Human Mesenchymal Stromal Cells, in the Presence of -Interferon Inhibit Jurkat T Cell and U937 Monocytic Cell Proliferation In Vitro
Vincent Kindler1,2*
1Department of Specialties in Medicine, Hematology Service, Geneva University Hospitals, Switzerland
2 Department of Orthopedic Surgery, University of Geneva, Switzerland
Submission: June 21, 2018; Published: July 13, 2018
*Corresponding author: Vincent Kindler, Department of Orthopedic Surgery, University of Geneva, Switzerland.
How to cite this article: Vincent Kindler. Human Mesenchymal Stromal Cells, in the Presence of -Interferon Inhibit Jurkat T Cell and U937 Monocytic Cell Proliferation In Vitro. Open Acc Blood Res Trans J. 2018; 2(4): 555591. DOI: 10.19080/OABTJ.2018.02.555591
Abstract
Human mesenchymal stromal cells (MSC) control activated T lymphocytes in vitro thanks to the activation of the indoleamine 2,3 dioxygenase enzyme degrading L-tryptophan (tryp). MSC are believed to play a key role in self tolerance maintenance as well as in acute inflammation resolution, but their impact upon dormant malignant cell activation or residual disease relapse remains fuzzy. We explored this issue in vitro using the human Jurkat (T cell) and U937 (monocytic) cell lines as models for leukemic disease, and peripheral blood T lymphocytes.
When coincubated with target cells MSC inhibited proliferation and DNA synthesis, and increased the fraction of apoptotic and G1 cells. This occurred only when MSC were exposed to-interferon either before or during coincubation with target cells. The inhibition correlated with the level of tryp depletion in cultures. Leukemic and normal cell incubation in low tryp concentrations recapitulated the inhibition initially observed in cocultures. Tryp half maximal inhibitory concentrations (IC50) were 1.2μM for Jurkat, 8.5μM for U937 and 2.1μM for normal T lymphocytes. Thus, Jurkat could survive unaffected by tryp depletion in conditions whereby normal T cells were inhibited, while U937 were inhibited in conditions whereby T cells remained active.
Altogether our data suggest that the tryp deprivation induced by MSC is a key element to control tumor fate, but that the later also relies upon the sensitiveness of both the effector and malignant cells toward tryp depletion.
Keywords: L-tryptophan; MSC; IDO; IC50;-interferon; leukemic cells; T lymphocyte inhibition;Tumor escape;Immunosuppression
Abbrevations: tryp: L-tryptophan; kyn: L-kynurenine; HPL: Human Platelet Lysate; ITS: Insulin, Transferrin, Selenious Acid;Bovine Serum Albumin;Linoleic Acid Premix
Introduction
Human mesenchymal stromal cells (MSC) result from the in vitro amplification in media supplemented with fetal calf serum, platelet-derived growth factor, or human platelet lysate (HPL) of pericytes and/or adventitial cells residing in vivo around the microvasculature[1-4]. After in vitro amplification MSC exhibit microfilaments composed of alpha smooth actin and myosin[5] and, are able to contract if further seeded in 3-dimension (D) compliant collagen-foam membranes, thus exhibiting functional features of myofibroblasts. Once shifted from HPL to specific media, MSC amplified in either 2-D or 3-D cultures further differentiate into various cell lineages, including adipocytes, chondrocytes and osteoblasts[6]. In both 2D and 3D cultures MSC control the proliferation of activated T lymphocytes. MSC are thus believed to play a key role in the maintenance of self tolerance and in the resolution of inflammatory processes induced by tissue injury or trauma [7]. One of the major molecular factor mediating MSC-driven regulatory function is the enzyme indoleamine 2,3 dioxygenase (IDO), which degrades the essential amino acid L-tryptophan (tryp) into L-kynurenine (kyn)[8-10]. The enzyme is activated upon the exposure of MSC to various inflammatory factors including -interferon ( -IFN) which is expressed by activated T lymphocytes and natural killer (NK) cells[11,12]. lDO can degrade tryp to such an extend that T lymphocyte, B lymphocyte, and NK cell proliferation, whose initiation and sustain rely upon tryp concentration is either prevented or arrested[9]. Moreover, in vitro, human MSC activated with-IFN suppress the proliferation of various pathogens including bacteria, protozoal parasites and virus via IDO activation[13]. Thus, the tryp depletion associated with the activation of MSC during an inflammatory response allows first the elimination of invading microbes, and subsequently the resolution of the inflammatory process via the inhibition of the residual pathogen-specific T cells. The apparently paradoxical inhibitory role of human MSCs upon both microbial growth and pathogen specific T-cells can be reconciled by the observation that the degree of tryp depletion required for suppression of microbial growth is far less stringent than that required for T-cell inhibition. Thus pathogen-specific T cells can remain active and can collaborate with MSC while the pathogen is still present[14].
Due to the to control they exert on T lymphocytes, MSC have been assessed in human clinics for their ability to inhibit steroid-resistant graft-versus-host disease (GVHD)[15]. GVHD is a pathology that develops in some patents reconstituted with an allogenic hematopoietic stem cell graft. Such grafts can contain highly alloreactive T lymphocytes (i.e. T lymphocytes recognizing the patient tissues as targets to be destroyed), which may be activated in the patient. If alloreactive cell activation is too strong, it can induce patient death. So far the results of immunotherapies using MSC in such a context are encouraging, and several studies indeed documented the resolution of the GVHD in allografted patients after allogenic MSC infusion[15,16].
However, as the inhibitory effect of MSC upon T lymphocytes is not specific for alloreactive T lymphocytes and may also extend to residual disease-specific T lymphocytes, it may well be that MSC infusion, while rescuing patient from GVHD may as well favor residual disease relapse[17]. Alternatively, one cannot exclude that MSC, as it is the case for pathogens[13], could directly target the residual disease and neutralize it even in the absence of functional leukemic cell-specific T lymphocytes.
In order to investigate this issue we used the immortalized human Jurkat T cell line (Jurkat) and the immortalized human U937 monocytic cell line (U937) as a models for a residual disease of lymphocytic and monocytic lineage respectively. We investigated whether Jurkat and U937 proliferation and survival in vitro were altered by MSC (activated or not with -IFN), and whether IDO activation was involved in such a process. Finally, we compared the data obtained with malignant cells with these of normal peripheral T lymphocytes.
Materials and Methods
Reactants
Cytokines and chemicals: -IFN was from R&D system Europe, Abingdon, UK. Hank’s balanced salt solution (HBSS), Roswell Park Memorial Institute (RPMI) 1640 medium, Iscove’s modified Dulbecco’s medium (IMDM,) penicillin-streptomycin trypsin- EDTA solution were from Gibco BRL, Paisley, U.K. ITS consisting in insulin, transferrin, selenious acid, bovine serum albumin, linoleic acid premix (ITS), 7-amino actinomycin D (7AAD) and L-tryptophan were from Sigma-Aldrich Missouri, USA). Microbeads coated with anti-CD3 and anti-CD28 antibodies were from Dynal Biotech ASA, Oslo, Norway. Spherotech microbeads were from Spherotech Inc, Lake forest, Illinois, USA. Batch Ae01 contained a concentration of approximately 1.2 x 106 beads/ml.
Antibodies
FITC labeled: Anti-CD45 (clone J33 mIgG1) was from Immunotech, Marseille, France; anti BrdU (clone B44 IgG1) was from Becton-Dickinson, San Jose, CA, USA.
RPE-labeled: Anti-CD3 (clone UCHT-1, mIgG1), and anti-CD14 (clone Tuk4, mIgG2a) were from DakoCytomationGlostrup, Denmark. Anti Ki67 (clone B56) was from BD-Pharmingen Europe.
MSC amplification and cocultures with Jurkat or U937
MSC were purified from femoral head remains after informed consent of patients undertaking hip surgery[18], and amplified in RPMI medium complemented with 5% HPL up to passage 2 or 3[6]. Once amplified, MSC phenotype was routinely checked by flow cytometry (not shown). MSC were then seeded at 6 x104 in 24-well plates in 500μl of RPMI with 5% of HPL for 48 hours. A control well for each series of experiments was trypsinated to establish the MSC content at the time of leukemic cell seeding. This averaged to 1.5 x 105 MSC/well. Wells containing adherent MSC were then washed and filled with 500μl of RPMI containing 10% fetal calf serum (FCS). One to 5 x 104Jurkat or U937 were seeded either directly on MSC or were deposited in transwells of 0.4μM pore size (Costar, Corning incorporated, Corning, NY, USA) above the MSC. In this setting, the volume of the cultures was adjusted to 600μl. The initial ratio of leukemic cells to MSC ranged from 1:15 to 1:3. Co-cultures were performed with or without 250μ/ml gamma interferon (-IFN) for 48 hours before further analyses. In some cases highly purified peripheral blood T lymphocytes activated for 48 hours with microbeads coated with anti-CD3 and anti-CD28 antibodies were added upon MSC. Jurkat, U937, and activated T lymphocytes were also cultured for 48 hours in RPMI medium without tryp, complemented or not with graded amounts of tryp to assess cell sensitivity toward tryp. These cultures were performed in the absence of serum to avoid tryp contamination from FCS. These cultures were supplemented with 0.1 % bovine serum albumin (BSA) and ITS.
Cell cycle and DNA synthesis determination
Cell cycle status of the leukemic cells was assessed by flow cytometry after ethanol fixation and using 7-AAD as described [19]. DNA synthesis was assessed by flow cytometry after the addition of BrdU as described by Ramassary et al. [20]with the exception that 7AAD instead of propidium iodide was used to stain DNA after cell permeabilization. All flow cytometry analyses were performed on an AccuriC6 cytometer (Becton- Dickinson, Mountain View, CA, USA).
Biochemical analyses
Tryp and kyn titers were determined as described[21,22] respectively. Tryp was determined by fluorescence of the cleared culture supernatants after exposure to formaldehyde and FeCl3 on a Twinkle LB970 plate fluorimeter (Berthold technologies, Regendsorf, Switzerland) with λex=355nm and λem=460nm. Kyn was determined by absorbance of the culture supernatant at 490nm on a Vmax ELISA plate reader (Molecular Devices Corporation, Menlo Park, CA) after precipitation with trichloroacetic acid and incubation with Ehrlich reagent.
Cell metabolism was established using the XTT assay according to manufacturer instructions. Briefly 100μl of XTT (2,3-Bis-(2-Methoxy-4-Nitro-5-Sulfophenyl)-2H-Tetrazolium- 5-Carboxanilide) was added per each well containing a 200μl culture. After a 4-hour incubation at 37°C, 200μl of supernatant were removed and the absorbance was measured at λ=450nm using an ELISA plate reader with a reference at λ=650nm.
Effect of graded concentrations of tryp upon cell survival and proliferation in absence of MSC
The sensitivity of cells toward tryp depletion was determined using tryp-free, serum free RPMI medium complemented with graded concentrations of the amino acid, and supplemented with 0.1% BSA and ITS. The effect tryp depletion was determined either using the XTT assay to assess overall cell metabolism, or using flow cytometry to assess cell cycle variations and DNA synthesis. Fluorescent microbeads were introduced in the analyses simultaneously with the cells in order to quantify the absolute number of cells in the tube submitted to flow cytometry, as previously described [23]. Briefly, cells were deposited in eppendorf conical tubes and centrifuged after cell permeabilization and staining with anti-BrdU antibody. The supernatant was disregarded and 100μl of the FACS buffer containing either 1 or 3x 104 spherotech fluorescent microbeads and 7AAD were added in each tube. Tubes were then analyzed on the Accuri C6 analyzer. Appropriates gates were designed to eliminate debris and dead cells. The total number of live cells, apoptotic cells, G1 cells and SG2M cells were recorded. The absolute number of each of such populations was computed according to the following formula:

For tryp IC50 determination, the ratio of counted cells/ counted beads was computed for each tryp concentration analyzed.
Statistics
Mann-Whitney nonparametric rank test was used when 2 sets of data were compared and the Gaussian distribution of samples could not be assessed. A value of p<0.05 was considered as significant. For multiple comparisons, ANOVA with the Bonferroni correction was used after confirmation of the Gaussian distribution of the data. Means ± SDs are shown if not stated differently. IC50 of tryp was computed using the log/logit fitting algorithm of the ATT bioquest IC50 calculator available on Internet (https://www.aatbio.com/tools/ic50-calculator/).
Results
MSC inhibit Jurkat and U937 cell proliferation
Preliminary experiments showed that Jurkat and U937 cells cocultured with MSC that had been previously activated for 48 hours with -IFN exhibited a defect in cell proliferation compared to control cultures. Mean cell output, which was after 48 hours of culture in control conditions 3.9 ± 0.6-fold and 4.6 ± 0.9-fold (n=7) of the input value for Jurkat and U937 respectively, dropped to 0.4 ± 0.1-fold and 0.3± 0.01-fold when cells were cocultured in the presence of activated MSC(n=5, p<0.01 according to Man-Whitney rank test- relative to control conditions). The percentage of apoptotic cells was increased and the percentage of cells in the S-G2M phases was decreased (Figure 1A, left panels). Labeling with the anti-Ki67mAb, which identifies a marker associated with cell cycling, showed that Jurkat cocultured with -IFN-activated MSC contained a smaller Ki67-positive cell fraction compared to the other conditions (Figure 1A, right panels). MSC that had not been exposed to -IFN did not induce such effects (Figure 1A median panels).
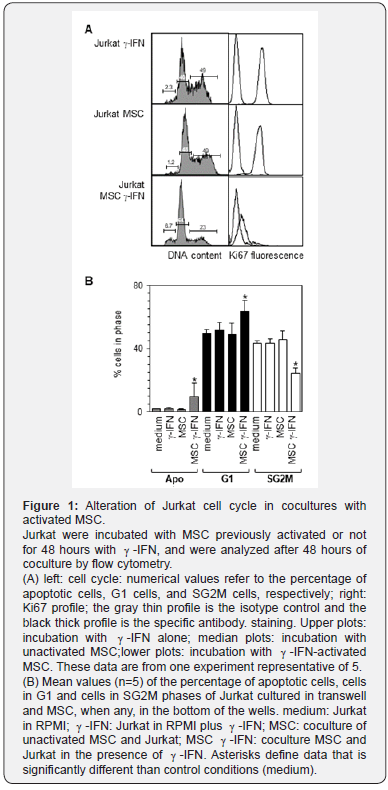
In order to determine whether such an inhibition required a cell-cell contact or whether it was mediated by soluble factors, a series of 5 cocultures were undertaken whereby MSC were coated in the bottom of 24 well plates and Jurkat were seeded over in transwells comprising a membrane with a 0.4 micron porosity. This showed that MSC in the absence of contact and in the presence of -IFN, significantly increased the fraction of apoptotic and Jurkat in the G1 phase, while it decreased the fraction of cells in the SG2M phases (p values of 0.03, 0.01, and 1 x 10-5 respectively according to ANOVA corrected according to Bonferroni (Figure 1B). Similar observations were also done in cocultures of MSC and U937 (not shown).
Quantitative flow cytometry (realized by adding known amounts of fluorescent beads to the samples immediately prior to analysis) showed that Jurkat proliferation was not altered by -IFN complementation nor by culturing in transwells when cells were cultured alone (Figure 2 upper left panel). The coculture with MSC in absence -IFN did not impair cell proliferation. Proliferation inhibition was observed only when Jurkat or U937 were cultured in the presence of MSC and -IFN for 48 hours. In these conditions the total number of cells recovered was under the seeding values (Figure 2 upper left panel). Moreover, the number of cells in G1 and in SG2M decreased for both cell types (Figure 2 upper right and lower left panels). By contrast, the absolute number of apoptotic cells was not altered in cultures with MSC and -IFN.
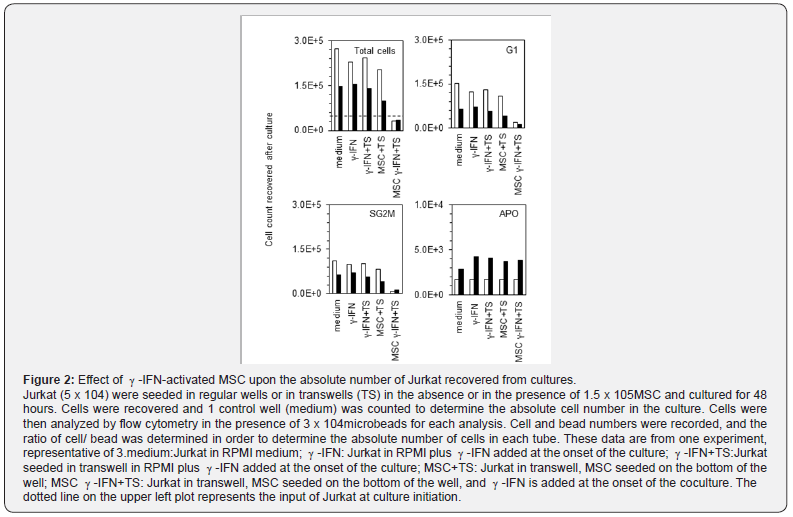
MSC-induced proliferation inhibition correlates with a decrease of tryp titer in the cultures
The catabolism of tryp by IDO leading to kyn production is one of the processes associated with the inhibition exerted by MSC in vitro upon T lymphocytes[9,12]. We therefore assessed tryp titers in culture supernatants of Jurkat or U937 cells after culture in various conditions. A significant decrease of tryp was observed (p<0.005, n=4) compared to the other culture conditions when MSC were cocultured in the presence of -IFN with leukemic cells (Figure 3A). Thus we further investigated whether very low tryp concentrations modified the proportion of Jurkat and U937 in the apoptotic, G1, and SG2M phases of the cell cycle. Indeed tryp deprivation by itself was sufficient to decrease significantly the fraction of cells in the SG2M phase compared to tryp replete cultures (p<0.007, n=3). A trend toward an increase of apoptotic cell fraction and in the G1 cell fraction, although not statistically significant was observed also observed in low tryp concentration (Figure 3B). Complementation of the tryp replete cultures with kyn was not inhibitory. (Figure 3B).
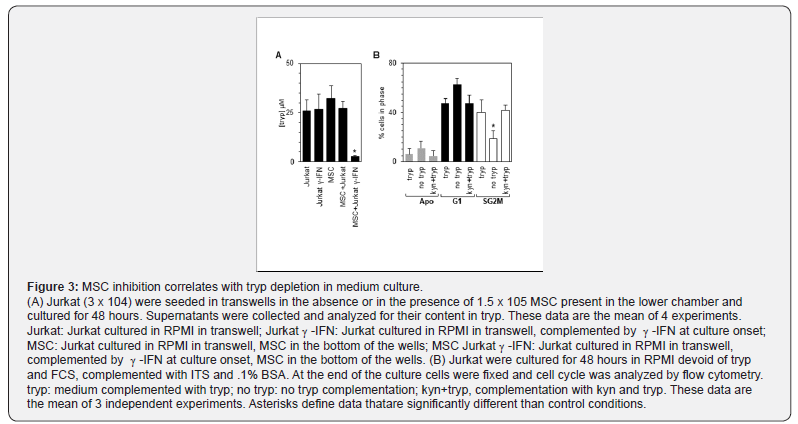
Jurkat exhibit an IC50 for tryp which is smaller compared to normal T lymphocytes
We exposed Jurkat and U937 to tryp concentrations ranging from 0.1μM to 24μM and incubated them for 48 hours. This showed that the number of leukemic cells recovered after culture decreased with decreasing tryp titers (Figure 4A). Jurkat were less sensitive than U937 toward low concentrations of tryp as determined by tryp IC50 computation (Figure 4B). The IC50 of cell proliferation, BrdU incorporation, apoptotic cell fraction, and SG2M cell fraction variations were determined for Jurkat and U927. These data are summarized in Table 1. All the aforementioned biological parameters were altered by the decrease of tryp concentration: the total cell number recovered, the percentage of BrdU-positive cells and the fraction of cells in the SG2M fraction decreased in correlation with tryp titers, while the percentage of apoptotic cells increased when tryp titers decreased. Altogether the tryp IC50 of these various parameters were lower for Jurkat compared to U937, indicating that the former cells were more resistant to low tryp concentrations. We also assessed the impact of tryp depletion upon normal peripheral blood T lymphocytes stimulated for 48 hours with beads coated with anti-CD3 and anti-CD28mAb before being exposed to graded concentration of tryp. T cell global metabolic activity (Figure 4C), as well as the proportion of cells in the SG2M phase decreased in correlation with the decreased concentrations of tryp in the medium. Moreover, computation of IC50 showed that normal peripheral blood T lymphocytes, compared to Jurkat exhibited higher IC50s for these 2 parameters (Table 2) indicating that they were more sensitive to tryp depletion than Jurkat.
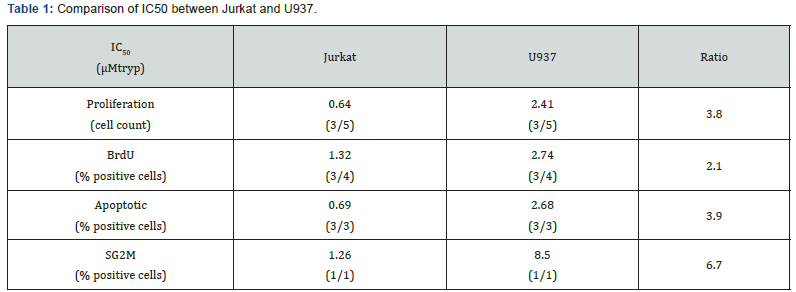
IC50 were computed for the various parameters displayed in the Table using the ATT bioquest IC50 calculator. A series of 3 independent experiments were used to compute the IC50 when enough data were available. Numbers in the brackets are the number of experiment used to establish the IC50 over the total number of experiments realized.
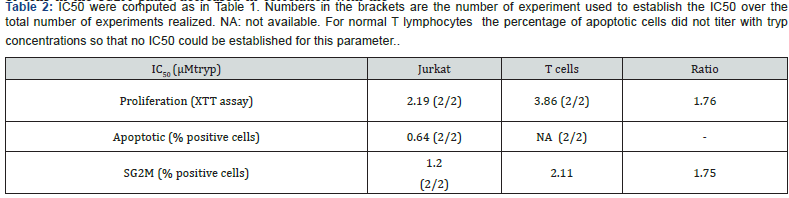
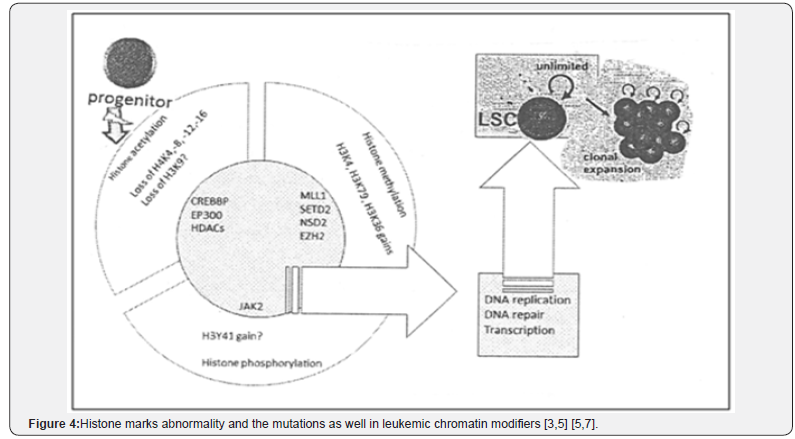
Discussion
This study showed that human MSC can control in vitro the proliferation of immortalized leukemic cells lines like the T cell line Jurkat, and the monocytic cell line U937. The mechanism of inhibition was similar to that observed upon non-malignant T lymphocytes or NK cells[9,11,12], in that it is mediated essentially by tryp depletion secondary to the activation of IDO in MSC by -IFN. According to the IC50 computed, Jurkat were found to be the most resistant to tryp depletion. Tryp depletion secondary to MSC activation by -IFN correlated with a decreased global cell proliferation, metabolic activity (as seen by quantitative flow cytometry and XTT assays, respectively), and DNA synthesis (BrdU incorporation) of the leukemic cells. Moreover, MSC impacted the various compartments of the cell cycle. In terms of percentages, it increased the percent of apoptotic cells and G1 cells, and decreased the percent of SG2M cells. These data are in agreement with that of Ramasami et al.[20], which showed similar alterations of BrdU incorporation and cell cycle upon the coincubation of human MSC with leukemic cell lines[20].
IDO activation is often associated with oncogenic events. Rather surprisingly, it seems to be involved both in tumor regression and in tumor relapse[24]. The present study underlined the inhibitory effect of IDO upon tumor cell growth when MSC are preactivated by- or in the presence of -IFN. The effect of -IFN was thus indirect in our study because it targeted the MSC, which in turn impaired malignant cell proliferation via tryp depletion. However other studies have reported that -IFN, when provided in absence of MSC to tumor cells that can express IDO, may induce tumor cell proliferation inhibition in a kind of “suicidal” mechanism via the activation of endogenous IDO in the cancer cells[25,26]. Several studies reported that IDO activation was also associated with tumor regression in vivo in mice[27,28]. These data contrast with other studies which reported that MSC co-injected with tumors in mice models induced an increase in tumor progression[20,29]. The field remains thus controversial and seems to be model-dependent.
In humans, IDO is spontaneously overexpressed in some malignant cells such as colon and hepatic carcinoma, but the impact of IDO expression over patient survival remains unclear[24]. Concerning the “therapeutic” activation of IDO via either -IFN injection or MSC infusion to patients, the results are contrasted: -IFN in combination with chemotherapy prolonged disease-free survival of patients[30,31]. By contrast, the infusion of MSC to control steroid-resistant GVHD seems to be associated with an increase of relapse in some patients[17]. Thus, as for the mice models, the impact of IDO activation upon the fate of human malignant cells remains largely controversial.
Conclusion
Perhaps, the key factor determining the fate of malignant cells exposed to IDO is not the fact that the enzyme is active or not. Rather, it may be related to the level of typ depletion achieved and the sensitiveness of the various cells or organisms involved in the rejection reaction. As reported for pathogen elimination by MSC [13], whereby elimination is successful when the microorganism exhibits a higher sensitiveness toward tryp depletion than the effector cells, one can speculate that activated MSC will help the eradication of tumor cells if these exhibit a sensitiveness toward tryp depletion that is higher than that of tumor-specific cytotoxic T lymphocytes or NK cells. By contrast, if the tumor cells are more resistant to tryp depletion than immunocompetent effector cells, one may expect that MSC activation will favor tumor escape.
However, one should keep in mind that tumor escape is generally the result of a prolonged process of tumor mutation in a selective environment[32,33]. Most likely MSC may, throughout one’s individual life, eliminate successfully a large number of tumor cells early upon their emergence, in a very silent way before they acquire a resistance toward tryp depletion, like the innate system neutralizes a large burden of pathogens every day without detectable side effects. Thus, the facilitation of tumor escape by MSC, although being armful to the host, may be a rare adverse event, which should not occult that IDO and MSC may play a key role in the containment of early malignancies during one’s individual life.
Acknowledgement
The author would like to thank Mrs. Nicolette Brouwers for excellent technical work , who was instrumental in the genesis and completion of this study. A special thank to Dr José-Maria Rincon-Garriz for preliminary input. This work was supported in part by the CANSEARCH Foundation and the Dr Henri Dubois- Ferrière Dinu Lipatti Foundation, both located in Geneva, Switzerland.
References
- Doucet C, Ernou I, Zhang Y, Llense JR, et al. (2005) Platelet lysates promote mesenchymal stem cell expansion: a safety substitute for animal serum in cell-based therapy applications. J Cell Physiol 205(2): 228-236.
- da Silva Meirelles L, Chagastelles PC, Nardi NB (2006) Mesenchymal stem cells reside in virtually all post-natal organs and tissues. J Cell Sci 119(Pt 11): 2204-2213.
- Crisan M, Yap S, Casteilla L, Chen CW, Corselli M, et al. (2008) A perivascular origin for mesenchymal stem cells in multiple human organs. Cell Stem Cell 3(3): 301-313.
- Popova AP, Bozyk PD, Goldsmith AM, Linn MJ, Lei J, et al. (2010) Autocrine production of TGF-beta1 promotes myofibroblastic differentiation of neonatal lung mesenchymal stem cells. Am J Physiol Lung Cell Mol Physiol 298(6): L735-743
- Lecarpentier Y, Schussler O, Sakic A, Rincon-Garriz JM, Soulie P, et al. (2018) Human Bone Marrow Contains Mesenchymal Stromal Stem Cells That Differentiate In Vitro into Contractile Myofibroblasts Controlling T Lymphocyte Proliferation. Stem Cells Int.
- Pinchuk IV, Saada JI, Beswick EJ, Boya G, Qiu SM, et al. (2008) PD-1 ligand expression by human colonic myofibroblasts/fibroblasts regulates CD4+ T-cell activity. Gastroenterology 135(4): 1228-1237, 1237 e1-2.
- Mellor AL, Keskin DB, Johnson T, Chandler P, Munn DH (2002) Cells expressing indoleamine 2,3-dioxygenase inhibit T cell responses. J Immunol 168(8): 3771-3776.
- Munn DH, Shafizadeh E, Attwood JT, Bondarev I, Pashine A, et al. (1999) Inhibition of T cell proliferation by macrophage tryptophan catabolism. J Exp Med 189(9): 1363-1372.
- Meisel R, Zibert A, Laryea M, Göbel U, Däubener W, et al. (2004) Human bone marrow stromal cells inhibit allogeneic T-cell responses by indoleamine 2,3-dioxygenase-mediated tryptophan degradation. Blood 103(12): 4619-4621.
- Pradier A, Passweg J, Villard J, Kindler V (2011) Human bone marrow stromal cells and skin fibroblasts inhibit natural killer cell proliferation and cytotoxic activity. Cell Transplant 20(5): 681-691.
- Suva D, Passweg J, Arnaudeau S, Hoffmeyer P, Kindler V (2008) In vitro activated human T lymphocytes very efficiently attach to allogenic multipotent mesenchymal stromal cells and transmigrate under them. J Cell Physiol 214(3): 588-594
- Meisel R, Brockers s, Heseler k, Degistirici O, Bülle H, et al. (2011) Human but not murine multipotent mesenchymal stromal cells exhibit broad-spectrum antimicrobial effector function mediated by indoleamine 2,3-dioxygenase. Leukemia 25(4): 648-654.
- Muller A, Heseler k, Schmidt SK, Spekker K, Mackenzie CR, et al. (2009) The missing link between indoleamine 2,3-dioxygenase mediated antibacterial and immunoregulatory effects. J Cell Mol Med 13(6) 1125-1135
- Le Blanc K, Frassoni F, Ball L, Locatelli F, Roelofs H, et al. (2008) Mesenchymal stem cells for treatment of steroid-resistant, severe, acute graft-versus-host disease: a phase II study. Lancet 371(9624): 1579-1586.
- Le Blanc K, Ringden O (2005) Immunobiology of human mesenchymal stem cells and future use in hematopoietic stem cell transplantation. Biol Blood Marrow Transplant 11(5): 321-334.
- Ning H, Yang F, Jiang M, Hu L, Feng K, et al. (2008) The correlation between cotransplantation of mesenchymal stem cells and higher recurrence rate in hematologic malignancy patients: outcome of a pilot clinical study. Leukemia 22(3): 593-539.
- Suva D, Garavaglia G, Menetrey J, Chapuis B, Hoffmeyer P, et al. (2004) Non-hematopoietic human bone marrow contains long-lasting, pluripotential mesenchymal stem cells. J Cell Physiol 198(1): 110-118.
- Garvy BA, Telford WG, King LE, Fraker PJ (1993) Glucocorticoids and irradiation-induced apoptosis in normal murine bone marrow B-lineage lymphocytes as determined by flow cytometry. Immunology 79(2): 270-277.
- Ramasamy R, Lam EW, Soeiro I, Tisato V, Bonnet D, et al. (2007) Mesenchymal stem cells inhibit proliferation and apoptosis of tumor cells: impact on in vivo tumor growth. Leukemia 21(2): 304-310.
- Bloxam DL, Warren WH (1974) Error in the determination of tryptophan by the method of Denkla and Dewey. A revised procedure. Anal Biochem 60(2): 621-625.
- Kudo Y, Boyd CA (2000) Human placental indoleamine 2,3-dioxygenase: cellular localization and characterization of an enzyme preventing fetal rejection. Biochim Biophys Acta 1500(1): 119-1124
- Sutherland DR, Nayyar R, Acton E, Giftakis A, Dean S, et al. (2009) Comparison of two single-platform ISHAGE-based CD34 enumeration protocols on BD FACSCalibur and FACSCanto flow cytometers. Cytotherapy 11(5): 595-605.
- Lob S, Konigsrainer D, Rammensee HG, Opelz G, Terness P (2009) Inhibitors of indoleamine-2,3-dioxygenase for cancer therapy: can we see the wood for the trees? Nat Rev Cancer 9(6): 445-452.
- Farrar MA, Schreiber RD (1993) The molecular cell biology of interferon-gamma and its receptor. Annu Rev Immunol 11: 571-611.
- Ozaki Y, Edelstein MP, Duch DS (1988) Induction of indoleamine 2,3-dioxygenase: a mechanism of the antitumor activity of interferon gamma. Proc Natl Acad Sci U S A 85 (4): 1242-1246.
- Yoshida R, Park SW, Yasui H, Takikawa O (1988) Tryptophan degradation in transplanted tumor cells undergoing rejection. J Immunol 141(8): 2819-2823.
- Yu WG, Yamamoto N, Takenaka H, Mu J, Tai XG, et al. (1996) Molecular mechanisms underlying IFN-gamma-mediated tumor growth inhibition induced during tumor immunotherapy with rIL-12. Int Immunol 8(6): 855-865.
- Friberg M, Jennings R, Alsarraj M, Dessureault S, Cantor A, et al. (2002) Indoleamine 2,3-dioxygenase contributes to tumor cell evasion of T cell-mediated rejection. Int J Cancer 101(2): 151-155.
- Giannopoulos A, Constantinides C, Fokaeas E, et al. (2003) The immunomodulating effect of interferon-gamma intravesical instillations in preventing bladder cancer recurrence. Clin Cancer Res 9(15): 5550-5558.
- Windbichler GH, Hausmaninger h, Stummvoll W, Graf AH, Kainz C, et al. (2000) Interferon-gamma in the first-line therapy of ovarian cancer: a randomized phase III trial. Br J Cancer 82(6): 1138-1144.
- Poggi A, Musso M, Dapino I, Zocchi MR (2014) Mechanisms of tumor escape from immune system: role of mesenchymal stromal cells. Immunol Lett 159(1-2): 55-72.
- Villalba M, Rathore MR, Lopez-Royuela N, Krzywinska E, Garaude J, et al. (2013) From tumor cell metabolism to tumor immune escape. Int J Biochem Cell Biol 45(1): 106-113.