Emulsification and Foaming Properties of Locust Bean (Parkiabiglobosa) and Pigeon Pea (Cajanuscajan) Seed Flours and their Protein Isolates
Oyewole AA*, Abu TFA and Enujiugha VN
Department of Food Science and Technology, Federal University of Technology, Nigeria
Submission: January, 2017; Published: February 07, 2017
*Corresponding author: AOyewole AA, Department of Food Science and Technology, Federal University of Technology, Ihiagwa PMB 1526, Nigeria, Email: akindayor79@gmail.com
How to cite this article: Oyewole A, Abu T, Enujiugha V. Emulsification and Foaming Properties of Locust Bean (Parkiabiglobosa) and Pigeon Pea (Cajanuscajan) Seed Flours and their Protein Isolates. Nutri Food Sci Int J. 2017; 2(3): 555590. DOI: 10.19080/NFSIJ.2017.02.555590
Abstract
Emulsification and foaming properties of Locust bean (Parkia biglobosa) and pigeon pea (Cajanus cajan) seed flours and their protein isolates was investigated in this study. Also, their protein isolates were profiled for amino acid status. Some of the flour and protein isolate samples were heat-treated at 85 °C for various times. The functional properties results showed that pigeon pea was rich in most of the properties except water and oil absorption capacity. Pigeon pea flour has higher bulk density (0.82g/cm3), loose density (0.63g/cm3), emulsion stability (73.38%), foaming stability (79.81%) and least gelation (14.37%) than locust bean flour. Locust bean flour has higher swelling capacity (2.17g/ cm3), water absorption capacity (3.76ml/g), oil absorption capacity (2.69ml/g), emulsification (33.68%) and foaming capacity (20.68%) than pigeon pea flour. Pigeon pea protein isolate treated at 85 °C for 10 minutes was a better emulsifier and foaming agent than Locust bean protein isolate with the same heat treatment. Protein solubility of pigeon pea was higher at all temperatures (60-90°C) than Locust bean. Pigeon pea protein isolate treated at 85 °C for 10 minutes gave higher values of both essential and non-essential amino acids showing that pigeon pea would be more useful in food formulations involving both low and high pH.
Keywords: Functional properties; Flour; Protein isolates
Introduction
World demand for proteins is increasing and so more food protein is required from both conventional and new sources of protein. Accepting that all proteins will have nutritional value, and then in both cases successes in the food industry, requires that protein have good functional properties to be acceptable as a food ingredient Akubor et al. [1]. African Locust bean seed is often cooked and later fermented. The fermented African locust bean seed is called dawadawa in Hausa, iru in Yoruba and ogiri in Ibo land of Nigeria; it is called kpalugu among the Kumasis and Dagombas of Northern Ghana, kinda in Sierra Leone and netelou or soumbara in Gambia. It is the most important natural food condiment in the entire Savannah region of West and Central Africa [2]. Pigeon pea (Cajanus cajan), a locally available, affordable and under-utilized grain legume of the tropics and sub-tropics. Pigeon pea varieties have protein content in the range of 23-26% [3]. The protein content is comparable with those in other legumes like cowpea and groundnut which have been used in complementing maize.
It is rich in mineral quality and fiber content. Pigeon pea grows well in Nigeria but the hard-to-cook phenomenon and the presence of anti-nutrients have limited its utilization [4]. Emulsification and proteins and other amphoteric molecules possess in the development of traditional or novel foods. During emulsification, these ingredients facilitate stable oil droplet formation through development of interfacial membranes. The membranes prevent coalescence of droplets, and so enhance droplet dispersion in the immiscible phase of the emulsion [5]. Foam formation and stability are also dependent on interfacial membrane formation or barrier establishment to prevent coalescence of air bubbles [6].
Therefore, apart from the use of products that consist mostly of proteins or starch, it may be possible to form and stabilize emulsions and foams with suitable combinations of both products. Though various pea flour products are currently produced in commercial quantities; utilization in food formulation is limited due to lack of information on their functional properties. In order to enhance acceptability of pea seed flours and Locust bean flour, there is need to provide some basic information on their ability to act as emulsifiers and foaming agents. Though the functional properties of Locust bean and Pigeon pea seed flours and their protein isolates have been established, there is need to determine the effects of heat on their emulsifying and foaming properties; as well as the Amino acid profile of their protein isolates
Materials and Methods
Procurement of raw material
The locust beans (Parkia biglobosa) and pigeon pea (Cajanus cajan) were obtained from a local market in Ado- Ekiti, Ekiti State, Nigeria. Care was taken to purchase all the seeds from a particular source so as to significantly reduce environmental and varietal errors. The seeds were processed at Food processing laboratory of the Food Science and Technology, Federal University of technology, Akure, using standard method
Equipments used
Soxhlet extractor, Test tubes, measuring cylinder, conical flask, Centrifuge, domestic blender, Freeze dryer, Technicon Sequential Multi-sample Amino acid Analyzer (TSM), Spectrometric Mineral Analyzer. All chemicals used were of analytical grade.
Preparation of seed flour samples
The seeds were screened to eliminate stones and other foreign materials. The locust bean seeds were cooked for 8hours and the tough hard seed coats were manually removed. The cotyledons were washed and cleaned thoroughly, drained and dried for 8hours at 90 5 °C in an oven. The dried sample was comminuted with a laboratory type hammer mill into fine particle size (≤ 300μm). The flour was then preserved in dry airtight jars at -4 °C prior to use. The pigeon pea, after screening, was washed thoroughly to remove dusty particles, drained and dried for 8hours at 90±5 °C in an oven. The dried sample was comminuted with a laboratory type hammer mill into fine particle size (≤ 300μm). The flour was then preserved in dry airtight jars at -4 °C prior to use. The flours were then defatted with n-hexane for 16hours. The hexane was decanted and the flour was air-dried and used for protein isolation preparation.
Preparation of protein isolates
The defatted flour was dispersed in distilled water at flour to water ratio of 1:10 (w/v); the pH was increased to 10 with 0.1M NaOH and stirred for 3hours at room temperature. Extract was separated by centrifugation at 10,000g for 30minutes. The residues were re-extracted twice under the same conditions. The extracts were then combined and protein was precipitated by adjusting the pH to 4.5 with 1M HCl before centrifugation. The protein isolate was washed twice with distilled water. It was then suspended in distilled water and the pH was adjusted to 7.0 with 0.1M NaOH prior freeze-drying (Figure 1).

Determination of bulk density
This was determined as outlined by Osundahunsi and Aworh. Ten grams of protein isolates were put into 100ml graduated cylinder and was tapped several times on the laboratory bench till the isolate stopped settling and values were expressed as g/cm3.
Protein solubility
The protein isolates (250mg) were homogenized in 20ml of 0.1 M NaCl at pH 7 for 1hour followed by centrifugation at 10,000 x g for 340 minutes. Nitrogen contents were determined in the soluble fractions and solubility was expressed as the percentage of total nitrogen of the original sample to that of soluble fraction [7].
Water absorption capacity
The sample (3g) was mixed with distilled water (25ml) and placed in pre-weighed centrifuge tubes. The tubes were stirred and centrifuged for 25minutes at 3000 x g after 30 minutes interval. The supernatant was removed by 25 minutes drainage at 50 °C, and protein isolate sample was re-weighed. Water absorption capacity was expressed as the number of grams of water absorbed per gram of sample [8].
Oil absorption capacity
The sample (0.5g) was mixed with corn oil (6ml) in preweighed centrifuge tubes. The tubes were stirred for one minute to get the complete dispersion of the sample in the oil. After 30 minutes holding time, the sample was centrifuged at 3000 x g for 25minutes. The separated oil was then removed with a pipette and the tubes were inverted for 25minutes to drain the oil prior to reweighing. The oil absorption capacity was expressed as grams of oil absorbed per gram of the sample [8].
Emulsifying activity and stability
Protein isolate (3.5g) was homogenised for 30 seconds in 50ml water using homogenizer at approximately 10,000rpm. Corn oil (25ml) was added and the mixture was homogenized again for 30 seconds. The emulsion was divided into two equal volume aliquots and centrifuged at 1100 x g for 5minutes; one aliquot was heated for 15 minutes at 85 °C according to the procedure of [9]. The ratio of the height of emulsion layer to the height of liquid layer was noted to calculate emulsion activity. The emulsion stability was expressed as the percentage of emulsifying activity remaining after heating [9].
Foaming capacity and stability
The capacity and stability of foams were determined by the method of Lin et al. [10]. A 50 ml of 3% (w/v) dispersions of protein isolate sample in distilled water were prepared and immediately transferred into graduated cylinder; volume was recorded before and after whipping. Foaming capacity was expressed as the percentage volume induced by whipping. The change in volume of foam after 60 minutes of standing at room temperature was recorded as foam stability.
Determination of protein
The Kjeldahl method was used for the determination of percentage crude protein. The process was in three stages:
Digestion: (0.5g) of the sample was weighed into Kjeldahl digestion flask and selenium catalyst was added. 10 ml concentrated H2SO4 was added. At this stage, all the carbon present in the sample would be converted to carbon (IV) oxide (CO2), nitrogen to ammonium sulphate (NH4)2SO4, sulphur and phosphorus to their oxides, which were given off as gases. The mixture was heated on an electro-thermal heater until solutions were obtained. Chemical reaction involved during digestion process:
Distillation: This involves steam distillation of the diluted digested samples which have been left to 5ml with distilled water after they have been made up to 50 ml with distilled water after they have been left to cool with 10 ml of 40 % NaOH. 5ml of 2% boric acid solution was pipette into 100ml conical flask to which 4 drops of mixed indicators was added (0.01g methyl red + 0.083g bromocresol green, 100ml alcohol). The conical flask was placed in such a way that the delivery tube touched the boric acid level inside the conical flask while the tube was been cooled with cold water. The 40 % NaOH solution was used to titrate ammonia out of the whole digest indicating that the solution has become alkaline. During distillation, all other outlets were tightly closed to avoid loss of ammonia. The liberated ammonia was trapped in boric acid in receiver flask turn light green, indicating the presence of ammonia. Distillation was continued until about 50 ml of distillate was collected into receiver flask.
Chemical reactions involved at this stage are as follows:
Titration: Titration was the final stage. The resulting solution from the distillation was titrated with 0.1M HCl until the initial colour was observed at the end point.
The chemical reaction involved is as follows:
The % Nitrogen was calculated from titre value as follows:
Where D = Dilution factor, T = Titre value, W = Weight of sample
Determination of amino acid profile
The amino acid profile in the known sample was determined using methods described by Benitez. The known sample was dried to constant weight, defatted, hydrolysed, evaporated in a rotary evaporator and loaded into the Technicon Sequential Multi-Sample Amino Acid Analyser (TSM).
Defatting of the sample
The sample was defatted using chloroform/ methanol mixture of ratio 2:1. About 4g of the sample was put in extraction thimble and extracted for 15hours in Soxhlet extraction apparatus [11].
Determination of nitrogen
A small amount (200mg) of ground sample was weighed, wrapped in whatman filter paper (No.1) and put in the Kjeldahl digestion flask. Concentrated sulphuric acid [10ml] was added. Catalyst mixture (0.5g) containing sodium sulphate (NaSO4), copper sulphate (CuSO4) and selenium oxide (SeO2) in the ratio of 10:5:1 was added into the flask to facilitate digestion. Four pieces of anti-bumping granules were also added. The flask was then put in Kjeldahl apparatus for 3 hours until the liquid turned light green. The digested sample was cooled and diluted with distilled water to 100ml in standard volumetric flask. Aliquot [10ml] of the diluted solution with 10 ml of 45% sodium hydroxide was put into Markham distillation apparatus and distilled into 10ml of 2% boric acid containing 4 drops of bromocresol green/ methyl red indicator until about 70ml of distillate was collected.
The distillate was then titrated with standardised 0.01N hydrochloric acid to turn into grey colour
a = Titre value of the digested sample
b = Titre value of blank sample
V = Volume after dilution (100ml)
W = Weight of dried sample (mg)
C = Aliquot of the sample used (10ml)
14 = Nitrogen constant (mg)
Hydrolysis of the sample
A known weight of the defatted sample was weighed into glass ampoule. 7ml of 6NHCl was added and oxygen was expelled by passing nitrogen into the ampoule (this was to avoid possible oxidation of some amino acids during hydrolysis e.g methionine and cystine). The glass ampoule was then sealed with Bunsen burner flame and put in an oven pre-set at 105 °C ±5 °C for 22 hours. The ampoule was allowed to cool before broken open at the tip and the content was filtered to remove the humus. It should be noted that tryptophan was destroyed by 6NHCl during hydrolysis. The filtrate was then evaporated to dryness at 40 °C under vacuum in a rotary evaporator. The residue was dissolved with 5ml of acetate buffer (pH 2.0) and stored in plastic specimen bottles, which were kept in the freezer.
Results and Discussion
Functional properties of locust bean and pigeon pea seed flours
The results of functional properties of the locust bean and pigeon pea flours are shown in Table 1. The locust bean flour has higher swelling capacity (2.17g/cm3), water absorption capacity (3.76ml/g), oil absorption capacity (2.69ml/g), emulsification [33.68%] and foaming capacity [20.68%) than pigeon pea flour that has swelling capacity [1.29g/cm3), water absorption capacity (2.60ml/g), oil absorption capacity [1.66ml/g], emulsification [32.20%] and foaming capacity [31.19%]. While pigeon pea flour has higher bulk density (0.82g/cm3), loose density (0.63g/cm3), emulsion stability (73.38%), foaming stability (79.81%) and least gelation [14.37%] than locust bean flour that has bulk density [0.75g/ cm3), loose density (0.44g/cm3), emulsion stability (51.07%), foaming stability (67.75%) and least gelation (12.22%).

Bulk density
The results of bulk density of locust bean and pigeon pea seed flours are presented in Table 1. There existed no significant difference in bulk density of the two samples. The bulk density was higher in pigeon pea (0.82 ± 0.02g/cm3) than locust bean flour [0.75 ± 0.02 g/cm3). The defatting process results porous texture of the defatted product that can be attributed for low bulk density would be an advantage in the formulation of complementary foods [12]. Present results are supported by [1]; the cowpea flour has bulk density 0.64g/cm3, while its isolates have bulk density of 0.82g/cm3 as investigated by [13]. Among the legume flours, peas and pigeon pea flours showed bulk density of 0.55 and 0.81g/cm3 respectively, whereas [14] reported similar findings for chickpea and winged bean flours.
Water and oil absorption capacity
Protein has both hydrophilic and hydrophobic properties thereby can interact with water and oil in foods. The results of water and oil absorption capacity of locust bean and pigeon pea seed flours are shown in Table 1. Locust bean flour showed higher water and oil absorption capacity (3.76 and 2.69ml/g respectively] than pigeon pea flour [2.60 and 1.66ml/g] respectively. Variation in water and oil absorption capacity of protein may be due to different protein concentration, their degree of interaction with water and oil and possibly their conformational characteristics.
The lower water absorption capacity of protein isolates is due to less availability of polar amino acids [15] and low fat absorption may be due to the presence of large proportion of hydrophilic groups and polar amino acids on the surface of the protein molecules [16]. Ragab et al. [13] found that water and oil holding capacity for cowpea protein isolates are 220% and 110%, respectively. In case of pea protein isolates, [17] observed 170% water absorption capacity and 120% oil absorption capacity. According to El-Adawy [18], mung bean had water and oil absorption capacity 200% and 135% respectively and for pigeon pea 87% and 173% [19]. Also these findings are in line with those reported by Kaur et al [14].
Least gelation concentration of locust bean and pigeon pea seed flours
A qualitative parameter expresses the minimum protein concentration at which the gel does not slide along the test tube walls in inverted position [20]. The lower the least gelation concentration the better is the gelling ability of proteins [21] because protein gels are aggregates of denatured molecules. Results showed that pigeon pea contained higher least gelation concentration (14.37%) while locust bean has 12.22% as shown in Table 1. The gelation formed at 14.37% for pigeon pea compared favourably with those reported for pigeon pea flour (14%) by [22].
Similarly, 16% least gelation concentration of cowpea protein isolates was observed by [4]. According to [19]; pigeon pea formed gel at 12%. It was reported that firm and resistant gels are formed from soy protein isolates at 16-17% concentrations and gelation properties are interrelated to water absorption capacities hence the low water absorption capacity recorded by the protein isolates flours could explain the deficient gel formation capacity. Gelation takes place more readily at higher protein concentration because of greater intermolecular contact during heating. High protein solubility is always necessary for gelation as observed by Wiltoon PR et al. [23].
Protein solubility of locust bean and pigeon pea flours at different temperatures

The results of the temperature effects on protein solubility of Locust bean and Pigeon pea seed flours are shown in Figure 2. It shows that pigeon pea has higher percentage solubility within the temperature range of 60-90°C than locust bean. However, the percentage solubility of both samples dropped as the temperature was increased except locust bean that was more soluble at the temperatures of 80 and 90 °C respectively. The isoelectric point value was 7.0. The isoelectric point of both samples are close to the values quoted by Oshodi et al (1993) [4] (4.0 and 7.0) and Ige MM et al. [25] (6.0 and 8.0). A-Locust bean flour, B- Pigeon pea flour Protein isolate soluble at pH 4-7 could be used in such beverages as milk [26]. The high temperature solubility of Locust bean and Pigeon pea protein indicates that they may be useful in the formulation of acid beverages and very low acid foods like meat products or beverages with high pH [27].
Swelling power profile of locust bean and pigeon pea flours at different temperatures
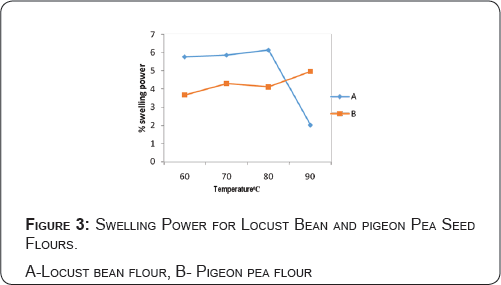
The temperature-dependent swelling power profile is presented in Figure 3. The results indicate that locust bean flour has more swelling power than pigeon pea flour at the temperature range of 60-80 °C. Whereas pigeon pea flour has higher swelling power than locust bean flour at the temperature of 90 °C.
Emulsion capacity and stability of locust bean and pigeon pea seed flours and the protein isolates

The results of the different samples are presented in Figure 4. The results show that samples ppI0, ppI5 and ppI10 have higher emulsion capacity than samples LbF10, LbF0 and ppF0; while samples LbI10, ppF5, and ppF10 have the least emulsion capacity while the results of emulsion stability of different samples after they were allowed to stand for 1hour at room temperature are shown in Figure 5. The results show that samples PpF0, PpI10, LbF0 and LbF10 have high emulsion stability; while samples PpF10, LbI5 and LbI10 were least stable. Protein, being the surface active agents, can form and stabilize the emulsion by creating electrostatic repulsion on oil droplet surface [28].

Maximum emulsifying activity was observed in pigeon pea protein isolates without heating (45.28%) followed by pigeon pea protein isolate heated for 5minutes at 85 °C (43.64%), locust bean flour heated for 5 minutes at 85 °C (42.11%) and pigeon pea protein isolate heated for 10 minutes at 85oC (42.11%), locust bean flour heated for 10minutes at 85 oC (40%), locust bean flour without heating (34.06%). While locust bean protein isolate heated for 10minutes at 85 °C, pigeon pea flour heated for 5 minutes and 10 minutes at 85 °C have the least emulsion capacity of 9.52, 9.08 and 8.87% respectively. Emulsion stability of the locust bean and pigeon pea seed flours and their protein isolates are shown in Figure 5. The results show that pigeon pea flour without heating has the highest value of 76%, followed by the pigeon pea protein isolates heated at 85 °C for 10minutes (67.49%) and the locust bean flour without heating (66.82%).
While locust bean protein isolate heated at 85 °C for 5minutes and locust bean protein isolate heated at 85 °C for 10 minutes have the least values (40.00% and 30.04%) respectively. The results shows the study are in concordance with those reported earlier by Mwasaru MA et al. [22]; who calculated 48.16 and 54.90% emulsifying activity and stability for cowpea, 39.50 and 44.98% for pigeon pea protein isolates. According to [19] pigeon pea contained 97.97%emulsion stability. [13] reported the emulsifying activity of 50% and stability of 82% for cowpea protein isolates. Emulsion stability of 55.5% was observed by Fuhrmeister H et al. [29] for smooth pea protein isolates.
Foaming Capacity and Stability of Locust Bean and Pigeon Pea Seed Flours and the Protein Isolates
The results of foaming capacity of different samples are presented in Figure 6. The results show that samples PpF5, PpF10 and PpI10 have high foaming capacity; while samples LbI5, LbI0 and LbF10 have the least foaming capacity. Foam formation is an important requirement in the manufacture of foods such as ice cream, cakes and meringues. Therefore, the ability of the pea seed flours to form foams could be essential to their application in the manufacture of non-dairy foods. The foaming properties are used as indices of the whipping characteristics of protein isolates [30]. The results of foaming capacity of locust bean and pigeon pea seed flours and their protein isolates are presented in Figure 5.
Maximum foaming capacity of 33.33% was observed in pigeon pea flour heated at 85 °C for 5 minutes, followed by pigeon pea flour heated at 85 °C for 10 minutes (32.14%) and 27.58% for pigeon pea protein isolate heated at 85 °C for 10 minutes. However, locust bean protein isolate heated at 85 °C for 5 minutes, locust bean protein isolate without heating and locust bean flour heated at 85 °C for 10 minutes have the least values of 8.33, 6.96 and 5.26% respectively. Low foaming capacity could be due to inadequate electrostatic repulsions, lesser solubility and hence excessive protein-protein interactions [31]. Whereas, higher value for foaming stability indicates highly hydrated foams and decrease in foaming stability might be due to protein denaturation.

The results of foaming stability of locust bean seed flours and their protein isolates are shown in Figure 6. The results followed a similar trend as foaming capacity. Maximum foaming stability of 100%was observed in pigeon pea protein isolate heated at 85 °C for 10 minutes and locust bean flour heated at 85 °C for 10 minutes, followed by pigeon pea flour heated at 85 °C for 5minutes (93.80%) and 88.90% for pigeon pea flour heated at 85 °C for 10 minutes. However, locust bean protein isolate heated at 85 °C for 5minutes, locust bean flour without heating and locust bean protein isolate heated at 85 °C for 10minutes have the least values of 52.22%, 48.22% and 29.40% respectively. Kaur & Singh [14] observed decrease in foam volume with the passage of time for protein isolates of different chickpea cultivars.
. A similar trend was reported for mucuna bean protein concentrates by Adebowale KO et al. [32]. The decrease in foam volume as a function of time was observed for all protein isolates. These results agree with the findings of Mwasaru et al [30]; who reported foaming capacity and stability for cowpea 35.30%, 79.40% and 34.00%, 77.80% for pigeon pea protein isolates, respectively. [13] reported foaming capacity of 65% for cowpea protein isolates. Pigeon pea had foaming capacity and stability 80% and 102% as reported by Akintayo ET et al. [21] and 44.70%, 78.79% by [19], respectively. Likewise, values for these traits in mungbean were 108% and 58% [18]. In a study conducted by Fernandez-Quintela A et al. [17], it was observed that pea protein isolate has 94% foaming stability
Foaming stability of locust bean and pigeon pea seed flours and the protein isolates
The results of foaming stability of different samples after they were allowed to stand for 1hour at room temperature are presented in Figure 6. The results show that samples PpF10, LbF10 and PpI10 have higher foaming stability than all other samples. Samples LbF5, LbF0 and LbI10 were least stable. Figures 5& 6 showed the results of effects of heat on foaming capacity and stability of locust bean and pigeon pea seed flours and their protein isolates. It was observed that the pigeon pea flour heated at 85 °C for 5 and 10 minutes respectively have the highest foaming capacity of 33.33% and 32.14% respectively. Whereas, locust bean protein isolate without heating and locust bean flour heated at 85 °C for 10 minutes have the least values of 6.96% and 5.26% respectively (Figure 5). The foam formed by the locust bean flour and pigeon pea protein isolate heated at 85oC for 10 minutes have the highest stability values of 100% each.
This was followed by pigeon pea flour heated at 85 °C for 10 minutes. While locust bean flour without heating and locust bean protein isolate heated at 85 °C for 10 minutes have the least values of 48.22% and 29.40% respectively (Figure 6).The effects of heat treatment on the emulsion formation and stability are shown in Figure 3& 4 respectively. It was observed that the pigeon pea protein isolate heated at 85 °C for 5 minutes (43.64%) and the pigeon pea protein isolate heated at 85 °C for 10 minutes (42.11) have better emulsion capacity. While the locust bean flours heated at 85 oC for 5 and 10 minutes respectively had their emulsion capacity enhanced (42.11% and 40% respectively) as shown in Figure 3.The emulsion formed by pigeon pea flour without heating was most stable (76%), followed by pigeon pea protein isolate heated at 85 °C for 10 minutes (67.49%). Whereas locust bean protein isolate heated at 85 °C for 10 minutes was least stable (30.04%) as shown in Figure 4. This is due to the initial heating of locust bean to enhance ease of de-hulling which would have led to protein denaturation
Amino acid profile of locust bean and pigeon pea protein isolates
The results of the amino acid profile of locust bean and pigeon pea protein isolates of different samples are presented in Table 2. The results show that samples PpI10 and PpI5 have higher amino acid values especially some essential amino acids, followed by samples LbI5 and LbI10; while samples LbI0 and PpI0 have the least values of amino acid. It was observed that heat treatment at 85 °C enhanced the availability of virtually all the amino acids. Pigeon pea protein isolate heated at 85 °C for 10minutes has the highest values of all the amino acids analyzed and this was followed by pigeon pea protein isolate heated at 85 °C for 5 minutes. Whereas the locust bean protein isolate heated at 85 °C for 5 minutes has higher values of amino acids than locust bean protein isolate heated at 85 °C for 10 minutes. Meanwhile, both isolate samples that were not heated have the least values of amino acids. The amino acid composition of both locust bean and pigeon pea protein isolates is comparable to that of FAO/WHO reference protein (FAO/WHO) [33] for growing children from 3 to 5 years of age, except for tyrosine and lysine. The levels of essential amino acids such as leucine, isoleucine and valine in pigeon pea found in the present study are higher than locust bean protein isolates.

These differences in amino acid composition can be attributed to differences in the preparation methods. The results from the study have shown that heat treatment enhanced foaming capacity and stability; emulsion capacity and stability (to some extent) of both locust bean and pigeon pea seed flours and their protein isolates. The results also showed that heat-treated pigeon pea protein isolate has higher value of Amino acid than locust bean. Hence, it is suitable to prepare protein enriched products. The traditional foods from both locust bean and pigeon pea are expected to serve as income generating sources for the women in boosting their family sustainability [34-36].
Sample legends
- LbF0 - locust bean flour without heat, LbI0 - locust bean protein isolate without heat
- PpF0 - pigeon pea flour without heat, PpI0 - pigeon pea protein isolate without heat
- LbF5 - locust bean flour heated for 5mins, LbF10 - locust bean flour heated for 10mins
- PpF5 - pigeon pea flour heated for 5mins, PpF10 - pigeon pea flour heated for 10mins
- LbI5 - locust bean protein isolate heated for 5mins, LbI10 - locust bean protein isolate heated for 10mins, PpI5 - pigeon pea protein isolate heated for 5mins
- PpI10 - pigeon pea protein isolate heated for
References
- Akubor PI, Flora O, Adamolekun CA, Oba HO, Abudu IO (2003) Chemical composition and functional properties of cowpea and plantain flour blends for cookie production. Plant Foods Human Nutrition 58(3): 1-9.
- Campbell-Platt G (1980) Microbiology of dawadawa- A West African fermented food made from African locust beans (Parkiaspecies), Ph.D thesis, University of Strathclyde, Glasgow, UK.
- Oshodi A, Olaofe AO, Hall GM (1993) Amino acid, fatty acids and mineral composition of pigeon pea (Cajanuscajan) In. J Food Sci Nutr 43(4): 187-191.
- Onimawo IA, Akpojovwo AE (2006) Toasting (Dry heat) and nutrient composition, functional properties and antinutritional factors of pigeon pea (Cajanuscajan). J Food Process Pres 30(6): 742-753.
- Halling PJ (1981) Protein-stabilized foams and emulsions. Crit Rev Food Sci Nutr 15(2): 155-203.
- Aluko RE, Yada RY (1995) Structure-function relationships of cowpea (Vignaunguiculata) globulin isolate: influence of pH and NaCl on physicochemical and functional properties. Food Chem 53(3): 259265.
- Morr CV, German B, Kinsella JE, Regensten JM, Van Buren JP, et al. (1985) A collaborative study to develop a standardized food protein solubility procedure. J Food Sci 50(6): 1715-1718.
- Sosulski FW, Humbert ES, Bui K, Jones JO (1976) Functional properties of Rapeseed flour concentrates and isolates. J Food Sci 41(6): 13481354.
- Naczk, M, Diosady LL, Rubin LJ (1985) Functional properties of conola meals produced by a two-phase solvent extraction system. J Food Sci 50(6): 1685-1689.
- Lin MP, Leeder GJ (1974) Mechanism of emulsifier action in an ice cream system. J Food Sci 39(1): 108-111.
- AOAC (2006) Official Method of Analysis of the AOAC. (W Horwitz Editor) (18th edn.), Washington DC, USA.
- 12. Akpata MI, Akubor PI (1999) Chemical composition and selected functional properties of sweet orange (Citrussinensis) seed flour. Plant Foods Hum Nutr 54(4): 353-362.
- Ragab DM, Babiker EE, Eltinay AH, (2004) Fractionation, solubility and functional properties of cowpea (Vignaunguiculata) proteins as affected by pH and /or salt concentration. Food Chem 84(2): 207-212.
- Kaur M, Singh N (2007) Characterization of protein isolates from different Indian chickpea (Cicerarietinum L.) cultivars. Food Chem 102(1): 366-374.
- Kuntz ID (1971) Hydration of macromolecules III. Hydration of polypeptides. J Am Chem Soc 93(2): 514-515.
- Sathe SK, Deshpande SS, Salunkhe DK (1982) Functional properties of winged bean (Psophocarpustetragonolobus L. DC) proteins. J Food Sci 47(2): 503-509.
- Fernandez-Quintela A, Macarulla MT, Del Barrio AS, Martinez JA (1997) Composition and functional properties of protein isolates obtained from commercial legumes grown in northern Spain. Plant Foods Hum Nutr 51(4): 331-342
- El-Adawy TA (2000) Functional properties and nutritional quality of acetylated Succinylated mung bean protein isolate. Food Chem 70(1): 83-91.
- Mizubuti IY, Junior OB, De-Oliveira-Souza LW, Da Silva RS, Elzalouko I (2000) Functional properties of pigeon pea (Cajanuscajan L.) flour and protein concentrate. Arch Latinoam Nutr 50(3): 274-280.
- Moure A, Sineiro J, Dominguez H, Parajo JC (2006) Functionality of oilseed protein products. Food Res Int 39(9): 945-963.
- Akintayo ET, Oshodi AA, Esuoso KO (1999) Effect of ionic strength and pH on the foaming and gelation of pigeon pea (Cajanuscajan) protein concentrates. Food Chem 66(1): 51-56.
- Mwasaru MA, Muhammad K, Bakar J, Yaakob B, Man C (2000) Influence of altered solvent environment on the functionality of pigeon pea (Cajanuscajan) and cowpea (Vignaunguiculata) protein isolates. Food Chem 71(2): 157-165.
- Wiltoon PR, Larry K, Beuchat, Dixon P (1997) Functional properties of cowpea (Vignaunguiculata) flour as affected by soaking, boiling and fungal fermentation. J Food Sci 45(2): 480-486.
- Oshodi AA, Ipinmoroti KO, Adeyeye EI (1997) Functional properties of some varieties of African Yam bean (Sphenostylissternocarpa) flour III. Int J Food Sci Nutr 48(4): 243-250.
- Ige MM, Ogunsua AO, Oke OL (1984) Functional properties of the proteins of some Nigerian oil seeds, conophor seeds and three varieties of melon seeds. Journal of Agric. And Food Chem 32(4): 822-825.
- Del Rosairo R, Flores MJ (1981) Functional properties of the African yam bean flour (Sphenostylissternocarpa). Journal Sci Food Agric 32:175.
- Olaofe O, Arogundade LA, Adeyeye EI, Falusi OM (1998) Composition and food properties of the variegated grasshopper (Zonocerusvariegatus). Tropical Science 38(4): 233-237.
- Makri E, Papalamprou E, Doxastakis G (2005) Study of functional properties of seed storage proteins from indigenous European legume crops (lupin, peas, broad bean) in admixture with polysaccharides. Food Hydrocolloids 19(3): 583-594.
- Fuhrmeister H, Meuser F (2003) Impact of processing on functional properties of protein products from wrinkled pea. J Food Eng 56(2-3): 119-129.
- Mwasaru MA, Muhammad K, Bakar J, Yaakob B, Man C (1999) Effects of isolation technique and conditions on the extractability, physicochemical and functional properties of pigeon pea (Cajanuscajan) and cowpea (Vignaunguiculata) protein isolates. I. Physicochemical properties. Food Chem 67(4) : 435-444.
- Kinsella JE, Damodaran S, German B (1985) Physicochemical and Functional properties of Oil seed Proteins with emphasis on Soy proteins. In: New protein Foods, USA, pp. 107-179.
- Adebowale KO, Lawal OS (2003) Foaming, gelation and electrophoretic characteristics of mucuna bean (Mucunapruriens) protein concentrates. Food Chemistry 83(2): 237-246.
- FAO/WHO/UNU (1985) Expert consultation. Energy and protein requirements.
- AOAC (1990) Official methods of analysis, Association of Official Analytical Chemists. (15th edn.), Washington DC, USA.
- FAO/WHO, Nutrition Meetings, Report series No. 724, FAO/WHO, Geneva, USA
- Alschul AM, Wilke HL (Eds.), Volume 5, New York, Academic press, USA, pp. 107-179.
- Alschul AM ,Wilke HL (Eds.), Volume 5, New York, Academic press,USA ,pp.107-179.