Reducing the Carbon Emission with Sustainable Green Corridor for Win-Win-Win Strategies
BB Jana*
International Centre for Ecological Engineering, University of Kalyani, India
Submission: December 20, 2018; Published: October 22, 2019
*Corresponding author: BB Jana, International Centre for Ecological Engineering, Centre for Environmental Protection and Human Resource Development, University of Kalyani, Kalyani- 741235, West Bengal, India
How to cite this article: BB Jana. Reducing the Carbon Emission with Sustainable Green Corridor for Win-Win-Win Strategies. Adv Biotechnol Microbiol. 2019; 14(5): 555897. DOI: 10.19080/AIBM.2019.14.555897
Keywords
Environment; Climate change; Municipal; Greenhouse gas; Industrial; Chlorococum; Ankistrodesmus; Separate branches; Chlorella sp; Scenedesmus sp; Sphaerocystis sp; Spirulina sp
Introduction
Global warming and climate change is the ultimatum before mankind in twenty first century posing a serious threat to the survival of human race. This was the driving force for a vast number of worldwide researches to stabilize CO2 emissions in the atmosphere and to counteract impinging interconnected issues of global warming, biodiversity loss, ecosystem service and so on. Forecast is that it is hardly possible to stop the global warming; even if all emissions were to stop today, the Earth’s average surface temperature would rise to another 0.6 degrees or so over the next several decades. This delayed reaction is due to the presence of excess energy that is stored in the ocean, which has a tremendous heat capacity. The man-made interrelated global warming and climate change has not only manifested its negative impacts on environment but is also responsible for weather related natural disasters including tropical cyclones, tsunamis, drought, high intensity storms fueled by water vapor contained in the atmosphere. Climate change could cause more severe droughts in 98% of European cities. A new theory has been put forward to suggest that there is a link between man-made climate change and increased seismic events including earthquakes, tremors, volcanic activities, etc (McGuire ? ), There is a general consensus that an increase in temperature variability will have more stress on hot weather rather than cold weather. India is already experiencing a late shift of on soon causing serious economic loss to farmers (NAAS, 2019).
As per guidelines for 17 sustainable Development Goals (SDGs) of the United Nations (2015), 2030 as the target year for MDGs, carbon sequestration should address zero hunger, climate action, life on land. Accordingly, India has to reduce the GHG emis sion by 33-35% by 2030 considering 2005 as base line level. Furthermore, creation of additional carbon sink of 2.5 to 3 million tons of carbon dioxide equivalent in necessary by 2030. There are several setbacks that hinder to achieve the target. While trying to solve one issue, a new problem arises out of it. For instance, food security is a challenge to human race, but more than 50 % food is simply wasted due to faulty processing, food wastes causing global warming through feedback mechanism. Burning of rice crop residue is associated with carbon emission. Similar losses do occur along the food chain in different sectors such as harvesting, processing of fish, meat, cereals, etc (NAAS, 2019). As global warming cannot be stopped, it is rightly urged to have adaptive strategies that are evolved to challenge the issue of global warming by reducing the vulnerability to the effect of climate change. Mitigation is a action that can reduce the pace of greenhouse gas emissions. Adaptations and mitigations are complimentary to each other necessitating the integration of adaptation into mitigation strategies.
Mitigation through wastes recycling Any material of the Earth is bound to turn into waste as per the second law of thermodynamics. This very waste may become source of carbon emission or a resource following the waste management hierarchy to achieve zero waste. Wastes are of two categories- solid and liquid. Solid wastes are generated from municipal, household, industrial, commercial sites, while liquid wastes include sewage, industrial effluents, etc. Though both of them play important role in global cycling of carbon and global warming, they have different modes of action. Solid wastes act as a source GHG emission through burning or anaerobic decomposition, while the later is largely involved in carbon capture and sequestration sink.
Emission of GHG from solid wastes can be reduced by following the principle 4-R (Figure 1) with the promotion of turning wastes into wealth through recycling and to achieve the mission for zero waste concept (Figure 2).
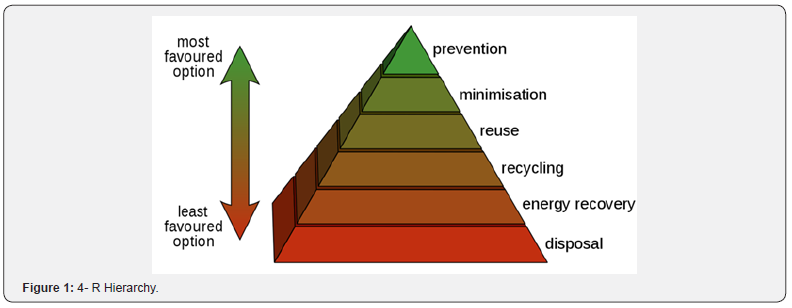
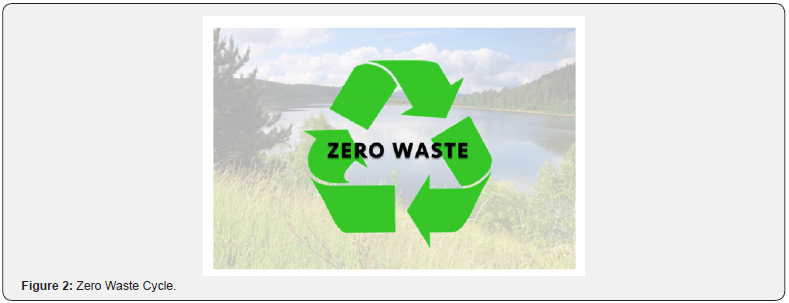
Indian scenario
Currently in India, about 960 million tons of solid waste is being generated per year from different sectors of which 350 million tons are organic (agricultural sources), 290 million tons are inorganic (industrial and mining sectors) and 4.5 million tons are hazardous wastes from different sectors. The contribution of GHG of these wastes is primarily through emissions of carbon dioxide (CO2), methane (CH4) and nitrous oxide (N2O).The major quantity of wastes generated from agricultural sources are sugarcane baggase, paddy and wheat straw and husk, wastes of vegetables, food products, tea, oil production, jute fiber, groundnut shell, wooden mill waste, coconut husk, cotton stalk etc. The municipal and urban wastes include animals’ wastes, horticulture wastes, domestic refuses and other agro industrial wastes. These wastes are expected to reach 300 MT by 2047 creating a big problem for management.
By composition, the organic wastes mainly consist of organic matter (46%), paper (6%), glass (0.7%), rags (3.2%), plastic (1%) and the rest is moisture. Though some efforts are being made for reuse of these materials in value added applications such as bricks, blocks, tiles, aggregates, ceramics, cement, lime, soil, timber and paint [1], a large fraction of it causes environmental pollution during different stages of decomposition process. High concentrations of carbon monoxide, oxides of Sulphur, oxides of nitrogen and suspended particulate matter are invariably emitted to the atmosphere led to pollution of air, water, soil, that finally affect flora, fauna, aquatic life and human health. Several simulation models are now available to predict the potentials of solid waste to contribute GHG based on the life cycle assessment. Based on this criteria, the IPCC estimated 2.7% of the global GHG gas emission from different wastes such as landfill/dumping, composting, waste incineration and sewage disposal. However, this data does not fully reflect the actual potential for reducing GHG emissions by the waste management sector while comparing end of pipe and life cycle approaches [2]. A case study of the city of Cairo showed that a consequent recycling and composting approach can even lead to a negative emissions balance, meaning that the SWM sector is a net sink [2].
Indian scenario
Mitigation through wastes recycling Any material of the Earth is bound to turn into waste as per the second law of thermodynamics. This very waste may become source of carbon emission or a resource following the waste management hierarchy to achieve zero waste. Wastes are of two categories- solid and liquid. Solid wastes are generated from municipal, household, industrial, commercial sites, while liquid wastes include sewage, industrial effluents, etc. Though both of them play important role in global cycling of carbon and global warming, they have different modes of action. Solid wastes act as a source GHG emission through burning or anaerobic decomposition, while the later is largely involved in carbon capture and sequestration sink.
Biofuel production form agricultural wastes
There is a growing concern for agricultural wastes, especially the rice straw is mostly burnt contributing GHG emission to the atmosphere. hemi cellulosic and cellulosic biofuel production systems enable the conversion of nearly all sugar-based plant polymers into fuels (ethanol, butanol, diesel) [3]. Ongoing research at the Indian Institute of Technology, Kharagpur, West Bengal, India on rice straw show that about 4-5% ethanol (v/v) has been obtained from agricultural rice straw. (Banerjee, personal communication). Methane generated from anaerobic decomposition can be trapped for production of cooking gas – a source of energy, while the residue is converted into safe manure. This is novel method of turning solid wastes into zero wastes in the form of manure using aerobic, anaerobic or combined processes of decomposition. Vermi- composting has been emerging fast as an eco-friendly protocol to utilize suitable organic substrates as high quality manure.
Mass production of algae
Biological sequestration is of considerable significance in trapping atmospheric carbon dioxide in aquatic environment. Algae are also considered for food, fuel, cosmetics, soil fertilizer, filtering materials, pest control, sewage treatment components. Further, their recognition for carbon sequestration is well known. As a result, considerable efforts have been made to culture different types of algae for biomass production to be used for bioelectricity, bioethanol, biodiesel, biochar, gasoline, etc. using different technologies.
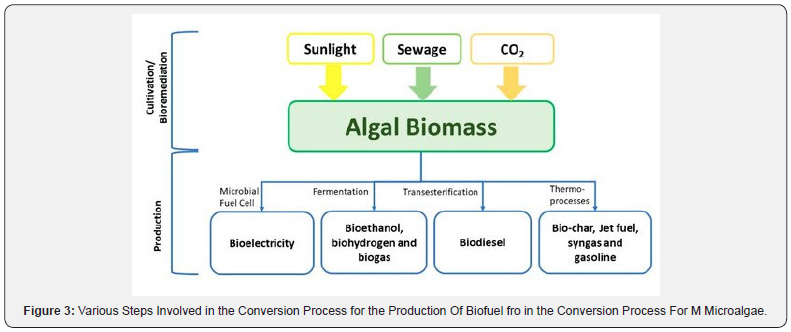
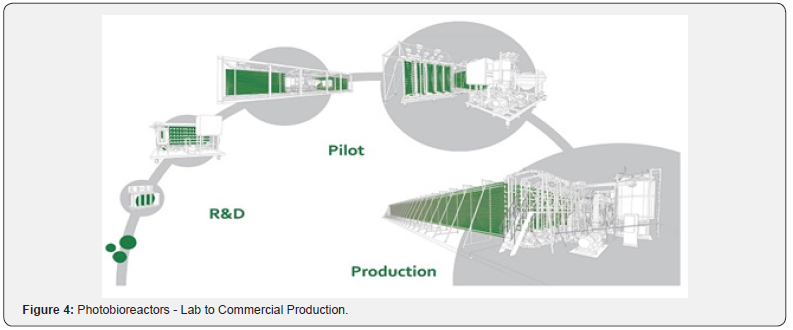
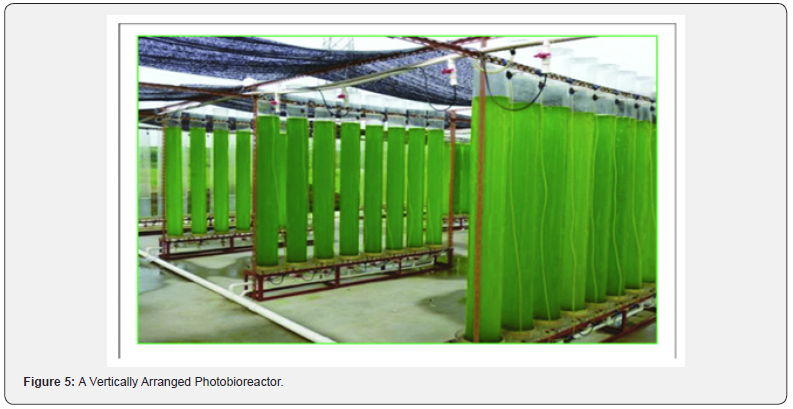
Photobioreactors
A photobioreactor is a bioreactor that utilizes a light source to cultivate phototrophic microorganisms under open or closed condition. A variety of photobioreactors (Figure 3-5) are used to culture a large number of photosynthetic organisms such as microalgae, cyanobacteria, purple bacteria utilizing natural sunlight in open water and artificial illumination in closed condition. The advantage of closed photobioreactor is that algae is cultivated in a large scale because of possibility of maintaining specific condition required for algal growth in closed photobioreactor [4]. Hypothetically, phototropic biomass could be derived from nutrient-rich wastewater and flue gas carbon dioxide in a photobioreactor. The design of such apparatus plays an important role in the success of production.
Biofuel production from algae
One of the important uses of microalgae is the production of fuel where high lipid content of microalgal biomass can be extracted and recertified into fuel. A large number of researches are dedicated for exploring the best strains of algae and developing the most efficient farming practices under different environmental conditions for the production of fuel [5].Various estimates indicate that potential oil and biomass yields from algae ponds range from 20,000 to 60,500 liters per hectare per year (2000 to 6000 gallons per acre per year) and 50,000 to 15,000 kilograms per hectare per year (140 to 420 tons per acre per year), respectively [6].
Carbon capture in open water
One advantage of aquatic carbon capture and biomass production in microalgae is the ability to capture CO2 in ponds in a nongaseous form as bicarbonate to fertilize algal growth. It is known that 1.6 and 2 grams of captured CO2 is used for every gram algal biomass [7]. Further efficiencies are gained by harvesting 100% of the biomass, much more than is possible in terrestrial biomass production systems. At moderate pHs (≥ pH 7) and temperatures (below 30 degrees Celsius), the dominant form of CO2 in water is bicarbonate. The microalgae are able to capture as high as 90% of carbon dioxide or bicarbonate in open ponds though the ability varies among species. About 20% CO2 tolerant strains such as Scenedesmus, Chlorococum and Ankistrodesmus formed several separate branches suggesting that certain groups or genotypes of algae tend to perform better under high CO2 level compared with other algal groups [8]. A microalgal consortium consisting of Chlorella sp., Scenedesmus sp., Sphaerocystis sp. and Spirulina sp. procured from wastewater ponds revealed quite high CO2 sequestration (53- 100%; 150- 291 mg/g) [9].
Mechanism of carbon capture
Photoynthesis by microalgae and aquatic plants occurs in two phases. The first reaction is light dependent where protein rich chlorophyll pigments are held in chloroplast. The second reaction or dark reaction or Blackman’s reaction is slower than light reaction and takes place in the stroma of chloroplast that embedded in the plasma membrane of photoautotrophs. The black reaction also occurs in the presence of light. There are two cyclic reactions in dark reaction –Calvin cycle or C-3 cycle and Hatch and Slack pathway or C4 cycle. The Calvin cycle or C3 cycle, first observed by Calvin in unicellular green alga Chlorella, is a cyclic reaction occurring in the dark phase of photosynthesis when energy poor CO2 is converted into energy rich sugars during the process of carbon fixation or assimilation (Figure 6 & 7).
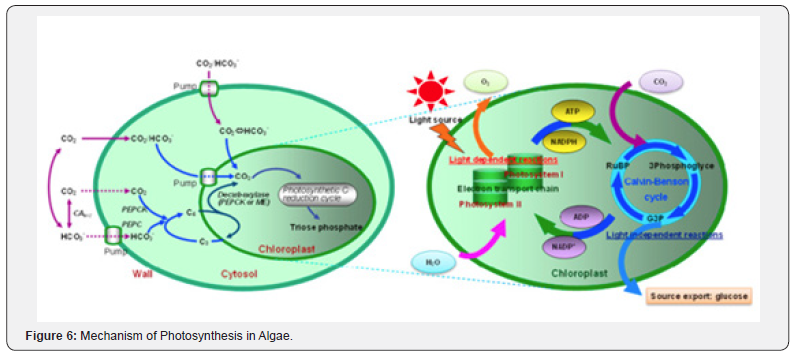
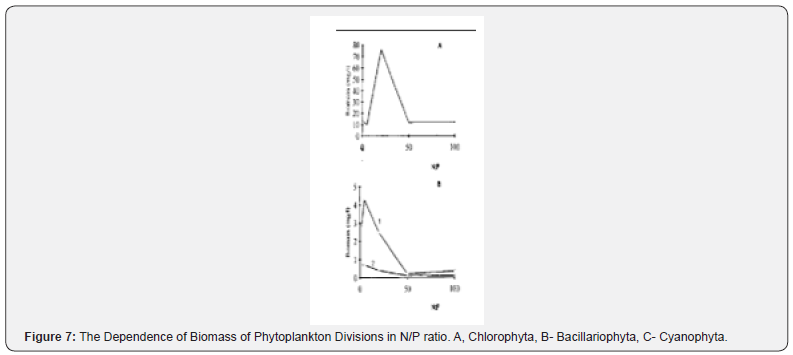
Rubisco (Ribulose-1,5-bisphosphate carboxylase/oxygenase) is an enzyme and is involved in the first major step of carbon fixation when carbon dioxide is converted to glucose (Figure 4). In this pathway, it involves the carboxylation of ribulose bis-phosphate (RuBP) in presence of RuBisCO producing phosphoglycerate (PGA). The Rubisco enzyme is capable of utilizing both CO2 and O2 leading to formation of carboxylation in presence of CO2 and oxygenation of RuBP in presence of oxygen, respectively (Figure 4). When concentration of O2 is high and CO2 is low, RuBisCO acts as an oxygenase and further catalyzes the photorespiration reaction resulting in reduced carbon fixation due to production of 2-carbon molecule phosphogycolate. Many aquatic plants using RUBISCO as their initial carboxylase counter the limitations on CO2 supply via the operation of biophysical CO2 concentrating mechanisms which are based on active transport of HCO−3, CO2 or H+ at the plasmalemma, and use bulk‐phase HCO−3 or CO2 as the C source [10]. In aquatic environment, algae capture carbon is pumped into the cell by bicarbonate transporters present in both the plasma membrane and in the chloroplast envelope of eukaryotic algae [11]. Inside the chloroplast, bicarbonate is concentrated, dehydrated spontaneously or by carbonic anhydrase through Calvin-cycle activity, finally yielding algal biomass.
Manipulation of N/P ratio of input fertilizer
Manipulation of N/P ratio of input fertilizer in open ponds is another method for mass culture of microalgae. In a Canadian lake lowering the N/P atomic ratio to about 22 favors the nitrogen fixing cyanobacteria. N/P ratios are lowered when P is added and species that once P limited become limited by N. Green algae some diatoms and blue greens incapable of reducing N2 may find high N/P ratio favorable. The addition of P changes the picture The N/P ratio of the aquatic system play a critical role in the regulating the dominance of blue-green or green microorganisms. Decrease of the N: P ratio, through the addition of phosphorus compounds, leads to cyanobacterial blooming, while addition of nitrogen results in the development of blooming of green algae Chlorococcales which does not have negative effects since they are actively consumed by grazers [12]. Thus, blue greens are most abundant at lower N/P ratio than at green algae. As the N/P ratio gets higher, the biomass of green algae increased while that of cyanobacteria decreased (Figure 7). It has been experimentally demonstrated that the growth of blue green algae Microcystis aeruginosa was maximum with longest exponential growth when initial nitrogen concentration was at 10 mg/l and the N/P ratio of 16 [The results of the growth of microalgae at different N/P ratios (5, 20, 40, 50, 60, 70, 80, 90, 100) of water using urea and single super phosphate as sources of N and P showed almost similar pattern of growth in all the treatments, but the magnitude of peak was highest in the treatment of 70, followed by 80 or 60. The samples in control remained the lowest all throughout [14]. Fortuitously, the benefits of flue gas injection on algal growth can be greater than the growth impacts solely attributed to inhibition of photorespiration by high CO2 concentrations. Recent studies have shown that direct injection of flue gasses into ponds increases biomass productivity by 30% compared with direct injection of an equivalent concentration of pure CO2. However, the efficiency of CO2 capture by algae can vary according to the state of the algal physiology, pond chemistry, and temperature. Carbon-dioxide capture efficiencies as high as 80% to 99% are achievable under optimal conditions and with gas residence times as short as two seconds [5].
Role of wetlands in carbon sequestration
Wetlands contain about 35 per cent of global terrestrial carbon though they comprise only 6 - 9% of the Earth’s surface. The net sinks for carbon in different wetlands have been estimated to be at rates up to 3 g C m-2 d-1 [15,16]. Sediments of aquaculture ponds are again very important in the carbon sequestration. The carbon sequestration by aquaculture ponds is about 0.21% of the annual global emissions. However, under unmanaged anaerobic conditions, wetlands can also emit greenhouse gases such as methane, nitrous oxide and hydrogen sulphide though this is limited in saline conditions or swampy areas. Notwithstanding the manifold positive impacts of wetlands overweighed towards beneficial environmental impacts and led to argue that wetland should not be destroyed or restored because of GHG emissions under limited conditions [17].
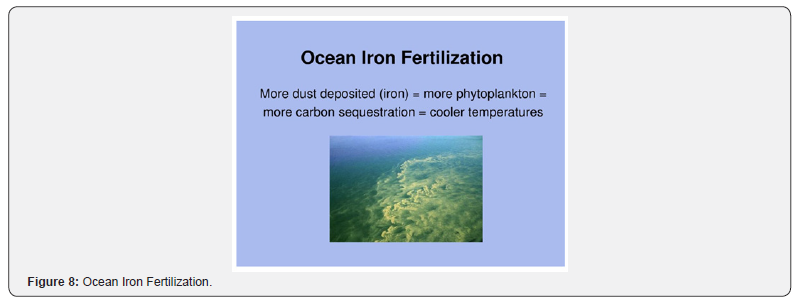
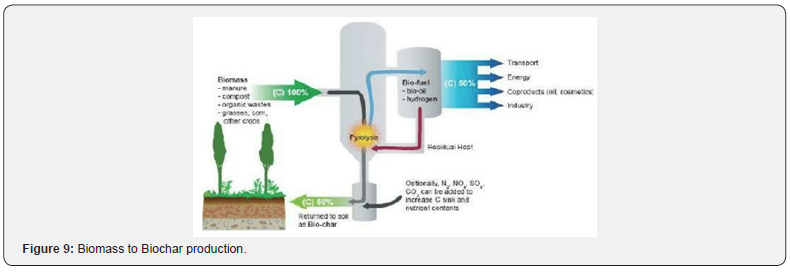
Carbon sequestration through burial
There are two possible ways of carbon sequestration
a. Permanent burial of total algal biomass in deep geologic formations, and
b. Burial of extracted or processed carbon-rich fractions from algal biomass [18]. One of the interesting way of biomass carbon sequestration is dumping iron filling or urea into seawater to stimulate phytoplankton production in areas that have photosynthetic production (Figure 8 & 9). This is because phytoplankton production is limited by iron deficiency. Direct burial of algal biomass is the most energy-efficient way to sequester carbon, because no dewatering is required after processing. However, considerable amounts of inorganic nutrients such as N and P would also be buried with the algal biomass. About 7% of algal dry weight is inorganic elements or ash. It is suggested to bury only the neutral lipid or hydrocarbon fraction of the algal biomass.
Biotechnological interventions
Biotechnology of algae has received much impetus since new species of microalgae that are being invented are capable of tolerate extremely high CO2 levels (40 - 100 vol%) and raised temperatures. Some of the mechanisms that regulate the cellular pH homeostasis of microalgae are: the photosynthetic apparatus state transitions that increase ATP generation, upregulation of H (+) -ATPases pumping protons out of the cell, rapid shutdown of CO2-concentrating mechanisms, and adjustment of membranes’ fatty acid composition [19].
Biochar
Biochar is charcoal used as a soil amendment. Biochar is a stable solid, rich in carbon, and can endure in soil for thousands of years. Biochar is more than 90% carbon and is a by-product of pyrolysis at high temperatures. Biochar is used for carbon sink, soil amendments. Slash – char, water retention, and energy production. It is rightly recommended that instead of being buried, it may be used for various reasons such as reducing energy costs and expanding the range possible applications [20-28].
Conclusion
Because of the excellent ability of microalgae to pump nongaseous bicarbonate into cell biomass in aquatic environment, microalgal culture is one of the lucrative tools for mitigation of global warming through trapping of atmospheric CO2 into the biomass of microalgae that served as natural food for zooplankton and herbivorous fishes. Selective microalgae can be grown by manipulation the pond fertilization schedule. This would help to grow selective microalgae which would capture atmospheric carbon and serve as food source for low carbon footprint fishes. Therefore, these optimum conditions can be applied for high growth of Chlorella. in open ponds.
References
- Pappu A, Saxena M, Asolekar SR (2011) Wastes to wealth – Cross sector waste recycling opportunities and challenges. Canadian Journal Environmental Construction and Civil Engineering 2: 14.
- Spies S (2010) SWM GHG Calculator -a Tool for Calculating Greenhouse Gases in Solid Waste Management (SWM) Manual KfW Entwicklungsbank Regine VOGT, IFEU Jürgen GIEGRICH, IFEU Ellen GUNSILIUS, GTZ.
- Pauly M, Keegstra K (2008) Cell-Wall Carbohydrates and Their Modification as a Resource for Biofuels. Plant J 54(4): 559-568.
- Zhu Y, Anderson DB, Jones SB (2018) Algae Farm Cost Model: Considerations for Photobioreactors. Pacific North West National Laboratory, PNNL-28201.
- Keffer JE, Kleinheinz GT (2002) Use of Chlorella vulgaris for CO2 mitigation in a photobioreactor. J Ind Microbiol Biotechnol 29(5): 275-280.
- Weyer KM, Bush DR, Darzins Al, Willson DB (2010) Theoretical Maximum Algal Oil Production. Bio Energy Research 3(2): 204- 213.
- Herzog H, Golomb D (2004) Carbon capture and storage from fossil fuel use. Encyclopedia of energy 1(6562): 277-287.
- Zheng Liu, Fan Zhang, Feng Chen (2013) High throughput screening of CO2-tolerating microalgae using GasPak bags. Aquat Biosyst 9(1): 23.
- Bhakta JN, Lahiri S, Pittman JK, Jana BB (2015) Carbon dioxide sequestration in wastewater by a consortium of elevated carbon dioxide tolerant microalgae. Journal of CO2
- Raven JA (2010) Inorganic carbon acquisition by eukaryotic algae: four current questions. Photosynth Res 106(1-2):123-134.
- Spalding KL, Arner E, Westermark PO, Bernard S, Buchholz BA (2008) Dynamics of fat cell tur nover in humans. Nature 453(7196): 783.
- Levich AP (1996) The role of nitrogen-phosphorus ratio in selecting for dominance of phytoplankton by cyanobacteria or green algae and its application to reservoir management. Journal of Aquatic Ecosystem Health 5(1): 55- 61.
- Liu X, Lu X, Chen Y (2011) The effects of temperature and nutrient ratios on Microcystis blooms in Lake Taihu, China: An 11-year investigation. Harmful Algae 10(3): 337-343.
- Jana BB (2019) CO2 mitigation potentials of microalgae: A new proposition for closing the loop of carbon through food chain of herbivorous fishes in open ponds. 34-40.
- Bonneville MC, Strachan IB, Humphreys ER, Roulet NT (2008) Net ecosystem CO2 exchange in a temperate cattail marsh in relation to biophysical properties. Agricultural and Forest Meteorology 148(1): 69-81.
- Zhou L, Zhou G, Jia Q (2009) Annual cycle of CO2 exchange over a reed (Phragmites Australis) wetland in Northeast China. Aquatic Bot 91: 91- 98.
- Mitsch WJ, Bernal B, Nahlik AM, Mander U, Zhang L, et al. (2019) Saving the harvest: Reducing the food loss and waste. Policy Brief. No 5 National Academy of Agricultural Sciences, New Delhi, India, pp. 10.
- Sayre, R (2010) Microalgae: The Potential for Carbon Capture Bio Science 60(9): 722–727.
- Solovchenko A, Khozin-Goldberg I (2013) High-CO2 tolerance in microalgae: possible mechanisms and implications for biotechnology and bioremediation. Biotechnology. Lett 35(11): 1745-52.
- Badger MR, Price GD (1994) The role of carbonic anhydrase in photosynthesis. Annual review of plant biology 45(1): 369-392.
- Hangata N, Takeuchi T, Fukuju Y (1992) Tolerance of microalgae to high CO2 and high temperature. Phytochemistry 31(10): 3345- 3348.
- Heilmann SM, Davis HT, Jader LR, Lefebvre PA, Sadowsky MJ, et al. (2010) Hydrothermal carbonization of microalgae. Biomass and Bioenergy 34(6): 875-882.
- IPCC (2014) Climate change Impacts, Adaptations, and Vulnerability. Fifth Assessment Report of the Intergovernmental Panel on Climate Change.
- Jana BB (1998) The State-of-the-art of lakes in India: An overview. Archives fur Hydrobiology (Suppl) (Monograph Studies) 121: 1-89.
- Marcel J, Johannes T, Luuc RM, René HW (2003) Enclosed outdoor photobioreactors: light regime, photosynthetic efficiency, scale-up, and future prospects. Biotechnol Bioeng 81(2): 193-210.
- Nogia P, Kaur S, Mehrotra R, Mehrotra S (2016) Capturing atmospheric carbon: biological and nonbiological methods. International Journal of Low-Carbon Technologies 11(2): 266-274.
- Raven JAQ, Osborne BA, AM Johnston (1985) Uptake of CO2 by aquatic vegetation. Plant Cell Environ 8: 417- 425.
- Zhu XG, Long SP, Ort DR (2008) What is the maximum efficiency with which photosynthesis can convert solar energy into biomass? Curr Opin Biotechnol 19(2): 153-159.