Effect of Mg2+, Ca2+ and Sr2+ Ions Doping on the Band Gap Energy of ZnO Nanoparticle
Yohannes Abdissa, Khalid Siraj* and Girma Selale
Department of Chemistry, Jimma University, Ethiopia
Submission: December 11, 2017; Published: January 10, 2018
*Corresponding author: Khalid Siraj, Department of Chemistry, College of Natural Sciences, Jimma University P.O. Box 378, Jimma, Ethiopia, East Africa, Email: khalid.chemprof@gmail.com
How to cite this article: Yohannes A, Khalid S, Girma S. Effect of Mg2+, Ca2+ and Sr2+ Ions Doping on the Band Gap Energy of ZnO Nanoparticle. JOJ Material Sci. 2018; 3(4): 555620. DOI: 10.19080/JOJMS.2018.03.555620
Abstract
The main objective of this study was to investigate the lowering of the band gap energy and optical property of ZnO doped with Mg2+,Ca2+ and Sr2+ ions. ZnO nanoparticle was synthesize by adding Zinc acetate to aqueous NaOH solution and subjected to ultrasonic irradiation for 2hr after that a white precipitate was obtained which was filtered and washed with ethanol and de-ionized water and dried in an oven at 60 0C for 3hr. The dried white powder was then calcined at 400 0C for 2hr and subjected to UV-Vis spectrophotometer to characterize the synthesized ZnO nanoparticle. The band gap energy of ZnO undoped and doped with Mg2+,Ca2+ and Sr2+ ionswas determined. From all dopants 0.006M Mg2+ doped ZnO nanoparticles was found to be narrowing band gap more.
Keywords: Alkaline Earth metals; Optical property; ZnO; Dopant
Introduction
Nanocrystalline materials got wide attention due to their unique properties. Transparent conducting oxides like ITO, SnO2, CdO, ZnO, etc., have been widely studied due to their interesting optical and electrical properties. Among these conducting materials, zinc oxide is the most attractive because of its low cost, non-toxicity, chemical stability, and facility to doping with a wide variety of ions [1]. ZnO is promising for many potential applications including thin film transistors [2,3], sensors [4,5], light emitting diodes UV photo detectors [6,7], UV lasers [8,9], and piezoelectric power generators [10,11]. Many potential applications rely on the delicate control over the doping of ZnO materials [12]. Zinc oxide (ZnO) has a wide direct band gap energy which makes it transparent to visible light and is a promising candidate for blue and ultraviolet light emitting devices (LEDs) and lasers. In general, ZnO with a wurtzite structure is an unintentional n-type semiconductor due to the deviation from stoichiometry [13].
The band gap for pure ZnO is found to be Eg=3.35 eV and for V-doped ZnO samples of 1,5 and 9 % V, it is 3.24, 3.25 and 3.30eV, respectively. The band gap of V2O5 is 2.3eV and ZnO is 3.37eV. When V2O5 is doped in ZnO, a mixed oxide of ZnV2O is formed and causes the decrease in the band gap [14]. For Ni-doped ZnO films, the energy gap decreases from 2.95 to 2.72eV as the [Ni]/ [Zn] ratio increases from 0 to 0.02 and then increases to reach 3.22eV for [Ni]/[Zn]=0.04 [15]. The value of the band gap energy of undoped nanocrystalline ZnO nanoparticles was 3.26eV and increased by incorporation of Sb doping as it was 3.309eV for doping 1% Sb, 3.318eV for 3% Sb and 3.329eV for 5% Sb [16]. Alkaline earth metals have received great interest due to their attractive electronic, optical and thermal properties as well as catalytic properties and potential applications in the fields of chemistry, physics, biology, medicine, and material science and their different interdisciplinary fields and therefore, the synthesis and characterization of ZnO doped with alkaline earth metals have attracted considerable attention from a fundamental and practical point of view.
The alkaline earth metals are high in the reactivity series of metals, but not as high as the alkali metals of Group I. The metals of Group II are harder and denser than sodium and potassium, and have higher melting points. These properties are due largely to the presence of two valence electrons on each atom, which leads to stronger metallic bonding than occurs in Group I [17]. This work is aimed at a band gap narrowing by doping into ZnO by alkaline earth metals (Mg2+,Ca2+,Sr2+). UV-Vis experiments were carried out for ZnO undoped and doped with alkaline earth metals. By measuring UV-Vis spectra of the resulting Zn1- xMxO, (x=0.001, 0.003 and 0.006) we tried to investigate how a material's composition affects its electronic band structure and optical properties.
Materials and Method
All chemicals for this research were used as received without any treatment.
Preparation of ZnO nanoparticles
The 50ml of aqueous solution of 0.2M Zinc acetate Zn(CH3COOH)2.2H2O was added to 10ml of 0.2M aqueous NaOH solution and subjected to ultrasonic irradiation for 2hr. The obtained white precipitate was filtered and washed with ethanol and deionized water and dried in an oven at 60 0C for 3hr. Then the sample was calcined at 400 0C for 2hr.
Preparation of Mg2+ Ca2+,Sr2+ doped ZnO nanoparticles
In a typical synthesis, 10ml of Mg2+, Ca2+, Sr2+solution (0.006 M) were added to 0.05g of calcined ZnO. The sample was heated at 110 0C for 30min. The powder cooled to room temperature, and again calcined at 400 0C for 2hrs. The product obtained was labeled as Mg2+, Ca2+ and Sr2+ doped ZnO. The above procedure was repeated for 0.003M and 0.001M Mg2+Ca2+, Sr2+doped ZnO nanoparticles.
Band gap and Optical property determination
The measurement of the absorption spectrum leads to determination of the optical band-gap energy. The optical band gap of the nano powders was determined by applying the Tauc's relationship is given by;
(αhv) = (hv - Eg)n
Where; α is the absorption coefficient (α=2.303A/t, A is the absorbance and t is the thickness of the cuvett), Eg is the energy band gap, hv is the energy of the radiation (photon energy), The value of n=1/2, 3/2, 2, or 3 depending on the nature of the electronic transition responsible for absorption and n=1/2 for the direct band gap of semiconductor [18].
Results and Discussion
Characterization of ZnO nanoparticles

The absorbance of the ZnO nanoparticles solution was measured using a quartz tube. The UV-visible absorption spectra of ZnO nanoparticles samples were recorded in the wavelength range of 250 to 500 nm; it exhibits a strong absorption band at about 380nm, (Figure 1). The size of the nanoparticles plays an important role in changing the entire properties of materials. UV-Visible absorption spectroscopy was widely being used technique to examine the optical properties of Nanosized particles. The average particle size was calculated from the absorption spectra by using effective mass model where the band gap E can be approximated by [19].
Where Egbulk is the bulk band gap expressed in eV, h is Plank's constant, r is the particle radius, me is the electron effective mass, mh is the hole effective mass, m0 is free electron mass, e is the charge on the electron, ε is the relative permittivity, and ε0 is the permittivity of free space. Due to the relatively small effective masses for ZnO (me= 0.26, mh= 0.59, mo= 9.11x10-31Kg, ε0 = 8.85x10-12 F/m, ε = 8.5, Charge of electron = 1.602x10-19C, planks constant = 6.626x10-34 m2kg/s) band gap enlargement is expected for particle radii less than about 4nm. To find the size of the particle using absorbance spectra, the effective mass model, given above, was modified with small mathematical simplification [20],
Where λp is peak absorbance wavelength in nm. The prepared ZnO nanoparticles exhibit an absorbance peak at about 380 nm which corresponds to the particle size of 3.6nm.
UV-Visible spectroscopy and Optical properties studies of doped ZnO nanoparticles

UV-Visible spectra of Mg2+, Ca2+ and Sr2+ doped ZnO nanoparticles were obtained by doping different concentration of metal ions in Zn M O (M= Mg2+,Ca2+,and Sr2+ ; x = 0.001, 0.003, 0.006) [21]. Figure 2, shows the UV-Vis spectra of Mg2+ doped ZnO nanoparticles along with undoped it can be seen from the figure that the absorption peaks position changes from 380nm to 390nm for 0.001M, 391 for 0.003M and 393nm for 0.006M. With increase in Mg2+ concentration, the optical absorption edge slightly shifts towards the longer wave length region which may be attributed to the increase in particle size of ZnO [22]. Figure 3, illustrates that the absorbance plot for various concentrations of Ca2+. It can be observed from the plot that the maxima changes compared from ZnO.


The changes of maxima are 386,387 and 389nm for 0.001, 0.003 and 0.006M respectively. This small shift in absorption band may be due to the doping effect of Ca2+into ZnO [23]. UV- Visible spectra for Sr2+ doped ZnO can be seen from Figure 4. ZnO nanoparticles shows the absorption peak at 380nm and the Sr2+ doped ZnO nanoparticle with different concentration of Sr2+ shows the absorption peaks at 382,383 and 385 nm respectively The optical absorption edge corresponds to the transition from valence band to conduction band, while the absorption edge shifting to the lower energy relates to some local energy levels caused by some intrinsic defects [24].
Comparative study of effect of doping on band gap energy of undoped ZnO nanoparticles
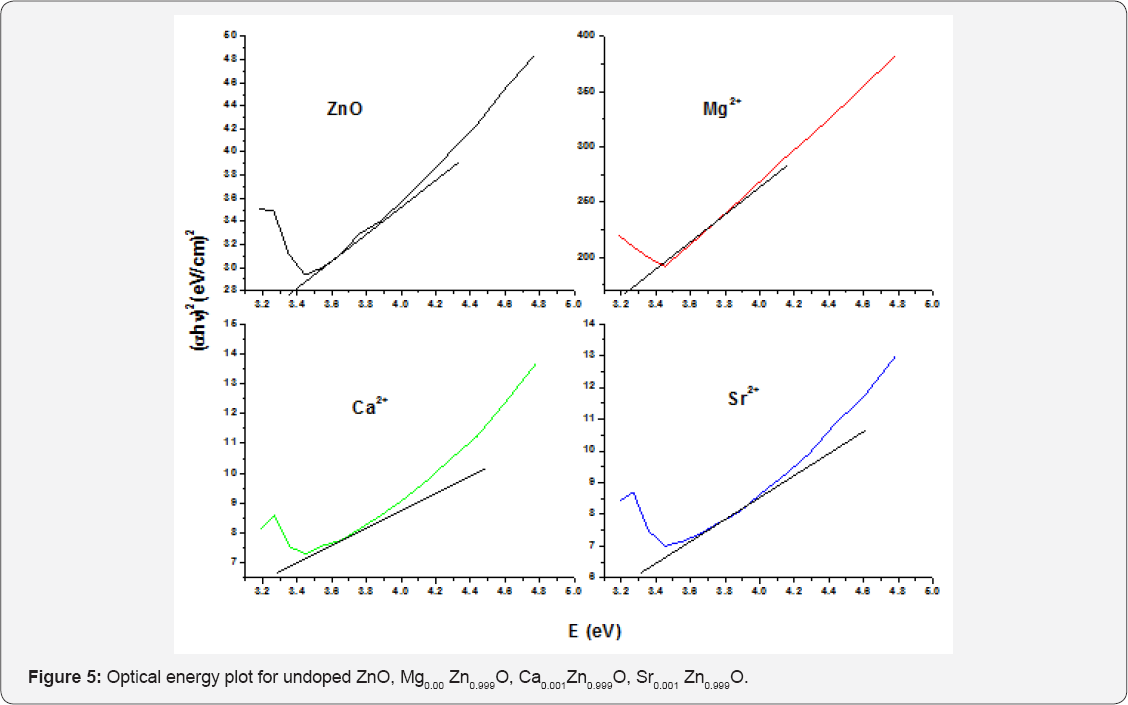
Optical energy plot of pure and Mg2+, Ca2+ and Sr2+ doped ZnO at 0.001M shown in (Figure 5). The absorption coefficient a were calculated and plotted for the direct transmission of the ZnO undoped and doped Mg2+, Ca2+ and Sr2+ at 0.001M concentration. The value of band gap energy of undoped ZnO nanoparticle was 3.263eV. The band gap of a 0.001M Mg2+, Ca2+ and Sr2+ doping was 3.179, 3.212 and 3.245eV respectively. It can be seen that Mg2+ doped narrows the band gap of undoped zinc oxide nanoparticle more than Ca2+ and Sr2+[2 5]. The band gap energies were determined by taking the extrapolated lines from the linear vertical regions near the band edge as shown in Figure 6. The band gap energy for pure ZnO was found to be 3.263eV.

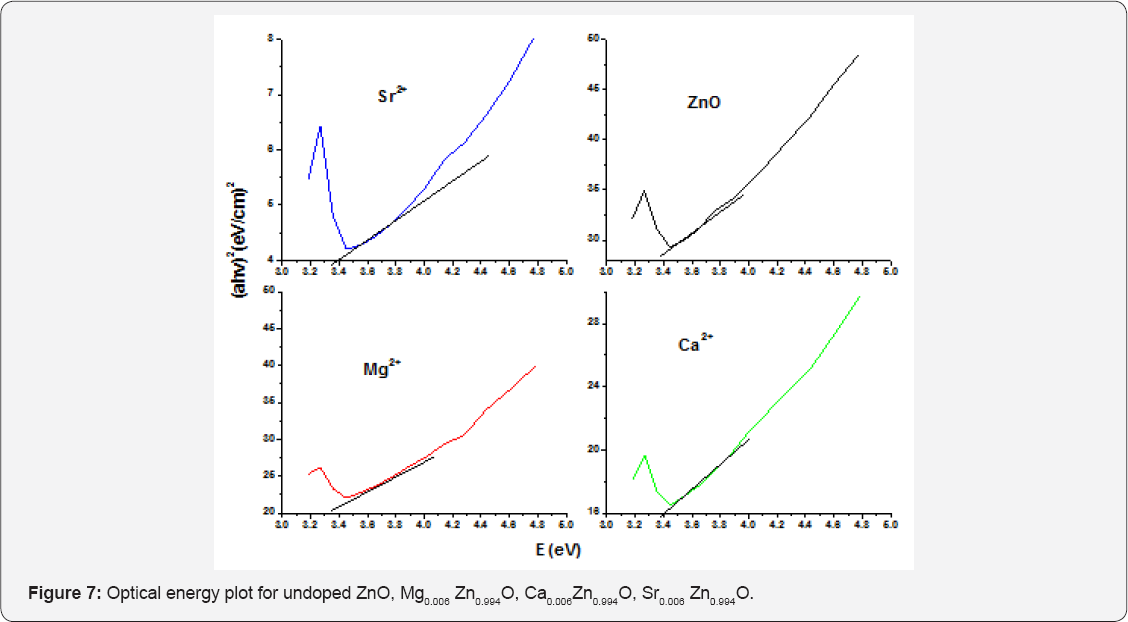
The measured direct band gap energy of ZnO with 0.003M concentration of Mg2+, Ca2+ and Sr2+ was 3.17, 3.203 and 3.22eV respectively. In a 0.003M of Mg2+, Ca2+ and Sr2+ doped ZnO nanoparticle a band gap of Mg2+ with 3.17eV narrows the band gap of undoped ZnO nanoparticle than that of a 3.203eV of Ca2+ doped ZnO nanoparticle and 3.203eV of Ca2+ doped ZnO nanoparticle narrows the band gap undoped ZnO than that of 3.22eV of Sr2+ doped ZnO nanoparticles [26]. The UV-Vis absorption spectra of the pure and 0.006M Mg2+, Ca2+, Sr2+ doped ZnO nanoparticle were investigated at room temperature. As shown in Figure 7, we can find that the optical absorption spectra changes after doping of Mg2+, Ca2+, Sr2+. In order to evaluating the band gap presents the dependence of a as a function of hv for the pure and Mg2+ Ca2+, Sr2+ doped ZnO nanoparticle.
The band gap obtained by extrapolation from the Figure 6 for 0.006M concentration was 3.154, 3.187 and 3.237eV respectively. Since the band gap of Mg2+ was small compared to the band gap of Ca2+ and Sr2+, it narrows the band gap of undoped ZnO nanoparticle, So Mg2+ is a suitable dopant for ZnO nanoparticle and it conducts electricity more than that of Ca2+ and Sr2+. A band gap of 3.187eV of Ca2+ narrows the band gap of undoped Zinc oxide nanoparticles than that of 3.22eV Sr2+ doped ZnO nanoparticle [27]. The band gap of Mg2+ doped ZnO for different concentration was around 3.179, 3.17 and 3.154eV respectively. As the Mg2+ concentration increases the absorption edge slightly shifts towards the longer wave length region which may be attributed to the decrease in band gap. This decrease of the band gap after doping of Mg2+ was probably related to the difference in ionicity between Zn2+ and Mg2+ bonds Tables 1 & 2. Moreover, since ZnO and Mg2+ atoms have strong mismatch in electro negativity was a reason for decrease of the band gap.


It was seen that the energy band gap decreases with the increase dopant conc. of Mg2+ which can be explained as the narrowing of band gap energy is possibly due to the existence of more Mg2+ impurities in the ZnO crystallites, which induces the formation of new recombination centers with lower emission energy [27]. The band gap of Ca2+ doped ZnO for different concentration was around 3.212, 3.203 and 3.187eV respectively With the increase in the Ca2+concentration, the optical absorption edge shifts slightly towards the longer wavelength region which may be attributed to the slightly decreases band gap. The reason for the decrease in the band gap may be explained on the basis of alloying effect between ZnO and Ca2+ [28].
Conclusion
ZnO nanoparticles were successfully synthesized by addition of aqueous solution of zinc acetate dehydrate with aqueous sodium hydroxide. The UV-Visible spectra for ZnO nanoparticle synthesized in aqueous media exhibited excitonic absorption peak at 380nm. The band gap obtained for the synthesized of ZnO nanoparticles was determined by extrapolation to the X-axis was ^3.263eV. From all dopants Mg2+ doped ZnO nanoparticles were showing narrowing more band gap energy compared to Ca2+ and Sr2+ doped ZnO nanoparticle. As the concentration increases, the band gap slightly decreases. This decrease in band gap energy after doping of Mg2+ was related to difference in iconicity between ZnO and Mg2+. It may also due to a strong mismatch of electro negativity in ZnO and Mg2+. The existence of more Mg2+ impurities in ZnO crystallites which induces the formation of new recombination centers with lower emission energy.
Acknowledgement
We are gratefully acknowledging the Department of Chemistry, College of Natural Sciences, Jimma University, Jimma, Ethiopia for providing necessary facilities to carrying out this work.
References
- A Bouaoud, A Rmili, F Ouachtari, A Louardi, T Chtouki, et al. (2013) Transparent conducting properties of Ni doped zinc oxide thin films prepared by a facile spray pyrolysis technique using perfume atomizer, Materials Chemistry and Physics, 137: 843-847.
- R L Hoffman, B J Norrisa, JF Wager (2003) ZnO-based transparent thin- film transistors. Applied Physics Letters 82(5): 733-735.
- K Nomura, H Ohta, K Ueda, T Kamiya, M Hirano, et al. (2003) Thin- film transistor fabricated in single-crystalline transparent oxide semiconductor. Science 300(5623): 1269-1272.
- Q Wan, QH Li, YJ Chen, TH Wang, XL He, et al. (2004) Fabrication and ethanol sensing characteristics of ZnO nano wire gas sensors. Applied Physics Letters 84(18): 3654-3656.
- X Wang, C J Summers, Z L Wang, (2004) Large-scale hexagonal- patterned growth of aligned ZnO nanorods for nano-optoelectronics and nano sensor arrays. Nano Letters 4(3): 423-426.
- A Tsukazaki, A Ohtomo, T Onuma, Makoto Ohtani, Takayuki Makino, et al. (2005) Repeated temperature modulation epitaxy for p-type doping and lightemittingdiode based on ZnO. Nature Materials 4(1): 42-46.
- J Bao, M A Zimmler, F Capasso, X Wang, Z F Ren, et al. (2006) Broadband ZnO single-nanowire light-emitting diode. NanoLetters 6(8): 17191722.
- C Soci, A Zhang, B Xiang, S A Dayeh, D P R Aplin, et al. (2007) ZnO nanowire UV photodetectors with high internal gain. Nano Letters 7(4): 1003-1009.
- Y Jin, J Wang, B Sun, J C Blakesley, N C Greenham, et al. (2008) Solution-processed ultraviolet photodetectors based oncolloidal ZnO nanoparticles. Nano Letters 8(6): 1649-1653.
- K Govender, D S Boyle, P O Brien, D Binks, D West, et al. (2002) Room- temperature lasing observed fromZnO nanocolumns grown by aqueous solution deposition. Advanced Materials 14: 1221-1224.
- Z L Wang, J Song (2006) Piezoelectric nanogenerators based on zinc oxide nano wire arrays. Science 312(5771): 243-246.
- K Ueda, H Tabata, T Kawai (2001) Magnetic and electric properties of transition-metal-doped ZnO films. AppliedPhysics Letters 79(7): 988990.
- Siva Vijayakumar, S K, S Vasanth, Arul Ganesh, G. Bupesh, et al. (2013) Synthesis of Silver Doped Zinc Oxide Nanocomposite by Pulse mode Ultrasonication and its characterization studies. J Nanosci 1-7.
- Joshi R, pramod Kumar, Anurag Gaur, Asokan K (2014) Structural, optical and ferroelectric properties of V-doped ZnO. Appl Nanosci 4: 531-536.
- Mondal S, Mitra P (2013) Preparation of Ni-doped ZnO thin films by SILAR and their characterization. J Phys 87:125-131.
- Shashi R, Amarpal Singh, Satbir Singh (2013) Characterization and optical studies of pure and Sb-doped ZnO Nanoparticle. Int J Nanoelec Mat 6: 45-57.
- Shakhashiri B Z I (2009) A handbook of chemistry for teachers. The university of Wisconsin Press, USA.
- Murugesan, Nirmala, Achuthanunni, Anukaliani (2010) Structural and optical properties of undoped and co-doped ZnO nanostructured thin film. J sci Technol 18: 81-88.
- Satyanarayana Talam, Srinivas Rao Karumuri, Nagarjuna Gunnam (2012) Synthesis, Characterization, and Spectroscopic Properties of ZnO Nanoparticles. ISRN Nanotech: 1-6.
- Louis Brus (1986) Electronic wave functions in semiconductor clusters: experiment and theory. Journal of Physical Chemistry 90(12): 2555-2560.
- Chewki Zegadi, Khalil A, Denis Chaumont, Mohamed Adnane, Saad Hamzaoui, et al. (2014) Influence of Sn Low Doping on the Morphological, Structural and Optical Properties of ZnO Films Deposited by Sol-Gel Dip-Coating. Adv Mat Phy Chem 4: 93-104.
- Saber Farjami shayesteh, Armin Ahmadi (2013) Effect of doping and annealing on the physical properties of ZnO: Mgnanorods. J Phys stat sol 81(2): 319-330.
- Eskandaria, V A M (2012) The Effect of magnesium doping concentration on morphology and optical property of ZnO Nanorods. J.ICNS 4:1-3.
- Elilarassi R, ChandrasekaranG. (2010) Synthesis and optical properties of Ni-doped zinc oxide nanoparticles for optoelectronic applications. Optoelec Let 6: 6-10.
- Ruby Chauhan, Ashavani Kumar and Ram Pal Chaudhary (2010) Synthesis and Characterization of Copper doped ZnO nanoparticles. J chem pharmRes 4: 178-183.
- S Udayakumar, V Renuka, K Kavitha (2012) Structural, optical and thermal studies of cobalt doped hexagonal ZnO by simple chemical precipitation method. J chem and pharm Res 4(2):1271-1280.
- Talaat M Hammad, J K Salem, Roger G Harrison, Rolf Hempelmann, Nasser K Hejazy, et al. (2013) Optical and magnetic properties of Cu- doped ZnO nanoparticles. J Mater Sci Mater Elec 24: 2846-2852.
- Ayadi Z B, El Mir L, El Ghoul J, Djessas K, Alaya S, et al. (2010) Structural and optical properties of calcium doped ZnO sputtered from nano powders target material. International Journal Nanoelectronics and materials 3: 87-97.