause soil compaction thus limiting water supply or root approach to canal water. A similar situation was observed on roadsides where water tables would be lowered under extreme drought conditions. D. sissoo trees planted on watercourses were mainly infected with dieback due to root damage caused by exposure to water in a situation like waterlogging. D. sissoo dieback cases were severe in those plantations with limited water supply as compared to those had a constant supply of water that ensures high water table. The period that saw the dieback epidemic was mainly marked by low rainfall and high temperature that lasted for almost six years. This prolonged drought severity caused low rainfalls that resulted in the lowering of water tables and similarly, canals also faced water shortages to supply water to the plantations. This prolonged water shortage caused severe damage to the D. sissoo external and internal structure, caused energy imbalance due to low photosynthetic activity, reduced immunity, restricted growth, attracted secondary pathogens such as fungi that caused serious damage at the cellular level, and finally, insect pests burrow in the damaged softwood result into tree mortality. However, when drought conditions were receded many D. sissoo plants which were showing early or advanced dieback symptoms recovered and resumed normal growth. Based on these field observations and knowledge we have first time demonstrated that prolonged water deficit is the main cause of shisham dieback, fungi and insects then follow the infections if drought conditions are dragged on. Our findings, therefore, emphasize the importance of understanding the relationship between biotic and abiotic factors on D. sissoo productivity, especially considering the current climate-change scenario, which predicts an increase in both drought stress and pathogen attack. Water deficit causes changes at the physiological and biochemical levels reducing energy levels thus compromising plants’ ability to grow and combat external challenges. It is therefore important to develop strategies to ensure water supply during through water conservation or to develop genotypes that can tolerate drought through efficient utilization of available water resources.
Acknowledgment
Dr. Muhammad Asif Javed (P.I.) also would like to thanks Professor Dr. Iqrar Ahmad Khan (T.I.) for providing a laboratory facility at the Center for Agriculture Security (CAS), UAF, and for his insight and unwavering support. P.I. also would like to acknowledge the immense support from Mr. M. Bilal Zia, Forest Geneticist at Pakistan Forest Institute (PFI), Peshawar, KPK Pakistan, and Fazal Ur Rehman my graduate student in making field surveys possible in Khyber Pakhtunkhawa (KPK) and Punjab, Pakistan.
References
- Choat B, Brodribb TJ, Brodersen CR, Duursma RA, López R, et al. (2018) Triggers of tree mortality under drought. Nature, 558(7711): 531-539.
- McDowell NG, Michaletz ST, Bennett KE, Solander KC, Xu C, et al. (2018) Predicting chronic climate-driven disturbances and their mitigation. Trends in ecology and evolution 33(1): 15-27.
- Allen CD, Macalady AK, Chenchouni H, Bachelet D, McDowell N, et al. (2010) A global overview of drought and heat-induced tree mortality reveals emerging climate change risks for forests. Forest ecology and management 259(4): 660-684.
- Breshears DD, Cobb NS, Rich PM, Price KP, Allen CD, et al. (2005) Regional vegetation die-off in response to global-change-type drought. Proceedings of the National Academy of Sciences 102(42): 15144-15148.
- Walker X, Johnstone JF (2014) Widespread negative correlations between black spruce growth and temperature across topographic moisture gradients in the boreal forest. Environmental Research Letters 9(6): 064016.
- Pellizzari E, Camarero JJ, Gazol A, Sangüesa Barreda G and Carrer M (2016) Wood anatomy and carbon isotope discrimination support long term hydraulic deterioration as a major cause of drought induced dieback. Global Change Biology 22(6): 2125-2137.
- Zhu L (2019) Response to climate change of vessel features and radial growth of four hardwood species from temperate forests of Northeast China. Northeast Forestry University Harbin.
- Lambers H and Poorter H (1992) Inherent variation in growth rate between higher plants A search for physiological causes and ecological consequences. Advances in Ecological Research 23: 187-261.
- Zwieniecki MA and Secchi F (2015) Threats to xylem hydraulic function of trees under “new climate normal” conditions. Plant Cell and Environment 38(9): 1713-1724.
- Tyree MT and Sperry JS (1988) Do woody-plants operate near the point of catastrophic xylem dysfunction caused by dynamic water stress answers from a model. Plant Physiology 88(3): 574-580.
- Tyree MT, Davis SD, Cochard H (1994) Biophysical perspectives of xylem evolution: is there a trade-off of hydraulic efficiency for vulnerability to dysfunction? IAWA journal 15(4): 335-360.
- Nardini A, Battistuzzo M and Savi T (2013) Shoot desiccation and hydraulic failure in temperate woody angiosperms during an extreme summer drought. New Phytologist 200(2): 322-329.
- Kile GA (1993) Plant diseases are caused by species of Ceratocystis sensu stricto. In: Chalara MJ, Wingfield KA, Seifert JA, Webber (Eds.), Ceratocystis and Ophiostoma: Taxonomy, Ecology, and Pathogenicity. APS, St Paul, Minnesota, USA, pp.173-183.
- Hartvig I, Czako M, Kjær ED, Nielsen LR, Theilade I (2015) The Use of DNA Barcoding in Identification and Conservation of Rosewood (Dalbergia spp.) PLoS ONE 10(9): 0138231.
- Javaid A, Bajwa R, Shah MBM (2003) Dieback resistance potential in different varieties of Shisham (Dalbergia sissoo Roxb) Mycopath 1: 105-110.
- Javaid A, Bajwa R, Anjum T (2004) Identification of some more varieties of shisham (Dalbergia sissoo Roxb) and their response to dieback and wilt. Mycopath 2: 55-59.
- Atkinson NJ, Urwin PE (2012) The interaction of plant biotic and abiotic stresses: from genes to the field. Journal of Experimental Botany 63(10): 3523-3543.
- Desprez-Loustau ML, Marçais B, Nageleisen LM, Piou D, Vannini A (2006) Interactive effects of drought and pathogens in forest trees. Ann For Sci 63(6): 597-612.
- Pyngrope S, Bhoomika K, Dubey RS (2013) Reactive oxygen species, ascorbate–glutathione pool and enzymes of their metabolism in drought sensitive and tolerant indica rice (Oryza sativa L) seedlings subjected to progressing levels of water deficit. Protoplasma 250(2): 585-600.
- Han RY, Gong XW, Li MY, Leng QN, Zhou YJ, et al. (2023) Combined tree-ring width and wood anatomy chronologies provide insights into the radial growth and hydraulic strategies in response to an extreme drought in plantation-grown Mongolian pine trees. Environmental and Experimental Botany 105259.
- Chaves MM, Maroco JP, Pereira JS (2003) Understanding plant responses to drought - from genes to the whole plant. Func Plant Biol 30(3): 239-264.
- Berger S, Sinha AK, Roitsch T (2007) Plant physiology meets phytopathology: plant primary metabolism and plant-pathogen interactions. J Exp Bot 58(15-16): 4019-4026.
- Bostock RM, Pye MF, Roubtsova TV (2014) Predisposition in plant disease: exploiting the nexus in abiotic and biotic stress perception and response. Annu Rev Phytopathol 52: 517-549.
- Barradas C, Pinto G, Correia B, Castro BB, Phillips AJL, et al. (2018) Drought × disease interaction in Eucalyptus globulus under Neofusicoccum eucalyptorum infection. Plant Pathol 67(1): 87-96.
- Repka V, L Slovakova (1994) Purification, characterization and accumulation of three virus-induced cucumber peroxidases. Biologia Plantarum 36(1): 121-132.
- Poussio GB, Kazmi MR, Akem C, Fateh FS (2010) First record of Ceratocystis fimbriata associated with shisham (Dalbergia sissoo) decline in Pakistan. Australasian Plant Disease Notes 5(1): 63-65.
- Al-Adawi AO, Barnes I, Khan IA, Al-Subhi AM, Al-Jahwari, et al. (2013) Ceratocystis manginecans associated with a serious wilt disease of two native legume trees in Oman and Pakistan. Australasian Plant Pathology 42(2): 179-193.
- Khan S H, Idrees M, Muhammad F, Mahmood A and Zaidi SH (2004) Incidence of Shisham (Dalbergia sissoo Roxb.) Decline and In Vitro Response of Isolated Fungus Spp to Various Fungicides. Int J Agri Biol 6(4): pp611-614.
- Farouk S, Qados AAMS (2013) Osmotic adjustment and yield of cowpea in response to drought stress and chitosan. Indian J Appl Res 3(10).
- Roux J and Wingfield MJ (2009) Ceratocystis species: emerging pathogens of non-native plantation Eucalyptus and Acacia species. Southern Forests 71(2): pp115–120.
- Sims DA and Gamon JA (2002) Relationships between leaf pigment content and spectral reflectance across a wide range of species, leaf structures and developmental stages. Remote Sens Environ 81(2-3): 337-354.
- Mohammad M, Karr AL (2001) Superoxide anion generation in effective and ineffective soybean root nodules. Journal of Plant Physiology 158(8): 1023-1029.
- Misra HP, Fridovich I (1971) The generation of superoxide radicals during the autoxidation of ferredoxins. Journal of Biological Chemistry 246(22): 6886-6890.
- Boveris A (1984) Determination of the production of superoxide radicals and hydrogen peroxide in mitochondria. In Methods in enzymology Academic Press 105: 429-435.
- Kuo MC, Kao CH (2003) Aluminum effects on lipid peroxidation and antioxidative enzyme activities in rice leaves. Biologia Plantarum 46: 149-152.
- Gay C, Gebicki JM (2000) A critical evaluation of the effect of sorbitol on the ferric–xylenol orange hydroperoxide assay. Analytical biochemistry 284(2): 217-220.
- Tukey JW (1977) Exploratory data analysis. Addison-Wesley, Reading.
- Houston DR (1984) Stress-related diseases. Arboric J 8(2): 37-149.
- Mukhtar I, Bajwa R, Nasim G (2014) Trees survival exposed to dieback disease implies evolutionary modulation resistance in shisham (Dalbergia sissoo Roxb) In various agroecological zones of Punjab (Pakistan) Pak J Phytopathol 26(2): 289-300.
- Bakshi BK, Reddy MAR, Puri YN, Singh S (1972) Forest disease survey (Final Technical Report). For Pathol 1: 17.
- Auclair A, Worrest RC, Lachance D, Martin HC (1992) Global Perturbation as a General Mechanism of Forest Dieback', in P. D. Manion and D. Lachance (eds.), Forest Decline Concepts. American Phytopathological Society Press, St. Paul, Minnesota, USA.
- Bakshi BK (1954) Wilt of shisham (Dalbergia sissoo Roxb.) due to Fusarium solani Sensu Snyder and Hansen. Nature 174(4423): 278-91.
- Bakshi BK (1957) Wilt disease of Shisham. IV: The effect of soil moisture on the growth and survival of Fusarium solani in a laboratory. Indian Forester 83: 505-512.
- Bakshi BK, Singh SL (1959) Root disease of shisham (Dalbergia sissoo). VIII Inoculation studies on wilt. India For 85(7): 415-421.
- Bakshi BK (1995) Wilt diseases of shisham (Dalbergia sissoo Roxb.) The behavior of Fusarium solani, the wilt organism in the soil. Indian For 81: 276-281.
- Dayaram J, Kumar M, Sharma S, Chaturvedi OP (2003) Shisham mortality in Bihar: extent and causes. Indian Phytopathol 56: 384-387.
- Van Niekerk JM, Strever AE, Gerhard DT, Francois H, Paul F (2011) Influence of water stress on Botryosphaeriaceae disease expression in grapevines. Phytopathol. Mediterr 50(4): 151-165.
- Sherwood P, Villari C, Capretti P, Bonello P (2015) Mechanisms of induced susceptibility to diplodia tip blight in drought-stressed Austrian pine. Tree Physiol 35(5): 549-562.
- Ragazzi AS and Moricca DI (1999) Water stress and the development of cankers by Diplodia mutila on Quercus robur. J Phytopathol 147(7-8): 425-428.
- Pérez CA, Wingfield MJ, Slippers B, Altier NA, Blanchette RA (2009) Neofusicoccum eucalyptorum, a Eucalyptus pathogen, on native Myrtaceae in Uruguay. Plant Pathol 58(5): 964-970.
- Bruce TJA, Matthes MC, Napier JA, Pickett JA (2007) Stressful ‘memories of plants: evidence and possible mechanisms. Plant Sci. 173: 603-608.
- Flexas J, Escalona JM, Medrano H (1999) Water stress induces different levels of photosynthesis and electron transport rate regulation in grapevines. Plant Cell Environ 22(1): 39-48.
- Ashraf M, Ashraf MY, Khaliq A, Rha ES (2004) Growth and leaf gas exchange characteristics in Dalbergia sissoo Roxb and D. latifolia Roxb. under water deficit. Photosynthetica 42(1): 157-160.
- Chaudhry AK (2006) Abiotic and biotic stresses on shisham trees. In Third National Seminar on Shisham Dieback, Faisalabad, Pakistan, PFRI.
- Jung T, Nechwatal J (2008) Phytophthora gallica sp. nov, a new species from rhizosphere soil of declining oak and reed stands in France and Germany Mycol. Res 112(10): 1195-1205.
- Bilgin DD, Zavala JA, Zhu J, Steven JC, Donald RO, et al. (2010) Biotic stress globally downregulates photosynthesis genes. Plant Cell Environ 33: b1597-613.
- Chakhchar A, Wahbi S, Lamaoui M, Ferradous A, El-Mousadik A, et al.(2015) Physiological and biochemical traits of drought tolerance in Argania spinosa. J Plant Interact 10(1): 252-261.
- Mitra S, Das R, Mukherjee S (1998) Intramolecular proton transfer in inclusion complexes of cyclodextrins: role of water and highly polar nonaqueous media. J Phys Chem B 102(19): 3730-3735.
- Crist CR, Schoeneweiss DF (1975) The influence of controlled stresses on the susceptibility of European white birch stems to attack by Botryosphaeria dothidea. Phytopathol 65(4): 369-373.
- Eastburn DM, McElrone AJ, Bilgin DD (2011) Influence of atmospheric and climatic change on plant-pathogen interactions. J. Plant Pathol 60: 54-69.
- Bellincampi D, Cervone F, Lionetti V (2014) Plant cell wall dynamics and wall-related susceptibility in plant-pathogen interactions. Front Plant Sci 5: 228.
- Heller J, Tudzynski P (2011) Reactive oxygen species in phytopathogenic fungi: signaling, development and disease. Ann Rev Phytopathol. 49: 369-390.
- Del-Rio LA (2015) ROS and RNS in plant physiology: an overview. J Exp Bot 66(10): 2827-37.
- Pereira GJG, Molina SMG, Lea PJ, Azevedo RA (2002) The activity of antioxidant enzymes in response to cadmium in Crotalaria juncea. Plant Soil 239(1): 123-132.
- Sitbon F, Hennion S, Sundberg B, Little CH, Olsson O, et al. (1992) Transgenic tobacco plants coexpressing the Agrobacterium tumefaciens iaaM and iaaH genes display altered growth and indoleacetic acid metabolism. Plant Physiol 99(3): 1062-1069.
- Basu R (2009) High ambient temperature and mortality: a review of epidemiologic studies from 2001 to 2008. J Environ Health 8(1): 40.
- Amoroso MM, Daniels LD, Villalba R, Cherubini P (2015) Does drought incite tree decline and death in Austrocedrus chilensis forests? Journal of Vegetation Science 26(6): 1171-1183.
- Anderegg WR, Anderegg LD, Sherman C, Karp DS (2012) Effects of widespread drought‐induced aspen mortality on understory plants. Conservation Biology 26(6): 1082-1090.
- Anderegg WR, Schwalm C, Biondi F, Camarero JJ, Koch G, et al. (2015) Pervasive drought legacies in forest ecosystems and their implications for carbon cycle models. Science 349(6247): 528-532.
- Ayub G, Smith RA, Tissue DT, Atkin OK (2011) Impacts of drought on leaf respiration in darkness and light in Eucalyptus saligna exposed to industrial‐age atmospheric CO2 and growth temperature. New Phytologist 190(4): 1003-1018.
- Bakshi BK (1976) Principles in practice in Forestry. Forest Pathology FRI Press, PLO, FRI, Dehra Dun, India.
- Ball RA, Oosterhuis DM (2005) Measurement of root and leaf osmotic potential using the vapor pressure osmometer. Environ. Exp Bot 53(1): 77-84.
- Bashyal BP, Joshi S, Shrestha S (2002) Role of fungal endophytes on dieback of Dalbergia sissoo. NST 4(1).
- Bernt E, Bergmeyer HU (1974) Inorganic peroxidases. In: Bergmeyer HU (ed.), Methods of enzymatic analysis. Academic Press New York (4): 2246-2248.
- Chakhchar A, Ferradous A, Lamaoui M, Wahbi S, El-Modafar C (2011) Changes in antioxidant enzymes activity and oxidative damage in four Argania spinosa ecotypes under water stress conditions. Nat Proceedings 1-1.
- De Micco V, Carrer M, Rathgeber CB, Camarero JJ, Voltas J, et al. (2019) From xylogenesis to tree rings: wood traits to investigate tree response to environmental changes. IAWA journal 40(2): 155-182.
- Fielding J, Hall J (1978) A biochemical and cytochemical study of peroxidase activity in roots of Pisum sativum: I. a comparison of DAB-peroxidase and guaiacol-peroxidase with particular emphasis on the properties of cell wall activity. J Exp Bot 29(4): 969-981.
- Klitgaard BB and Lavin M (2005) Tribe Dalbergieae GP Lewis, BD Schrire, M Lock, B Mackinder (Eds.), Legumes of the World, Royal Botanic Gardens. Kew, pp. 307-335.
- Lawlor DW (1995) Photosynthesis, productivity and environment. J Exp Bot 46: 1449-1461.
- Liu X, Wang LP, Li YC, Li HY, Yu T, et al. (2009) Antifungal activity of thyme oil against Geotrichum citriaurantii in vitro and in vivo. J Appl Microbiol 107(5): 1450-1456.
- Nicholson RL, Hammerschmidt R (1992) Phenolic compounds and their role in disease resistance. Ann Rev Phytopathol 30(1): 369-389.
- R Core Team (2021) R: A language and environment for statistical computing. R Foundation for Statistical Computing.
- Ramegowda V, SenthilKumar M (2015) The interactive effects of simultaneous biotic and abiotic stresses on plants: mechanistic understanding from drought and pathogen combination. J Plant Physiol 176: 47-54.
- Scholander PF, Bradstreet ED, Hemmingsen EA, Hammel HT (1965) Sap pressure in vascular plants: negative hydrostatic pressure can be measured in plants. Sci 148(3668): 339-346.
- Sindhan GS and Parashar RD (1996) Biochemical changes in groundnut leaves due to infection by early and late leaf spot pathogens. Ind J. Mycol Plant Pathol 26(2): 210-212.
- Sturrock RN, Frankel SJ, Brown AV, Hennon PE, Kliejunas JT et al. (2011) Climate change and forest diseases. Plant Pathol 60(1): pp133-149.
- Van Wyk M, Adawi AOA, Khan IA, Deadman ML, Jahwari AA, et al. (2007) Ceratocystis manginecans sp. causal agent of a destructive mango wilt disease in Oman and Pakistan. Fungal Diversity 27: 213-230.
- Wargo PM (1996) Consequences of environmental stress on oak: predisposition to pathogens. In Ann D Sci For 53(2-3): 359-368.
- Wylie FR, Bevege DI (1980) Eucalypt Dieback in Queensland. In: Old KM, Kile GA, Ohmart CE (Eds.), Eucalypt Dieback in Forests and Woodlands, Proceedings of a conference held in Canberra, 4-6 August, 1980. CSIRO Division of Forest Research, Canberra, Australia, p. 31-36.
- Zhang L, Ma H, Chen T, Pe, J, Yu S, Zhao X (2014) Morphological and physiological responses of cotton (Gossypium hirsutum L) plants to salinity. PLoSOne 9(11): 112807.