Photopedogenesis: A Fundamental Soil Forming Process in Rock Weathering and Rhizospheric Stability on Earth and Lunar Surface
Bipin B Mishra1*, Richa Roy2
1Bihar Agricultural University, Sabour, India
2Department of Biotechnology, TNB College, Bhagalpur, Bihar, India
Submission: September 06, 2019 Published: November 07, 2019
*Corresponding author:Bipin B Mishra, Bihar Agricultural University, Sabour, India
How to cite this article: Bipin B Mishra, Richa Roy. Photopedogenesis: A Fundamental Soil Forming Process in Rock Weathering and Rhizospheric Stability on Earth and Lunar Surface. Agri Res& Tech: Open Access J. 2019; 22(4): 556231. DOI: 10.19080/ARTOAJ.2019.22.556231
Abstract
Sunlight penetrates into soil (2mm) with water based on simple classical experiment followed by subsequent interactions depending upon several soil-microbe-plant related factors in the rhizosphere. Beyond the soil, even the fresh rock under rain (wetness) promotes the growth of lichen, phototrophs, mosses, blue green algae forming mat, hyphae and root. The biomass so produced undergoes decomposition and gradually helps in weathering of the rock surface, where contribution of light in presence of moisture is vital. In almost all known soil forming processes, horizonation begins at surface of the parent material forming “O” horizon and subsequent A, B horizons over C horizon. The “O” horizon is the outcome of the contribution of sunlight and moisture. In 1996, Theory of Photopedology was initially developed, which was subsequently improved to Photopedogenesis in 2006 as being the fundamental soil forming process. Chandrayan 1 in India discovered signals for water on lunar surface (https://images.app.goo.gl/8BNb2fRoj4MXHP1C6) having weatherable rocks (basalt) and minerals (pyroxene). Photopedogenesis needs to be expanded on lunar surface for soil genesis by Indian Space Research Organization (ISRO).
Keywords:Sunlight penetration into soil; Water as a medium; Photopedogenesis; Lunar soil genesis
Introduction
Knowledge of light penetration in soil is of particular interest, wherein light penetration is distinct from light interaction (reflection, absorption, scattering, transmission, emission). Significance of photochemically active solar radiation is however substantiated in earth’s air and water environments [1]. Based on findings with diversed group of 20 soils, light induced alteration of dissolved organic carbon, nitrogen, pH, phosphorus including soluble and mineral forms of iron, manganese, aluminium, silica and calcium may be possible. Doane et al. [1] stated that the light has both pedological as well as edaphological implications. Mei Mo et al [2] published a scientific research in Journal of Frontiers in Plant Sciences under the title “How and why do root apices sense light under the soil surface, in which, based on findings, they stated that light can penetrate several centimeters below the soil surface probably on certain findings as reported by Kasperbauer and Hunt [3] and Mandoli et al. [4] However, Mei Mo et al. [2] emphasized that growth, development and behavior of plant roots are markedly affected by light despite their underground lifestyle. The spectrum and intensity of light underneath the soil surface can be sensed by roots, with diverse photoreceptors distributed specifi cally in different root zones. When sunlight strikes the ground, the spectral characters of light are altered with depth under the soil surface [3-4] Photons in the red and far-red part of the spectrum can penetrate deeper than blue light photons. Furthermore, vascular tissue can conduct light to the roots over several centimeters and, again, red to far-red light reaches deeper than blue light [5].
Mandoli et al [4] in Plant Science Department in USA investigated the light transmission through several sands, soils and size classes of “glass beads” wherein the glass beads served as a highly reflective model system for the sands. Light penetration was significantly better through sands than through either sandy or silty loams. However, the soil being so complex in its state as a natural entity could be examined for precisely for light penetration as well as interactions at soil surface-rhizosphere interface. The Theory of Photopedology [6] was accordingly the first ever attempt to witness the penetration of light into soil when wet following the classical principles, which were accumulated under a new chapter in soil science “Photopedogenesis” [7-8]. Photopedogenesis however refers not only to penetration of light but to its simultaneous interactions with soil and light loving microorganisms (phototroAbstract Sunlight penetrates into soil (2mm) with water based on simple classical experiment followed by subsequent interactions depending upon several soil-microbe-plant related factors in the rhizosphere. Beyond the soil, even the fresh rock under rain (wetness) promotes the growth of lichen, phototrophs, mosses, blue green algae forming mat, hyphae and root. The biomass so produced undergoes decomposition and gradually helps in weathering of the rock surface, where contribution of light in presence of moisture is vital. In almost all known soil forming processes, horizonation begins at surface of the parent material forming “O” horizon and subsequent A, B horizons over C horizon. The “O” horizon is the outcome of the contribution of sunlight and moisture. In 1996, Theory of Photopedology was initially developed, which was subsequently improved to Photopedogenesis in 2006 as being the fundamental soil forming process. Chandrayan 1 in India discovered signals for water on lunar surface (https://images.app.goo.gl/8BNb2fRoj4MXHP1C6) having weatherable rocks (basalt) and minerals (pyroxene). Photopedogenesis needs to be expanded on lunar surface for soil genesis by Indian Space Research Organization (ISRO). Keywords: Sunlight penetration into soil; Water as a medium; Photopedogenesis; Lunar soil genesisphs) and lichen under moist environment near the soil surface in rhizosphere.
Light has been appreciated in soil science merely as a means of heat as indicated by temperature change. However, presence of phototrophic bacteria including blue green algae, lichen, diurnal change in soil electrical conductivity, longer plant height at the centre of the densely seeded crop field, higher root proliferation during rainy season, vegetative cover change as affected by aspect (slope direction), more nitrogen efficiency in moist soils receiving intense light and possibly less infestation of insect, disease and weed during sunny period are some of appealing, but simple examples to recognize the direct role of light to the dynamics of soils even in crop production. In a qualitative expression of the “Theory of Photopedology” [6], Photopedogenesis thus seems to be the integral part of soil so long as soil is said to be a resource to plants as well as plant products [7-8].
Andrea et al. [9] determined the light penetration in soils using diffuse reflectance and transmittance spectroscopy and the relatively simple Kubelka–Munk model. Using the latter model of light propagation in turbid media, the optical properties of kaolinite, montmorillonite, barium sulphate, goethite and 19 different soils were determined in the wavelength range 275–700 nm. In particular, the light absorption coefficient, k, and light scattering coefficient, s, were determined. The depth at which the light intensity at the surface is reduced by 99% (light penetration depth) and the depth of the sample contributing to the measured reflected radiation (information depth) could also be calculated from k and s. For dry soils, an empirical relationship was established between the light absorption coefficient and the amount of particle-size fractions [9].
Survey on Impacts of Light in Pedosphere
Can sun light enter the soil surface without medium? It is also known that the optical fibers carry light. However, the upper few millimeters of soil are an area with physicochemical conditions distinct from those of bulk soil as a result of the surface being exposed to light and other environmental factors such as wind and rain erosion [10]. In soil, light directly affects those organisms on or just below the surface and indirectly by heating the soil surface. Phototrophs, such as plants, algae and cyanobacteria, use the energy from sunlight to synthesis carbohydrates. Soil surface communities are different from those of bulk soil due to the development of photosynthetic communities such as cyanobacteria, algae, mosses, and lichens, which can form biological soil crusts (BSC) with time [11-13]. The development of phototrophs at the soil surface has also been shown to have a profound impact on plant growth and biomass [14]. Adam [15] has developed light sensitive bacteria called Disco bacteria by genetically engineered to some red, green and blue light.
Lichen is probably the first life on earth, almost around 3.5 billion years ago. It is apprehended that the lichen formed on rocky substratum resembling microbial mats probably stromatolites. These microbial mats were surviving on photosynthetic organisms, producing huge volumes of organic material using energy from the sun. This organic matter was known to build up on rocky materials and gradually forming the soil. Jie Chena et al [16] presented a historical review on the interface between lichens and their rock substrates and indicated that the weathering of minerals can be accelerated by the growth of at least some lichen species. The effects of lichens on rock or mineral substrates can be attributed to both physical and chemical processes. The physical effects are reflected by the mechanical disruption of rocks caused by hyphal penetration, expansion and contraction of lichen thallus, swelling action of the organic and inorganic salts originating from lichen activity. Lichens also have significant impact in the chemical weathering of rocks by the excretion of various organic acids, particularly oxalic acid, which can effectively dissolve minerals and chelate metallic cations. As a result of the weathering induced by lichens, many rock-forming minerals exhibit extensive surface corrosion. The precipitation of poorly ordered iron oxides and amorphous alumino-silica gels, the neoformation of crystalline metal oxalates and secondary clay minerals have been frequently identified in a variety of rocks colonized by lichens in nature. For a better understanding of the impacts of lichens on environments, further work on the comprehensive involvement of the lichen effects on weathering of natural rocks, deterioration of building stonesand stonework, and formation of primitive soils should be carried out [16].
The symbiotic lichen association with algae and/or cyanobacteria, where photosynthetic symbionts provide a source of carbon and surface protection from light and irradiation, is one of the most successful means for fungi to survive in extreme subaerial environments. They need light to provide energy to make their own food. More specifically, the algae in the lichen produce carbohydrates and the fungi take those carbohydrates to grow and reproduce. Different lichens need different amounts of light [17]. That is why one can find lichens on exposed rock and desert soils, as well as on a leafy tree or in its shadow on the mossy ground below. The color of lichen is also dependent on the amount of light it receives. For example, Lobaria pulmonaria is normally in a shaded environment, yet when it grows in an exposed environment, the color is different, usually darker, and browner.
The thin strip of the earth’s surface, the soil, is the lowest boundary of the atmosphere where the incoming solar radiation in visible as well as other spectrum is subject to interactions with soil. The white soil is highly reflective compared to soils of other colours. Gray to black soils and soil rich in organic matter are relatively less reflective. Once soil is moist, it becomes less reflective and more absorbing to incoming light radiation. Calcareous white soils reflect more light compared to black and gray soils and so often are less fertile. Drip irrigation often keeps the surface soil layer drying and so escapes the light to interact with surface soil mass properly and as a result reflection of light is more possible if thin surface soil remains dry.
During rainy season, more root proliferation and vegetative growth of plants with increasing trend of microbial population are indicator of contribution of moisture and light. In absence or low intensity of light, the seed germination and growth are poor as one can observe in shade. Root proliferation is very intense when soil is moist during rains and light penetrates. In fact, light enters into the soil when it is moist, ionization in soil solution seems also to be controlled influencing the nutrient dynamics in rhyzosphere. Plant growth may thus also be an indicator to assess the interactive behaviour of soil for light. Light as an energy may be transformed to heat or other sources contributing to photosynthesis. Zinc deficiency occurs in areas with high light intensity [18].
Survey on radio transmission tower indicated that the radio frequency is diminished in area where soil is saline and moist, since such pre-condition might be conducive to ionization causing restrain to radio frequency. The electrical conductivity of soil is sensitive to temperature change through light energy. Colourless water looks white when water flows through a steep sloppy and rocky landform. When Newton’s colour chart is rotated, it also looks white. When a drop of colourless water touches the white dry cloth, the wetting point on cloth looks dull or gray until it again dries. When a soil derived from mountainous basaltic landscape looking gray to dark gray and even black in dry condition is getting moist during rain, its colour changed to reddish yellowish to even red. This might happen due to the fact that all other visible spectra (light) in moist condition are absorbed leaving red spectrum (colour) more intense (Figure 1). The shape of the land and the direction it faces make a difference in how much sunlight the soil receives and how much water soil can store. Deeper soils form at the bottom of a hill (foothill) because both gravity and water move soil particles down the slope.

Experimental Verification
During dark night, when a beam of light is flashed on a red coloured plastic tube at point B (Figure 2-i), in which water is flowing, the transparent red plastic pipe gets illuminated until its outlet, from where water is moving freely to the point A (striking point) and the flowing water is striking on a white solid surface and gives illumination with red colour at A. When a green leaf of cabbage was placed at point A, the red colour turned to white at A. This simple experiment indicates that light moves not only through water in the transparent plastic tube, but beyond the outlet of the tube with water also in the direction of flow of water (Figure 2-i).
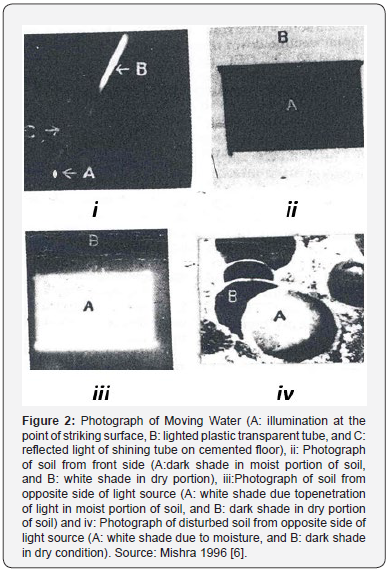
This observation was tested [6] in a thin section (2mm air dry) of soil paste (calcareous soil) on glass slide in which A portion of soil is separated from B (surrounding) with a fine knife and oven dried at 105°C. Then B portion was kept dried while A portion was moistened carefully drop by drop. The slide was then placed in front of a light source and black & white photograph was taken. What happens? On the front portion of light, the moist soil layer (A) looks black and dry soil looks more white in photograph (2- ii). Why? The light incoming from light source to the moist soil is penetrated in the soil and so look black at A. On the other hand in the same slide at B, the incoming light from its main source get reflected and looks white and more shining. This simply signifies that moist soil allows light to penetrate the soil and looks black from front side of the light source (Figure 2-ii).
Now, with the same arrangement, when we move to the same slide but from opposite side of the light source for photograph as shown in Figure 2-iii, the moist portion of soil on the same slide looks white in photograph, whereas dry portion is black. This is completely reverse to Figure 2-ii. Why it is so? It is clear that the light has passed through moist soil layer and gets illuminated at the opposite side of the light source. The photograph of disturbed soil (Figure 2-iv) from opposite side of the light source at A indicates white shade due to moisture, and B indicates the dark shade under dry condition. It discovers the following basic facts:
a. Moisture in a soil is capable to carry and penetrate the incoming light.
b. Moisture allows incoming light radiation to move with water downward.
c. Light retains colour through which it is passing and so wavelength could be changed depending upon the nature of striking material (soil types), associated constituents like organic matter, salt, iron or manganese.
d. Light loving microorganisms like blue green algae, phototrophic bacteria, lichen etc.
e. Light penetration may be linked to permeability but within a permissible spatial limit, wherein soil physical parameters like texture and structure may play major role.
f. Rainy season could be a very favourable period to verify how the impact of light contribute to root and plant growth in a particular ecosystem
Light as a Driving Force in Soil Genesis: Photopedogenesis
By “no soil” is meant that there is no sustenance of humans. Soil is a complete prescription for livelihood security. In fact, human exists because of soil. We take birth on soil, live on soil, walk on soil, die on soil and finally vanish in soil. Soil is synonym to Soul with immense spiritual values. Our civilization flourishes because of soil. Our health, culture and social behaviour are dictated by soils. And, soil exists primarily because of sun light over known common factors. The incoming solar radiation on earth surface (soil) includes visible, infrared, microwave and radio wave. Only a small fraction of visible (500-1000 candle) radiation is being consumed for photosynthesis by green plants and remaining part of visible, infrared, microwave and radio wave are subject to interaction. Arguments are forwarded that the incoming solar radiation beyond 700nm predominantly contributes to thermal manipulation in the environment. Even with regard to the visible radiation, almost around 60% of it is consumed during photosynthesis and the remaining 40% is virtually a strange for other purposes.
This research’s results also highlighted some knowledge management antecedents for the promotion of the agricultural development. They also represent relevant aspects for the sector’s success, which are: the incentive to innovation and management practices and technological transference; the need to integrate the public policies related to agriculture; the promotion of formal agricultural education; the viabilization of self-management on subnational levels and the interconnection of agricultural information systems of reference. Such actions can be understood as the need to develop national systems of innovation for the development of the agricultural sector. Thus, it is believed that the promotion and the adoption of several applications of science data, as well as the generation of national environments of innovation, are the most relevant aspects of knowledge management for the nations’ agricultural development. In conclusion, future researches will be able to investigate which are the main applications/practices of knowledge management for agricultural development, and how these applications have collaborated with the development of the sector in several parts of the world.
Pedology broadly refers to Genesis, Evolution, Landscape continuity, Classification, Survey and Evaluation of Soils for Land Use Planning by restoring productivity, safety, resilience, profitability and overall sustainability in order to enable the present generation to transfer its soil to the next generation without any abuse or degradation or environmental imbalance. But pedology begins basically with weathering of rocks and minerals. Edaphology refers to soil-plant relation mostly in relation to nutrition and confined normally from soil surface to rhizosphere Pedology is neither dead nor buried [19], but it functions in changing litho-pedo environments as induced by natural factors as well as anthropogenic management inputs. The soil beneath our feet is important from several aspects; such as where should I build my home, from what will my house be made: brick or wood or adobe? From where does water come? Underground aquifers or rivers, and how it is cleaned?
Once light enters and interacts with soil mass, it makes soil capable of doing work by changing its colour or otherwise. A white dry soil looks almost dull or gray when moist, but black soil looks reddish when moist, if it occurs on hilltop containing minerals like haematite. The low intensity of sun light in dense forest often delays the organic matter mineralization and promote podzolization, laterization and decalcification depending upon nature of parent material, vegetation type and climate (mainly rainfall). Intense light declines organic matter content in soil and promote shrinkage, salinization, alkalization etc. In calcareous waterlogged soils, greenish algal growth is profound as affected by light intensity.Soil with poor organic matter is more sensitive to the diurnal change in soil temperature. All radiations are not important for all purposes in plant sciences and the soil acts as filtering materials to allow only those radiation suitable to plants. Depending upon intensity, light can either destroy or promote selective microbial population in soils.
Soils are resilient to environmental changes. By this is meant that soil will recover from or adjust to change if sufficient ‘pedological’ time is allowed. The soil management practices that have been applied by humans in a short time frame are unsustainable -a declining soil health threatens human livelihood. The resilience of the soil in terms of human expectations and time frames will depend on its ability to recover to an equilibrium state once improved practices have been extensively applied.
Pedogenesis is a continuous process of soil formation virtually from parent material with an ultimate product called regolith or non-soil mass or simply soil residues in a set of factors comprising of parent material, biotic fauna and flora, relief, organism and climate as conditioned by relief, management and degradation with reference to time scale as energized by light photon. Light in whole system is an integral part to induce both initiation and maturity of soil development through its impact on diurnal temperature change, growth of fauna and flora on parent material and energy supply during biochemical, photochemical and electrochemical reactions. In particular, lichens are key players in a variety of environmental processes as well as rock weathering (Figures 3 & 4). For example, cyanobacterial photobionts participate in nitrogen fixation. Lichens also contribute to a phenomenon known as biological weathering. The lichen mycobionts can break down rocks and release minerals by producing certain chemicals. Lichens can also disrupt rock surfaces simply by physically attaching to them, and by the expansion and contraction of their thalli [16].

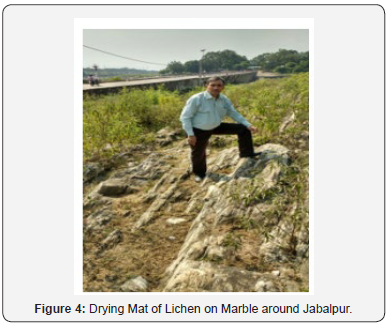
Dokuchaev established the soil forming factors and put forward an equation as

International recognition came to Jenny after the 1941 publication of Factors of Soil Formation: A System of Quantitative Pedology. He developed the generic mathematical relationship that connects the observed properties of soil with the independent factors in the process of soil formation as

where s – any or combined soil features (pedogenic); cl - regional climate; o - potential biota, r - relief; p - parent material; t - time.Jenny’s famous state equation for soil formation is as

which may be further modified and elaborated following the assumptions as climate (cl) is variable and all other four factors are constant (identical), the possible changes in soil features would be the function of varying climate and the soils developed under varied climate will be called climofunctions. By this is meant that pedogenic soil features will vary according to variation in climate. This led to the development of empirical models to describe pedogenesis, such as clinofunctions, biofunctions, topofunctions, lithofunctions, and chronofunctions.
However, two additional soil forming factors namely light radiation and sustainable management (Figure 5) are proposed earlier [20]. The first one is associated with initiation of weathering of rocks, while second contributes when soil begins to form. If the rock or weathered materials are lying in dark, devoid of light radiation, phototrophic microorganisms may not grow even with sufficient moisture and air and photosynthesis in green plant species remains restricted. Such situation slows down the formation of soil. However, even the low intensity of light in wet condition can facilitate the growth of phototrophic biota including lichen and moss on the fresh surface of rock. The thin mat of lichen is observed to facilitate the growth of higher plants and their thin roots spread profusely and penetrate in cavities or crevices in rocks, wherein these growing roots after being strong cause disintegration of rocks even. The whole system is initiated by light not just because light is a source of energy, but because its role to photosynthesis as well as phototrophic biota leading to soil formation.
Thus, the overall soil forming factors may be written as

wherein lr is light radiation and mgt is management. This state function could be expressed additionally as Photofunctions and Anthrofunction, respectively.
With passing of zero time, maximum alteration occurs on the surface of parent material through the process of soil genesis (true soil) in which light has specific role to play preferably under moist condition either through its transformation to heat or other energy sources, wherein light gets entry with water and interacts with parent material in presence of biotic fauna and flora, organic matter and moisture thus forming weathered material or even true soil in three dimension. Can we overlook the key role of light in pedogenesis? In fact, it is light induced surface layer of parent material that leads to horizon differentiation in the three-dimensional array of soil body called profile with O-A-E-B-C horizons following the sequence as below:

Here, it is to note that initiation of the true soil formation from parent material (C) is the organic horizon (O), which has direct linkage with light and moisture. Besides, horizon O remains always at the top of a profile and subsequent horizons are direct
relation with the top one (O horizon. However, variation in profile development is controlled by several factors including the geomorphology of the landscape (Figure 6). Thus, photopedogenesis has direct relevance among pedogenic processes covering a wide range from rock weathering to rhizospheric stability in the landscape.
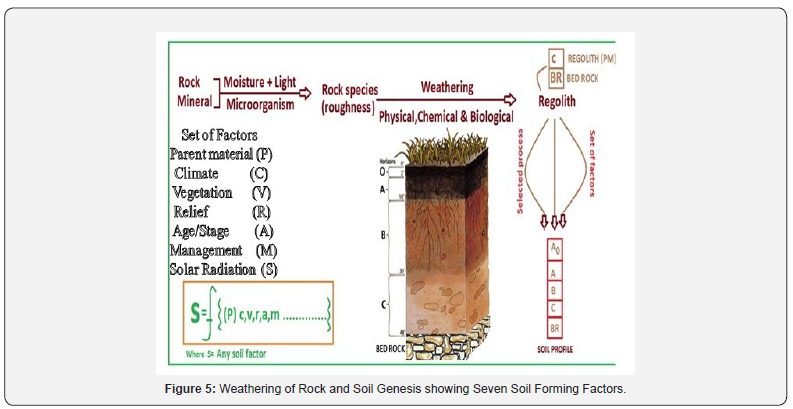
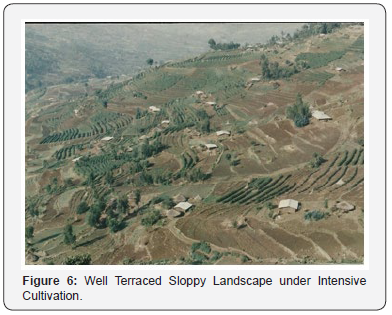
Photopedogenesis and Lunar Rock
It is presumed with current available knowledge that there is no soil on moon because there is no air, water and vegetation or even microorganism except sun light, necessary to bring change to the rock or minerals, on the moon for soil formation (pedogenesis). The mineral content on the lunar surface was mapped with the Moon Mineralogy Mapper, a NASA instrument and established the presence of iron as the subsequent changes in rock and mineral composition. This indicates the abundance of iron-bearing minerals like pyroxene. The Chandrayan’s Moon Mineralogy Mapper has confirmed the magma ocean hypothesis, meaning that the Moon was once completely molten. On 24 September 2009, Science journal reported that the Moon Mineralogy Mapper (M3) on Chandrayaan-1 had detected water ice on the Moon. But, on 25 September 2009, ISRO announced that the Chandrayaan-1 had discovered water on the Moon three months before NASA’s Mission as confirmed by NASA [21], Times of India 2008, Science Journal, 2009, The Hindu, 2013, DNA Bangalore, 2013, Bagla Pallav 2013).
This discovery of water with Indian Chandrayaan 1 mission (Figure 7) will certainly help to work for future of soil formation on moon, The fuffy (soft) appearance of lunar surface (Figure 7) could be suspected to have basaltic rocks being capable of absorbing moisture and intended to chemical reaction especially the reduction, if microorganisms and air will appear to support photopedogenesis [7-8] in presence of light and moisture on rock species at lunar surface. However, the existence of water in any form or state on lunar surface may probably be an indication of “primitive atmosphere” on moon, which may have unstable as well as differing gaseous composition. As reviewed by Mishra et al [22], the composition of primitive atmosphere of the earth was entirely different from present day’s composition [23]. Now, the Chandrayan 2 of ISRO which has started functioning in lunar orbit on 7th September 2019 would strengthen the current knowledge with more authentic database utilizable even in modern pedology, wherein photopedogenesis may substantiate and contribute to discover the pedogenic road map on moon and other planets in days to come. Besides, the fundamental principles to expand our knowledge to climate change issues may be possible to refine.

Conclusion
Under such situation, culture of suitable but specific microorganism seems to be a possibility. As such, ISRO and NASA should come forward to undertake further investigation on possible trend of soil genesis on Moon or other planets like Mars simply under basic principles lying with Photopedogenesis, since evidence of water in any form or state also indicates the possible existence of primitive atmosphere which may support biotic environment with selective microorganisms. This necessitates researchable planning with ISRO in order to promote future strategies towards soil genesis on Moon and other planets.
References
- Pieters R, Vander-Graff N (1980) Resistance to Gibberella xylarioides in Coffee Arabica: Resistance to Gibberella xylarioides in Coffea arabica: Evaluation of screening methods and evidence for the horizontal nature of the resistance. Netherlands Journal of Plant Pathology 86(1): 37-43.
- Lewis Ivey M, Miller S, Hakiza G, Geiser D (2003) Characterization of the coffee wilt.
- Stewart RB (1957) Some plant diseases occurring in Kaffa province, Ethiopia. Imperial Ethiopian College of Agriculture and Mechanical Arts Alemaya Ethiopia.
- Kranz J, Mogk M (1973) Gibberella xylarioides Heim et Saccas on Arabica coffee in Ethiopia. Journal of Phytopathology 78(4): 365-366.
- Van der Graaff NA, Pieters R (1978) Resistance levels in Coffea arabica to Gibberella xylarioides and distribution pattern of the disease. Netherlands Journal of Plant Pathology 84(4): 117-120.
- Girma A (1997) Status and economic importance of Fusarium wilt disease of Arabica coffee in Ethiopia.
- Oduor (2005) Surveys to establish the spread of coffee wilt disease, Fusarium (Gibberella) xylarioides, in Africa. In: Proceedings of the 20th International Scientific Conference on Coffee Science (ASIC), pp. 1252-1255.
- Meyer FG (1965) Notes on wild Coffea arabica from southwestern Ethiopia, with some historical considerations. Economic Botany 19(2): 136-151.
- Paulos D, Demel T (2000) The need for forest germplasm conservation in Ethiopia and its significance in the control of coffee diseases. In: Proceedings of the Workshop on Control of Coffee Berry Disease (CBD) in Ethiopia, pp. 125-135.
- Merdassa E (1986) A review of coffee diseases and their control in Ethiopia. In: Abate, T. (Edt.), Proceedings of the First Ethiopian Crop Protection Symposium. Institute of Agricultural Research Addis Ababa Ethiopia, pp. 187-195.
- (2003) CABI (CAB International) Surveys to assess the extent and impact of coffee wilt disease in East and Central Africa. Final technical report. CABI Regional Centre Nairobi Kenya, pp. 149.
- Pieters R, Vander-Graff N (1980) Resistance to Gibberella xylarioides in Coffee Arabica.
- Hakiza GJ, Birkunzira B, Musoli P Proceedings of the First Regional Workshop on Coffee Wilt Disease (tracheomycosis). International Conference Centre Kampala Uganda, pp. 53-61.
- Evaluation of screening methods and evidence for horizontal Nature of the resistance. Netherlands journal of Plant pathol 86: 37-43.
- Pathogen in Uganda. Phytopathol 93: 550.