SUITABILITY OF MAIZE COB ASH AS A PARTIAL CEMENT REPLACEMENT
John Kamau and Ash Ahmed*
Department of civil engineering, Leeds Beckett University, UK
Submission: August 24, 2017; Published: September 18, 2017
*Corresponding author: Ash Ahmed, Department of civil engineering, Leeds Beckett University, Leeds, England, UK, Email: A.R.Ahmed@leedsbeckett.ac.uk
How to cite this article: John K, Ash A. SUITABILITY OF MAIZE COB ASH AS A PARTIAL CEMENT REPLACEMENT. JOJ Material Sci. 2017; 2(5): 555599. DOI:10.19080/JOJMS.2017.02.555599
Abstract
Cement is the most utilised construction material, and the second most consumed global commodity after water. Its demand has soared proportionately with the exponential rise in population to match required development. The heavily energy-intensive processes involved in its production contribute to about 7 to 10 per cent (%) of the total global emissions, with potentially adverse environmental implications and are expensive economically. These processes and those of concrete production consume heavily on natural resources such as sand, gravel, water, coal and crushed rock, the mining of which mars the environment. It is however possible, that energy and cost efficiency can be achieved by reducing on the amount of clinker, and in its place utilising Partial Cement Replacement (PCR) materials that require less process heating and emit fewer levels of carbon dioxide (CO2). This study investigated the ability of corncob ash to be used as a PCR, by testing for either pozzolanic or cementitious properties. Experiments were carried out by replacing cement by weight in concrete mixes with corncob ash at 5%, 7.5%, 10%, 15% and 20% steps at the point of need. The results were compared with a control specimen made with no cement replacement. Durability was tested using the sulfate elongation test. The highest compressive strength was observed at the 7.5% replacement. However, higher replacement levels also showed impressive strengths suitable for structural applications. The sulfate elongation test results showed good performance for all corn cob ash specimens in comparison to the control mix. These findings showed good reproducibility and highlight the potential of corncob ash as an effective pozzolan.
Keywords: Corncob ash; Pozzolans; Cementitious materials; Maize cob ash; Partial cement replacements
Introduction
Cement, a major constituent of concrete, is pivotal to development and is produced in virtually all countries. One ton of concrete on average is produced every year for every human being in the world. Cement is deemed to have a considerably high carbon footprint, contributing immensely to global anthropogenic CO2 [1-5]. Utilisation of Partial Cement Replacements (PCRs) reduces solid waste, cuts on greenhouse gas emissions and conserves existing natural resources, thereby enhancing sustainability as well as improving the properties of fresh and hardened concrete. This paper investigated the suitability of Corn Cob Ash (CCA) for use as a PCR in Africa, where it is available in abundance [6-10].
Methods
Cement was substituted with PCRs by weight in percentages of 0, 5, 7.5, 10, 15 and 20, with the 0% being the control specimen. For sulfate elongation tests, specimen prisms of 160 x 40 x 40 were cured for seven days before being immersed in 5% Na2SO4, 5% MgSO4 and 5% +5% Na2SO4 and MgSO4. Length change due to sulphates was measured to ASTM-C1012/C1012M (2013) at 133 days. All preparation and testing were done in accordance with BS EN 197-1:2000, BS EN 12390 series and ASTM C1012 [11-15].
Chemical analysis
The sum of SiO2+Al2O3+Fe2O3 for the CCA sample used for this study was 54.1%, and therefore did not satisfy the pozzolanic recommendations of ASTM (2012) and BSI (2000a) of SiO2+Al2O3+Fe2O3 70%, but it did satisfy some requirements of both pozzolanic and cementitious materials. However, the method used to incinerate the CCA may have affected its chemical composition as the CCA used by other researchers achieved these values.
Results and Discussion
Table 1 and Figure 1 show the compressive strengths achieved at different ages with different CCA replacements. The highest compressive strength for the population was found at 7.5%, with maximum stresses of 63.5Nmm-2 recorded at 91 days. Apart from the 20% replacement, all other replacements realised compressive strengths of above the 25N/mm-2 at 28 days. Compressive strength increased with curing age in line with literature, and at 91 days, all replacement levels showed impressive compressive strengths suitable for structural applications. CCA replaced specimens were darker in color and had a lower density than that of the control. The workability of the CCA replaced mixes increased with increased replacement.For the sulfate elongation tests, findings showed the change in lengths for all CCA samples were less than the control sample thus indicating improved sulphate resistance [15-20].
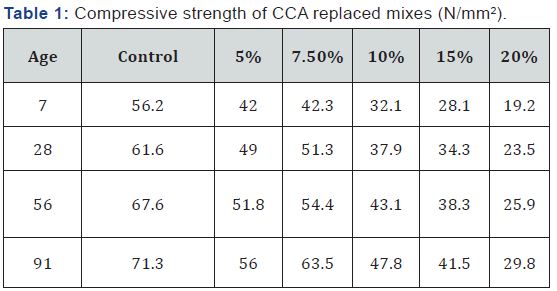
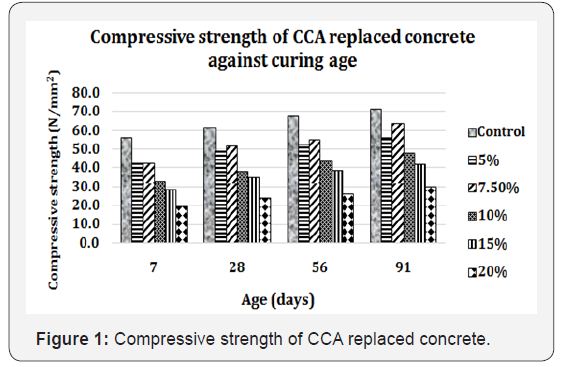
Conclusion
CCA used for the study did not satisfy the minimum chemical composition requirements for pozzolanic materials of SiO2+Al2O3+F2O3≥70%, but it did satisfy some requirements of both pozzolanic and cementitious materials. Compressive strengths observed throughout all replacements were capable of structural applications. The compressive and sulfate resistance tests also showed good repeatability with previous studies, with strengths capable of structural applications observed over all replacements. These results show that CCA can be used as a partial cement replacement to mitigate on the cost of cement and its impacts on the environment whilst also improving sulphate resistance [21-30].
References
- Bapat JD (2012) Mineral admixtures in cement and concrete: CRC Press, USA.
- Mohammed OH, Hamid RB, Taha MR (2012) A review of sustainable supplementary cementitious materials as an alternative to all-portland cement mortar and concrete. Australian Journal of Basic & Applied Sciences 6(9): 303.
- Brien EJO, Dixon AS, Sheils E (2012) Reinforced and Prestressed Concrete Design to EC2: The Complete Process: Spon Press, London, UK.
- Akinboboye F, Ogunfayo I, Dawodu H (2012) Assessment of the severity of CO2 emission from anthill soils used as replacement for shale in cement manufacture 2(12).
- Cline WR (1992) The Economics of Global Warming: Peterson Institute.
- Yerramala A, Desai B (2012) Influence of fly ash replacement on strength properties of cement mortar. International Journal of Engineering Science and Technology p. 4.
- Soyka MJGP, Conneely D CO2 emissions from cement production. Good Practice Guidance and Uncertainty Management in National Greenhouse Gas Inventories.
- Lippiatt B, Ahmad S (2004) Measuring the life-cycle environmental and economic performance of concrete: the BEES approach. Proceedings of the International Workshop on Sustainable Development and Concrete Technology p. 213-230.
- (2016) Worldometers Population.
- Muga H, Betz K, Walker J, Pranger C, Vidor A, et al. (2005) Development of appropriate and sustainable construction materials. Civil and Environmental Engineering Sustainable Futures Institute, Michigan Technology University, Michigan, USA.
- Sakai K, Noguchi T (2012) The Sustainable Use of Concrete: CRC press, USA.
- Al Salami A, Salem A (2010) Effects of mix composition on the sulfate resistance of blended cements, International Journal of Civil & Environmental Engineering 10(6).
- Islam M (2013) Strength and durability characteristics of concrete made with fly-ash blended cement. Australian Journal of Structural Engineering 14(3): 303-309.
- Gambhir (2009) Concrete Technology 4e: Tata McGraw-Hill Education.
- Mehta PK (2001) Reducing the environmental impact of concrete. Concrete International 23: 61-66.
- Johnson ME, Gonzalez A (2013) Estimating cost savings for aviation fuel and CO2 emission reductions strategies. Collegiate Aviation Review 31(2): 79-102.
- Khan R, Jabbar A, Ahmad I, Khan W, Khan AN, Mirza J (2012) Reduction in environmental problems using rice-husk ash in concrete. Construction and Building Materials 30: 360-365.
- Steele K, Cole G, Parke G, Clarke B, Harding J (2002) The application of life cycle assessment technique in the investigation of brick arch highway bridges. Proceedings of Conf. for the Engineering.
- Davison B, Owens GW (2011) Steel Designers’ Manual.
- Malhotra V, Mehta P (2005) High-performance, high-volume fly ash concrete: Materials, mixture proportions, properties, construction practice, and case histories. Supplementary Cementing Materials for Sustainable Development Inc, Ottawa ON, Canada.
- Sengul O, Tasdemir MA (2009) Compressive strength and rapid chloride permeability of concretes with ground fly ash and slag. Journal of materials in civil engineering 21(9): 494-501.
- BSI (2000) BS EN 197-1: 2000. Part 1 Cement composition, specifications and conformity criteria for common cements. British Standards Institution (BSI), London, UK, BSOL.
- BSI (2012) BS EN 12390-1:2012. Testing hardened concrete. Part 1: Shape, dimensions and other requirements for specimens and moulds. British Standards Institution (BSI), London, UK.
- BSI (2009) BS EN 12390-2:2009. Making and curing specimens for strength tests. British Standards Institution (BSI), London, UK.
- Oluokun FA (1994) Fly ash concrete mix design and the water-cement ratio law. Materials Journal 91(4): 362-371.
- ASTM (2015) C1012/C1012M−15. Standard Test Method for Length Change of Hydraulic-Cement Mortars Exposed to a Sulfate Solution1. American Society for Testing and Materials.
- BSI (2000) BS EN 12390-4:2000. Testing hardaened concrete. Compressive strength. Specification for testing machines. British Standards Institution (BSI), London, UK.
- BSI (2004) BS EN 1992-1-1: 2004: Eurocode 2: Design of Concrete Structures‚ Part 1-1: General Rules and Rules for Buildings. British Standards Institution (BSI), London, UK.
- BSI (2015) BS 8500-1:2015:Concrete – Complementary British Standard to BS EN 206. Part 1: Method of specifying and guidance for the specifier. British Standards Institution (BSI), London, UK.
- Oluokun FA (1994) Fly ash concrete mix design and the water-cement ratio law, Materials Journal 91(4): 362-371.