Laboratory Soil Handling Affects CO2 Respiration, Amino-N and Water Stable Aggregate Results
William F Brinton*
Woods End Soil Laboratory, USA
Submission: April 13, 2020;; Published: April 23, 2020
*Corresponding author: William F Brinton, Woods End Soil Laboratory, Mt Vernon ME 04352 USA Email: will.brinton@woodsend.com
How to cite this article: Brinton W F. Laboratory Soil Handling Affects CO2 Respiration, Amino-N and Water Stable Aggregate Results. Agri Res & Tech: Open Access J. 2020; 24(2): 556262. DOI: 10.19080/ARTOAJ.2020.24.556262
Abstract
Laboratory analysis of soil traits associated with soil quality or soil health has gained popularity very recently reflecting a trend begun over a half-century ago scrutinizing farming systems for potentially deleterious soil effects. This renewed focus has brought into the forefront concerns about how laboratory soil handling may influence the observed traits. We find evidence in the literature that many but not all these concerns were addressed decades ago before the topic became of widespread interest but also before the soil testing industry modernized to high-throughput methods of soil homogenization not designed for soil quality investigations. Consequently, we find it necessary to retrace some steps in order to better characterize artifacts that may hamper objective evaluation of soil responses associated with soil health before conclusions of management effects of farming systems are drawn. Our study examined soils processed by a soil proficiency program by creating two size groups representing intensive and light soil disturbance and discerned differential effects of sieve sizes and moistening properties depending on which soil quality tests were used. The largest effects were due to wetting and not to sieving when comparing CO2 respiration, whereas sieving significantly influenced extractable amino-N and water stable aggregates. We found no evidence that a colorimetric thin-layer gel method (Solvita) for CO2 capture would lead to different conclusions when compared to an IRGA-infrared respirometer. It is recommended that future work continue to examine soil handling factors commonly employed for nutrient analyses which may be inconsistent with the goals of soil quality evaluation.
Keywords: Soil sieving, Soil health, CO2-respiration, Water stable aggregates
Introduction
Early work established that soil analyses oriented to biological traits could enhance the capability to distinguish short- and long-term management effects on soils due to differing farming systems [1]. Around this time, biological indicators including CO2 evolution were proposed as criteria for “soil biological activity” [2] and “healthy soil” [3]. This coincided with methodological developments for microbial biomass via CO2 respiration [4] and efforts including microbial respiration and enzyme activity to derive a “soil microbial index” [5]. Following this, enzymes and other microbiological traits were generally recognized for soil quality and farm-system evaluation [6]. A shift in some research focus to alternative agriculture, defined as farming systems employing non-conventional methods, resulted in new recommendations for soil biological measurements [7] and development of a “soil quality index” [8]. Deployment of such methods to evaluate farming system effects led to incorporating enzymes, earthworm counts and soil microbial respiration as routine analytes [9]. A recent meta-analysis of 149 paired farm comparisons employing biological soil tests including enzymes, basal CO2 respiration and soil biomass, based on CO2 respiration indicated that most were effective to reliably distinguish soil impacts in organic farming compared to conventional systems [10].
Much of the early work with soil CO2 respiration and soil biomass measurement employed variations of base trap titration [11-13]. This technique involved at first a direct method with BaOH2 to capture carbon dioxide as a BaCO3 precipitate, thence a two-stage modification substituting NaOH for BaOH2 due to liquid surface skin formation by BaCO3 which inhibits uptake of CO2 [14]. Essentially all later soil base-trap CO2 respiration methods lead from this alteration [14-17] excepting KOH used in a conductivity mode [38], a method recently reported to indicate significantly lower CO2 respiration than standard NaOH base trap methods [17]. There appears to be limited discussion on soil handling and preparation prior to conducting a respiration test in the body of early work. In the [18] textbook on soil analysis it is recommended to have soil quantity in the range of 10 - 70 g per analysis and moistened to attain 60% of water holding capacity (WHC), a test performed separately. Jäggi [2] proposed a modification of the Isermeyer method with fresh, moist soil rubbed through a 2 mm sieve to avoid effects of drying and conducted respiration at 50 – 70% of soil water holding capacity (WHC). To easily measure WHC Jäggi proposed soil-filled capillary tubes moistened from below by capillary to obtain 100% saturation before calculating adjustment to approximately 60% WHC. He also recommended that 20 g equivalent dry weight of soil would be satisfactory for 24 hr respiration measurements. Anderson [15] recommended 10 - 200 g of moist soil per analysis and includes no discussion on soil preparation. Koepf [13,20] and Beck & Röschenthaler [14] reported extensive trials on optimal moisture and temperature for conducting soil respiration analyses, concluding that moisture required for respiration exhibits a fairly broad optima range and that temperature must be carefully controlled. They also were possibly the first to report that pre-drying soils had a strong, stimulatory influence on CO2 rate similar to observations later by Birch [21] and point out that due to the partial pressure of CO2 a certain amount of the CO2 will remain in equilibrium within the soil pores along with abiotic CO2. In the updated edition of the SSSA soil monograph, Zibilske [22] compared NaOH with KOH, IRGA and GC methods and concluded that differing technologies to measure CO2 lead to characteristically different results but included no additional discussion on effects of soil handling prior to analysis. In a manner foretelling a predicament in obtaining ideal soil respiration conditions, Beck & Röschenthaler [14] observed sources of variance in respiration due to soil type, quantity of soil used, moisture level and length of incubation time and observed these factors exerted 5-times greater influence on variability of CO2 results than did the actual measurement technology (in their case, base trap titrimetry was compared to colorimetric CO2 absorption, the latter method similar to the Solvita test used in this study).
In our early work [23] we measured soil CO2-evolution by slight modification of the Isermeyer method using NaOH, whereby 100 grams of air dried, 2 mm sieved soil were moistened to 60% of water-holding capacity determined separately for each soil and samples incubated for 24 hrs and 7-days at 26 °C. For heavy, clayey soils from the Swedish site, manual hand crushing by mortar and pestle was the only mechanical crushing employed. We also found no difference in interpreting long-term soil management effects whether respiration was assessed in a 24 hr mode versus a 7-day duration. With significant renewed interest in soil respiration as an index for soil quality or soil health, which we define similarly, the impact that soil sampling, crushing, milling, homogenization and final sieving may exert on results becomes of greater significance. The potential artifact induced by soil processing prior to analysis for short-term respiration appears to be complicated, involving both sieve size and wetting properties, with particular issues related to over-saturation of soils due to over-grinding [24,25].
Bremner & Waring and Zöttl [26,27] may have first reported disturbances in soil respiration rate due to grinding and sieving. Zöttl prepared variously amended soils using mechanicallyground versus non-ground (5 mm sieving) and reported significantly higher CO2 respiration and lower N- mineralization due to grinding, suggesting a de-coupling of C: N mineralization rates due to soil disturbance, similar to Bremner and Waring’s findings. In contrast to current generalizations concerning the “CO2-burst” effect [28] which is often linked to predicting nitrogen mineralization, Birch [21] to whom early observations of the phenomena are generally ascribed provides evidence of two differing events accompanying soil rewetting; one being a rapid release of soluble organic matter (readily observed in soils containing organic matter as coloring of water following wetting) along with a resultant burst of metabolism and CO2 release due to this, but not associated with N-mineralization, and a second and simultaneous event of mineralization of embedded, surface organic matter as CO2, the latter being the only cycle of the two coupled to N-mineralization. If this is generally true, then the assumption that a CO2-burst C-min test is necessarily a test for N-min may be flawed.
Modern methods of soil testing commonly employed in commercial laboratories for soil nutrients include homogenizing soils by high-speed hammermill or flail grinding followed by multiple stages of vibratory sieving to < 2 mm fineness and sometimes < 0.5 mm [26]. These instrumentations have largely displaced earlier soil handling methods consisting of hand crushing, rolling and roller-brush processing. Wetting methods employed in incubation for CO2 respiration tests may include gravimetric additions to 60% WHC as previously described but more commonly today either capillary wetting [28] or volumebased wetting to meet 50% of water-filled pore space (WFPS) [16].
In studies involving 8 commercial soil labs employing biological test methods, soil wetting and soil fineness were observed to be implicated in moisture-saturation of soil samples, which reduced measured CO2 respiration, depending on the level of destructuring of soil [24]. Curiously, the popular and rapid Haney & Haney [24] wetting method recommended for preparing soil for 24 h CO2 respiration tests appears identical to the early Jäggi [2] method that employed a perforated cylinder for capillary uptake, but as a means to determine the saturation point for soil prior to adjustment. This suggests that in unadjusted form the Haney capillary method is certainly likely bring soils to saturation for respiration testing, yet was not noticed until recently. Somewhat more recent work indicates that soil handling influences the observed CO2 respiration through mechanisms related to structural condition of the soil [16,30]. This work brings back into focus the long-standing concern that biological C-mineralization and N-mineralization measurements may be significantly impacted by soil lab grinding and sieving, introducing artifacts associated with releasing soluble fractions or previously protected organic matter [26].
The hypothesis of this study is that soil handling actions prior to conducting soil quality tests may act singly or interactively to influence biological response especially to short-term respiration, defined as anything within the first 96-hours after re-wetting, and used as an indicator of soil microbial activity. Therefore, further pursuit of accurate reporting of biological soil investigations could be helped by careful scrutiny of soil handling. To evaluate these potentially related disturbance events, we conducted soil tests on samples variously handled and homogenized within a soil proficiency program [31]. Selected were 4 well-characterized soils from across the USA which were prepared specifically into two (2) sieve-size groups, “course” and “fine” and subsequently tested for biological test properties. To evaluate other traits associated with soil quality and which may be differently influenced by soil preparation and handling, we also measured water stable aggregates [35] and Solvita extractable amino-N [32].
Materials and Methods
Samples for this study included farm field soils from across the USA drawn and prepared by Utah State University Plants, Soils and Climate Department (PSC) which processes soils for the NAPT Soil Proficiency program. The normal regimen to produce fine, sieved soil < 2 mm was altered to produce the “coarse” and “fine” fractions. The basic preparation entailed 3 different steps to obtain the final samples [31]. Soil was initially air-dried followed by coarse-sieving at 2 mm. Soil that did not pass the 2 mm sieve was sent to a soil-crusher and then returned to the 2 mm sieve, joining the previously sieved sample. Therefore, the 2mm coarse soil included dried, fresh < 2 mm soil and some exposed to a crusher. To further prepare the fine < 0.8 mm sample the process continued with all < 2 mm blended soil sent to a 0.8 mm sieve. Soil not passing the 0.8 mm sieve was sent to a flail-mill and then returned to pass the 0.8 mm sieve. This material was blended to become the “fine” fraction.
The two fractions of each of four soil series were subsequently provided in coded bags to Woods End Soil Lab which ran duplicate tests on different days using two types of wetting for respiration evaluation: the rapid bottom-wetting capillary method [24] and a 50% WFPS method [15].
The Four (4) soils are noted below with location, NAPT code, soil series, and USDA Soil Classification are:
a. NH-120 Lovewell Very Fine Sandy Loam, Coarse-silty, mixed, superactive, frigid Fluventic Dystrudept
b. ME-112 Paxton Fine Sandy Loam, Coarse-loamy, mixed, active, mesic Oxyaquic Dystrudept
c. SD-102 Graceville Silty Clay Loam, Fine-silty, mixed, superactive, mesic Pachic Haplustoll
d. ME-101 Fryeburg Silt Loam, Coarse-silty, mixed, frigid Fluventic Dystrochrept
The test traits of these soils have been fully characterized, as follows (Table 1)
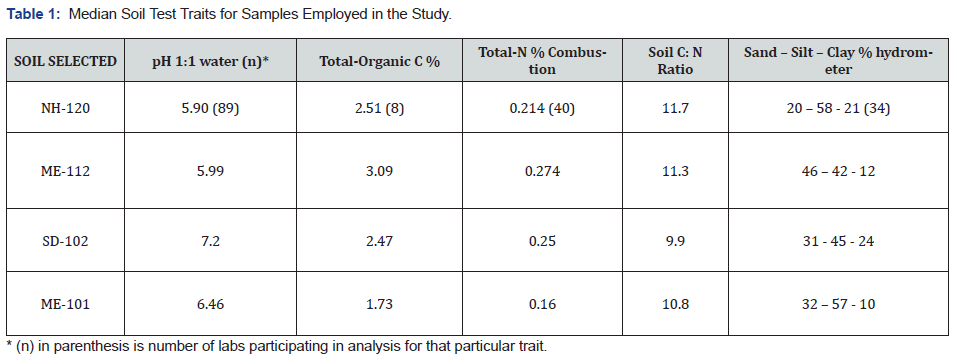
Biological tests
To obtain CO2 respiration rates 40 g of soil was moistened as indicated and evaluated after 24 hrs incubation at 20°C by utilizing two methods. Method A is the Solvita thin layer gel technique [33,35] and Method B employed an IRGA (ER-10 gas analyzer - Columbus Instruments Co, Ohio). The difference between the two approaches is that in Method A CO2 produced by microorganisms accumulates inside the sealed test jar and equilibrates with the NaOH-Borate gel impregnated with pH-sensitive dyes. The result is quantified by being read by a digital colorimeter equipped with filters for 520 nm and 470 nm from which CO2 % is derived by calibration equation. Following this, conversion to mg of CO2-C is performed internally based on the Ideal Gas Law (n =PV/RT) using R as 0.08206 atm/mol/K corrected to 20 °C and 1 ATM.
The Method B respirometer employed similarly sized test jars and was connected to a PC to record CO2 changes observed from a single beam NDIR detector during continuous operation. The instrument was set to sample continuously (from each chamber in turn) for 24 h set at 360 s cycles. By setting the ER10 respirometer to a continuous cycle each test jar in a series of 10 was automatically sampled for 6 minutes within each cycle of 1 hour with excess CO2 dumped to the air in-between. Thus, the system does not accumulate CO2 and interpolates overall respiration from sequences of the intervals by calculation for each hour. Since the IR is sensitive to water vapor, an internal water trap removes moisture and thus the soil sample will also lose moisture over time. We quantified water loss and observed a 10 - 12% loss rate within the first 24 hours which was considered acceptable. For longer term use such apparatus would need a moisturizing mechanism to avoid significant artifacts. Final respiration rate was expressed as carbon dioxide produced (ml min-1) and automatically converted by the instrument to mg of CO2 at STP and divided into soil weight to obtain mg kg-1.
For regular soil moistening at the start of incubation, to determine total pore space a fixed weight of soil is measured for bulk density and the absolute volume determined (soil weight ÷ 2.65). This amount is subtracted from the total volume to determine the available pore volume, from which 50% is water filled. Calculations of n =PV/RT for Solvita are made after first correcting for available jar air volume accounting for soil and water as factors.
In order to further evaluate effects of sieving and grinding, a biologically oriented test was included that does not depend on microbial activity, the hypothesis being that soil disturbance would not necessarily influence results unless physical-size disaggregation play a role. For measuring soil amino-N the Solvita SLAN test was employed [32] using soil samples extracted with alkali. Basically, dried and sieved (non-ground) 4-g soil samples were incubated for 24 h at room temperature in 20 mL of 2N NaOH in a 50-mL plastic beaker along with a colorimetric NH3-sensing probe inside the 265-mL sealed glass jar. The probe’s color after the incubation was determined with a digital colorimeter by comparing two filtered wavelengths (640nm and 470nm) previously calibrated to the amount of NH3–N absorbed into the thin-layer gel, according to Beer’s Law. The test results are reported as SLAN–N in milligrams per kilogram dry soil.
For measuring water stable aggregates, a volumetric aggregate stability test (VAST) was employed [35]. This is a sieving method utilizing 2 mm dried soil which is successively dipped on a 35- mesh sieve in room temperature water for 30 secs to initiate disaggregation. After destruction of the aggregates the test is repeated and the sand fraction > 0.5mm retained on the sieve is subtracted. Therefore, the resulting reported volume of soil is macro aggregates of size range 0.5 - 2.0 mm which survive the dipping process. Hypothetically preparing and sieving soil at 2 mm should not affect this size category but further processing of soil to greater fineness would be potentially highly destructive.
Results
The overall observations indicate that a determination of influence due to soil grinding, sieving and wetting must be keyed to the type of soil biological analysis performed. We observed only a slight and non-significant drop in respiration due to soil sieving when comparing fine, 0.8 mm soil to coarse, 2 mm soil. In contrast, the method of wetting soil for respiration exerted a significant impact on respiration rate, consistent with earlier observations [24,25]. For three of the four soils, the capillary wetting method caused significant reduction in observed CO2 rate. Only the Lovewell fine sandy load soil (NH) gave increased respiration with finer sieving; all the others including a river-bottom Maine Fryeburg series soil gave dramatically reduced respiration attributable to wetting method. A difficulty in interpreting data from these trials is that grinding, and sieving should normally increase respiration due to greater exposed organic matter as indicated by Waring & Bremner [26]. The effect is clearly confounded since the effect of soil destructuring from grinding also reduces the water capacity and therefore may increase the risk of over-wetting which would cause a reduction in respiration.
The overall mean CO2 respiration of the 50% WFPS method vs capillary-wetted soil was 71.8 ± 4.9 vs 39 ± 4.8 mg kg-1, respectively, approximately a two-fold difference. In contrast, the effect of sieving alone was to change the CO2 rate not significantly from 56.3 to 54.5 mg kg-1 for Coarse vs Fine, respectively. We also observed a significant interaction effect of (soil source) x (wetting). Excluding the Fryeburg soil sample that behaved differently the other 3 soils averaged 3.6-fold greater impact on respiration attributable to the wetting method. The ANOVA data is shown in Table 2. Effects for wetting were significant at p < 0.005**, for the interaction effect of (soil source) x (wetting) p < 0.001**. As expected, the respiration for actual soil source (location of sampling) were highly significantly different at p < 0.001*** (see Figure 1 and Table 2) a result correlating weakly with observed TOC in the soils.

IR Respirometry Comparison
There has been some speculation that Solvita which is thinlayer gel-chemistry based on pH differentiation due to dissolved CO2 differs from other instrumentation for CO2 measurement such as GC and infrared (IR) [36]. We ran the same set of soils by 24- hr IR respirometry and obtained nearly identical results to Solvita thin-layer gel methods in terms of effects related to sieve size and wetting methods (Figure 2). The relationship of the Solvita test and the IR response gave an r2 range of 0.93 - 0.96 for WFPS and Capillary Wetting, respectively when including all data from both wetting methods and sieving (Figure 3). In one case with the Paxton sandy loam soil IR appeared to respond more sensitively to sieving at 0.8 mm with a greater reduction in CO2 respiration than indicated by Solvita. This may be due to the moisture-interaction effect previously described for this instrumentation with differential moisture loss observed with soil samples subjected to pulse respirometry using constant air cycling. This observation however did not show up as a significant (CO2) x (Soil-Source) interaction effect in ANOVA. Thus, there is no evidence that the effect on soil respiration of sieving and wetting was expressed differently by gel-chemistry method than by IR.


Extraction of Soil Amino N
The labile-amino-N test (SLAN) employed reflects a trait in soil dependent on presence of organic-matter and utilizes NaOH for extraction, therefore it is not strictly a biological test but a chemical analysis. The overall impact of sieving was highly significant for all levels of comparison and significant at p < 0.014 for sieve size alone. For three of the 4 soils the observed magnitude of SLAN increase was small, and for the heavier clayey soil (SD) which very likely required more crushing and grinding, the impact was relatively large. The interaction effect of (soil series) x (sieving) was therefore very significant (p < 0.001). These results reinforce the concept that sieving, and grinding introduce differential factors which may have an indirect bearing on respiration and conceivably could influence longer term respiration beyond 1-day measurements. A soil study which examined grinding effects on PLFA, an extracted microbiological trait, found relatively large effects on recovered quantities of fatty acids due to the maceration effects [37].
Soil De-structuring
Based on earlier reported studies we cited, our hypothesis is that the potential negative effects of handling soils should not necessarily be confined to respiration per se but extend to include other biological traits and especially physical structuring on which respiration depends in a feedback loop of porosity and dynamic aggregate aeration. This is turn may influence analysis of other desired traits. In order to evaluate this factor, water stable aggregates (WSA) were measured on the same set of soil samples. These aggregates are defined as macro-structures of soil in the range of 50 μm - 2mm and in this study are measured by retention on a sieve between 0.5 and 2.0mm size range. WSA is associated with positive biological factors interacting with physical texture. The data show that additional sieving from < 2 mm to < 0.8 mm resulted in a significant loss of aggregate structure which was expected. The effect of soil sieving was very highly significant (p < 0.001) with adjusted r2 of 0.75. It is noteworthy that the overall reduction of WSA was on average 25% which is less than expected and suggests that a considerable amount of micro aggregates are small, densely coalesced structures surviving somewhat intensive sieving (Figure 5). The Maine Paxton sandy loam exhibited the least effect in this regard, and only required sieving and no additional grinding, whereas all the others required additional crushing and sieving and exhibited larger loss of aggregate structure. An example of the latter soil and its ability to be over-moistened with excessive soil handling is seen in Figure 6.


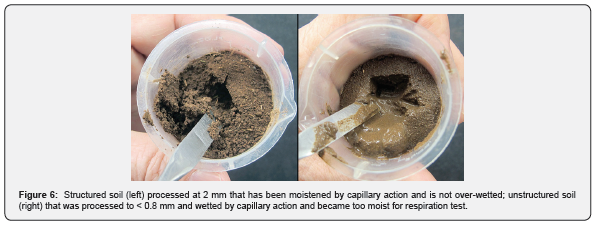
Conclusion
This study segregated four soils into two size groups, course and fine, and examined biological properties relevant to drawing conclusions about soil quality and soil health. The soil preparation methods were not severe and involved minimal processing to attain <2 mm soil, and some continued processing involving repeated crushing to obtain < 0.8 mm soil, a standard practice in soil proficiency programs. The hypothesis that additional sieving disrupts and reduces soil biological analysis was partly confirmed. Fine sieving did not decrease CO2 respiration significantly by either IRGA or Solvita, but the method of wetting after preparation and sieving caused very large effects. Sieving to a finer size also caused a significant reduction in macro-sized water stable aggregates (WSA) within the size range of the sieves indicting that the additional crushing required to attain the smaller sieve size was disrupting these small aggregates. It is, however, surprising that the reduction in aggregates was not larger suggesting aggregate strength is a significant factor. In contrast, grinding and sieving caused an increase in extractable amino-N with a significant interaction effect on soil type.
Common sense suggests that the disturbance of aggregate structure caused by lab grinding and sieving should artificially increase the availability of nonmicrobial substrates due to release of protected structures and therefore increase microbial activity [38]. However, many or most the early studies we examined did not evaluate wetting behavior as a separate variable in relation to this. Therefore, it cannot be ruled out that there are confounding influences whereby grinding and sieving potentially increase respiration while at the same time potentially compromising the quality of moisture absorption leading to reduced respiration.
The sieving effect on increased SLAN observed in this study was of relatively small magnitude except in the case of the most clayey soil we examined from South Dakota. This may be the result of the additional grinding required to obtain the < 0.8 mm fraction but may also relate to clayey soils retaining more amino-N compounds.
The study confirms our earlier work [24] that the major influence on respiration appears to be via the mechanism of wetting, which in 3 out of 4 soils tested here resulted in a significant improvement of CO2 rate but only when using the 50% WFPS wetting method instead of the capillary method. The study also employed an infrared (IRGA) respiration method. This produced similar results showing that there is nothing about the thin-layer gel technique employed by Solvita that is unique to observation of effects.
Soil laboratories should expect to obtain reliable and repeatable results with biological tests only by minimally processing soils with avoidance of crushing and grinding unless absolutely necessary. Even after preparing soil, the method of adding moisture to supply optimal microbial conditions for respiration must be carefully controlled in a manner consistent with quality, undamaged soil. Reflecting some of the cautions of early workers more recent work confirms that soil quantity and detection method technology do affect observed results [17,39]. It should also be readily possible for any lab to evaluate the impact of its grinding and sieving regimen by testing the soils under differing scenarios using multiple methods to avoid the circular argument trap [36].
It is instructive to note that while many current biologicallyoriented soil analysis methods applied to distinguish soil health effects have relatively long histories, much of the early work overlooked aspects of soil handling and preparation that are crucial to accurate reporting of soil health. Instead, by the mid 2000’s soil quality indexing which followed calls for alternate farming soil methods [7] was drawn into complex, unsatisfied issues of standardization [40].
Perhaps a way forward which captures the essence of developing testing consonant with the “holistic” topic of soil health is to view soil lab methods themselves as problematic as Rovira & Graecen [38] suggest concerning soil aggregate disruption:
“Laboratory tillage of soils causes an increase in the oxygen uptake of soil microorganisms. The effect is closely related to the extent of aggregate disruption caused by the tillage treatment and is attributed largely to exposure of organic matter that was previously inaccessible.”
Perhaps a motto for researchers in the field and laboratories offering tests in soil health would be one explicitly acknowledging that any soil test handling fundamentally compromises soil quality and soil health. However, by carefully documenting the technology and nature of soil handling and homogenization at all steps through the analysis process, labs may take continued steps forward in reporting potential management effects while understanding standards are not likely to emerge that unify the many direct and indirect influences on soil properties caused by soil testing itself.
Acknowledgment
The author thanks John Lawley, Utah State University Plants, Soils and Climate Department for preparation of these soil samples and laboratory staff of the Woods End Soil Lab for technical assistance. We also thank Herbert York of Sandy River Farms, Farmington ME, for access to his farm to obtain a sample of silty river-bottom soil.
References
- MELU (1977) Auswertung dreijähriger Erhebungen in neun biologisch-dynamisch wirtschaftenden Betrieben. Ministerium für Ernährung, Landwirtschaft und Umwelt, Baden-Württemberg, Germany.
- Jäggi W (1976) Die Bestimmung der CO2-Bildung als Mass der bodenbiologischen Aktivität. Schweiz. Landw. Forschung 15: 371-380.
- Buess O (1978) Die Gesundheit von Boden und Pflanze aus der Sicht des alternativen Landbaues. Deutscher Rat für Landespfleqe 31: 43-50.
- Anderson TH, Domsch KH (1978) A physiological method for the quantitative measurement of microbial biomass in soils Soil Biology & Biochemistry 10: 215-221.
- Beck T (1984) Mikrobiologische und biochemische Charakterisierung landwirtschaftlich genutzter Boden. I. Die Ermittlung einer Bodenmikrobiologischen Kennzahl. Zeitschrift für Pflanzenernährung und Bodenkunde 147: 456-466.
- Nestroy O, Hacker H (1986) Die Anwendung enzymatischer und mikrobiologischer Methoden In der Bodenanalyse. Communications of the Austrian Soil Science Society. 33. Vienna Austria ISSN 0029-893 X.
- Visser S, Parkinson D (1992) Soil biological criteria as indicators of soil quality: Soil microorganisms. American Journal of Alternative Agriculture 2: 33-38.
- Granatstein D, D Bezdicek (1992) The need for a soil quality index: Local and regional perspectives. American Journal of Alternative Agriculture 7: 12-17.
- Reganold JP, Palmer AS, Lockhart JC, Macgregor AN (1993) Soil Quality and Financial Performance of Biodynamic and Conventional Farms in NewZealand. Science 260(5106): 344-349.
- Lori M, Symnaczik S, Mäder P, G De Deyn, Gattinger A (2017) Organic farming enhances soil microbial abundance and activity—A meta-analysis and meta-regression. PLoS One 12(7): e0180442.
- Smith FB, Brown PE (1932) Methods for Determining Carbon Dioxide Production in Soils. Bulletin no. 147, Iowa State College of Agriculture, Ames Iowa.
- Isermeyer H (1952) Eine einfache Methode zur Bestimmung der Bodenatmung und der Carbonate im Boden. Zeitschrift für Pflanzenernährung, Bodenkunde. 56: 26-38.
- Koepf H (1954) Die biologische Aktivitat des Bodens und ihre experimentelle Kennzeichnung. Z. Pflanzenernährung Bodenkunde 64: 138-146.
- Beck T, R Roeschenthaler (1959) Eine einfache Methode zur kolorimetrischen Bestimmung der Bodenatmung im Laboratoriumsversuch. Zeitschrift für Pflanzenernährung, Düngung, Bodenkunde. 2: 143-156.
- Anderson JPE (1982) Soil Respiration. p 831-871. In A.L. Page et al (Ed) Methods of Soil Analysis. Part 2. Agron. Monogr. 9 ASA and SSSA Madison WI.
- Franzluebbers A (1999) Microbial activity in response to waterfilled pore space of variably eroded southern Piedmont soils. Applied Soil Ecology 11(1): 91-101.
- Franzluebbers AJ, KS Veum (2019) Comparison of two alkali trap methods for measuring the flush of CO2. Agronomy Journal. 2020;1–8 https://doi.org/10.1002/agj2.20141.
- Schlichting E, HP Blume (1966) Bodenkundliches Praktikum. Verlag Paul Parey Hamburg.
- Wollum ag, J E Gomez (1970) A Conductivity Method for Measuring Microbially Evolved Carbon Dioxide Ecology 51(1): 155-156.
- Koepf H (1953) Die Temperatur-Zeit–Abhängigkeit der Bodenatmung. Zeitschrift für Pflanzenernährung, Bodenkunde. 1: 29-47.
- Birch H (1959) Further Observations on Humus Decomposition and Nitrification. Plant and Soil 11(3): 262-286.
- Zibilske LM (1994) Carbon Mineralization. Pp. 835-864. In RW Weaver et al (Ed) Methods of Soil Analysis. Part 2. Series 5. ASA and SSSA Madison WI.
- Pettersson BD, Wistinghausen Ev, Brinton WF (1979) Effects of Organic and Inorganic Fertilizers on Soils and Crops; Nordisk Forskningsring: Järna, Sweden.
- https://scisoc.confex.com/scisoc/2015am/videogateway.cgi/id/23973?recordingid=23973
- Wade et al (2010) Sources of Variability that Compromise Mineralizable Carbon as a Soil Health Indicator. Soil Sci Soc Am J doi:10.2136/sssaj2017.03.0105.
- Waring SA, JM Bremner (1964) Effect of Soil Mesh Size on the estimation of Mineralizable Nitrogen in Soils. Nature 4937: 1141.
- Zöttl H (1966) Einfluß der mechanischen Zerkleinerung von Streulagenproben auf N-min-Anhäufung und C02-Produktion im Brut-Versuch. Short Communication. Plant and Soil 2: 336-340.
- Haney RL, EB Haney (2010) Simple and Rapid Laboratory Method for Rewetting Dry Soil for Incubations. Communications in Soil Science and Plant Analysis 41: 1493-1501.
- Miller R (2010) The Impact of Soil Pulverizing on Lab Analysis. Conference presentation, Starkville MS.
- Borken W, Matzner E (2009) Reappraisal of drying and wetting effects on C and N mineralization and fluxes in soils. Global Change Biology 15: 808-824.
- NAPT (2017) Soil Handling Protocol: 1 Procedures for drying NAPT soil samples. 2 Procedures for crushing and sieving NAPT soil samples 3) Procedures for Blending NAPT soil samples. SOP Document, North America Proficiency Testing and Soil Sci Soc of America.
- Moore DB, K Guillard, X Geng, TF Morris, WF Brinton (2019) Correlations between Two Alkali Extractable Amino-Nitrogen Tests and Response to Organic Fertilizer in Turfgrass Soils. Soil Sci Soc Am J 83: 791-799.
- Haney RL, Brinton WH (2008) Soil CO2 respiration: Comparison of chemical titration, CO2 IRGA analysis and the Solvita gel system. Renewable Agriculture and Food Systems 23(2): 171-176.
- Solvita (2016) Soil CO2-Burst Official Instructions Version 2016/1: Rev 700.6
- Jemison J, R Kersbergen, C Majewski, W Brinton (2019) Soil Health of Recently Converted No-till Corn Fields in Maine, Communications in Soil Science and Plant Analysis 50: 2384-2396.
- Brinton WF, Vallotton J (2019) Basis for comparisons of soil CO2 respiration test procedures. Agricultural & Environmental Letters, 4, https://doi.org/10.2134/ael2018.10.0053.
- Allison VJ, JR Miller (2005) Soil grinding increases the relative abundance of eukaryotic phospholipid fatty acids. Soil Science Society of America Journal 69(2): 423-426.
- Rovira AD, EL Greacen (1957) The effect of aggregate disruption on the activity of microorganisms in the soil. Australian Journal of Agricultural Research 8(6): 659-673.
- Franzluebbers AJ (2020) Soil mass and volume affect soil-test biological activity estimates. Soil Sci Soc Am J 1–10. https://doi.org/10.1002/saj2.20038.
- Bastida F, A Zsolnay, T Hernández, C García (2008) Past, present and future of soil quality indices: A biological perspective. Geoderma 147: 159-171.