Development of Refined Chemistries and Processing Methods for Integration of Carbon Dioxide into a Hydraulic Binder for Effective Heavy Metal Immobilization
Anagi M Balachandra1*, Kaize Zhu2, Salina Ramli2, Parviz Soroushian1,2, Faris Matalkah2 and Nastaran Abdol1
1Metna Co., 1926 Turner St., Lansing, MI, USA 48906. 2Dept. of Civil and Environmental Engineering, Michigan State University, 3546 Engineering Building, E. Lansing, MI, USA 48824-1226.
Submission: August 08, 2017; Published: August 30, 2017
*Corresponding author: Anagi M Balachandra Metna Co. 1926 Turner Street Lansing, MI 48906, USA, Email: abmetnaco@gmail.com
How to cite this article: Anagi M B, Kaize Z, Salina R, Parviz S, Faris Matalkah, Nastaran A. Development of Refined Chemistries and Processing Methods for Integration of Carbon Dioxide into a Hydraulic Binder for Effective Heavy Metal Immobilization. JOJ Material Sci. 2017; 2(4): 555593. DOI:10.19080/JOJMS.2017.02.555593
Abstract
A method is under developed for capture of carbon dioxide directly from combustion emissions into land filled coal ash, and simultaneous transformation of the carbon dioxide and ash with supplementary minerals into a hydraulic binder. In this study, several additives were investigated for raising the CO2 uptake of land filled ash and enhancing the end-product quality. The resultant binder meets the standard requirements for hazardous waste solidification and stabilization. The approach adopted here relies upon mechanochemical phenomena, induced by a simple milling action, to incorporate carbon dioxide into land filled ash while concurrently transforming the raw materials (including the captured carbon dioxide) into a hydraulic binder. The CO2 uptake in mechanochemical processing of cement was estimated as the difference in Loss of Ignition (LOI) of cements milled in CO2 and in air. The compressive strength achieved by hydration of cement was assessed after 7 days of curing at room temperature. The Toxicity Characteristic Leaching Procedure (TCLP) was used to evaluate the solidification and stabilization qualities of hydraulic cements. FTIR spectroscopy was used in order to get better understanding on the nature of the carbon dioxide incorporated into the hydraulic cement via mechanochemical effects. The results indicated that relatively high concentrations of carbon dioxide (up to 10wt.%) could be incorporated into hydraulic cements with desired hazardous waste stabilization and solidification qualities. The captures carbon dioxide benefited the resultant hydraulic cement performance.
Introduction
Since the beginning of the industrial age, the carbon oxide concentration in atmosphere has increased by about 30% [1,2]. This rise in CO2 concentration has accompanied climate warming with adverse ecological effects. Capture of carbon dioxide for value-added use in hydraulic cement is pursued here to reduce carbon emissions. The use of land filled coal ash as the primary raw material for production of the hydraulic cement developed here offers important environmental benefits. Significant quantities of coal ash, estimated at billions of tons, have accumulated in landfills across the world.
There are concerns with the environmental and health effects of land filled coal ash. Another consequence of human’s industrial activities is generation of hazardous wastes with adverse health and environmental effects. The application pursued for the hydraulic cements incorporating captured CO2 is the immobilization of hazardous wastes. The hydraulic cement developed here also provides important energy and cost savings when compared with the now prevalent Portland cement.
The CO2 capture aspect of this development effort can be viewed as a low-cost and simple variation of the methods employed for mineral CO2 sequestration. The approach devised here employs land filled coal ash together with supplementary minerals, which are transformed into a hydraulic binder in the presence of a dilute carbon dioxide atmosphere which represents combustion emissions. Carbon dioxide acts as gaseous raw materials that, together with the land filled coal ash and solid raw materials, are transformed into a hydraulic binder under input of mechanical energy in a ball-mill at ambient temperature and atmospheric pressure.
Some distinguishing features of this approach when compared with methods commonly employed for mineral CO2 sequestration [3-7] are:
- Use of abundant (land filled) industrial by-products as the primary minerals in the process.
- Value-added use of carbon dioxide as a raw material.
- Low-cost, energy-efficient and sustainable method of processing via simple milling action at ambient temperature and atmospheric pressure.
- High value of the end product as a hydraulic binder for hazardous waste stabilization and also use construction applications.
- A two-step process of transforming carbon dioxide into a stable crystalline carbonate, where the carbonate formed initially is not crystalline but transforms into a crystalline carbonate in the course of hydration of the cementitious binder.
Achievement of high CO2 uptake was an important objective of this project. For this purpose, the raw material blend was modified by the addition of supplementary materials that have been found to raise CO2 uptake in the conventional methods of mineral sequestration. These additives, and their mechanisms of action are:
- Sodium bicarbonate for catalytic increase of the rate and extent of carbonation [8].
- Silicate-based additives for increasing the rate and extent of carbon dioxide sorption [9].
- Magnesium (and calcium) silicate for facilitating intense penetration and sorption of carbon dioxide [10].
- Calcium (and magnesium) oxide for enhancing carbonation reactions.
- Amine compounds for catalytic action as CO2 sorbents [11-13].
- Acetic (and other weak) acids for catalytic enhancement of the extraction of calcium ions [14-19], with subsequent use of sodium hydroxide to promote carbonation reactions.
- Polyethylenimine for initial adsorption of significant quantities of CO2 [13,20].
A number of these additives were evaluated for facilitating and intensifying the solid-state (mechanochemical) carbonation reactions.
Materials and Methods
Materials
The base formulation comprised land filled coal fly ash, ground granulated blast furnace slag, quick lime and sodium hydroxide. Land filled coal fly ash was obtained from a landfill in Detroit (Michigan) that stores the coal fly ash produced in a DTE Energy power plant. This ash had been disposed of in landfill for two years. Median particle sizes of this landfill coal fly ash are 14m. The chemical composition of the ash obtained via x-ray fluorescence spectroscopy, includes: CaO (26.72 wt.%), Fe2O3 (21.82 wt.%), SiO2 (19.99 wt.%), Al2O3 (8.89 wt.%), SO3 (5.31 wt.%), (MgO (2.7 wt.%) Na2O (2.7 wt.%), BaO (2.14 wt.%), TiO2 (2.63 wt.%), K2O (2.4 wt.%), SrO (1.64 wt.%) and P2O5 (1.37 wt.%).
Granulated blast furnace slag (GBFS) was supplied by Lafarge. The chemical composition of GGBF slag, obtained via x-ray fluorescence spectroscopy, includes: CaO (40.8 wt.%), SiO2 (37.5 wt.%), Al2O3 (9.54 wt.%), MgO (10.9 wt.%) Fe2O3 (0.47 wt.%), Na2O (0.25 wt.%) and K2O (0.45 wt.%). Quick lime (calcium oxide) in powder foam was purchased from Sigma- Aldrich that meet analytical specification of FCC with puriss grade and density of 3.3 g/mL. Sodium hydroxide was purchased from Red Hot Devil Eye.
The base formulation was modified through the addition of various additives with the objective of achieving improvements in terms of the end product strength, CO2 uptake, and immobilization of heavy metals. Figure 1 shows pictures of these additives that are described in the following. They were used in as-received form, except for basalt that was ground. Dunite (a cumulate of olivine and minor crhomit [21]) was purchased from Persson minerals. The chemical composition of Dunite includes MgO, SiO2, Fe2O3, CaO and Al2O3. Olivine, which is the primary constituent of Dunite, comprises largely of Mg2SiO4 and Fe2SiO4.
The base formulation comprised land filled coal fly ash, ground granulated blast furnace slag, quick lime and sodium hydroxide. Land filled coal fly ash was obtained from a landfill in Detroit (Michigan) that stores the coal fly ash produced in a DTE Energy power plant. This ash had been disposed of in landfill for two years. Median particle sizes of this landfill coal fly ash are 14m. The chemical composition of the ash obtained via x-ray fluorescence spectroscopy, includes: CaO (26.72 wt.%), Fe2O3 (21.82 wt.%), SiO2 (19.99 wt.%), Al2O3 (8.89 wt.%), SO3 (5.31 wt.%), (MgO (2.7 wt.%) Na2O (2.7 wt.%), BaO (2.14 wt.%), TiO2 (2.63 wt.%), K2O (2.4 wt.%), SrO (1.64 wt.%) and P2O5 (1.37 wt.%).

Serpentine was supplied by Laurel Mountain Group. It is a subgroup of the Kaolinite-Serpentine group; the composition of serpentine can be expressed as (Mg, Fe, Ni, Mn, Al, Zn)3 (Si2O5)(OH)4, which is a compound of six metal elements. The most common serpentine species contain large amounts of Mg. Basalt was purchased from Fisher Scientific Co LLC. Its chemical composition includes SiO2, Al2O3, CaO, FeO, MgO and TiO2. Basalt is a common extrusive volcanic rock generated from rapid cooling of lava at the surface of earth. Normally, it contains 45% to 55% silica, and its average density is close to 3.0gm/cm3 [22]. Basalt has been categorized to different types depending on its silica, alumina and alkali contents; different levels of activity are exhibited by different types of basalt.
The celestine (or celestite) used in this study was purchased from (ebay). It is a mineral with formula (Sr,Ba)SO4 that isgenerally blue in color with orthorhombic crystalline structure. Pure celestite is colorless, and the blue color is caused by its impurities. Gypsum was purchased from Fisher Scientific Co LLC. Wollastonite, expressed by the simplified chemical formula CaSiO3, was supplied by Fisher Scientific Co LLC. Clinoptilolite was purchased from Fisher Scientific Co LLC. It is a natural zeolite with a microporous arrangement of silica and alumina tetrahedra. It has the complex formula (Na,K,Ca)2- 3Al3(Al,Si)2Si13O36•12H2O. It forms as white to reddish tabular monoclinic tectosilicate crystals with a Mohs hardness of 3.5 to 4 and a specific gravity of 2.1 to 2.2 [23].
Talc was purchased from Sigma Aldrich. It is a mineral with pale color and waxy luster, with a chemical formula Mg3Si4O10(OH)2, and a hardness of 1. Talc is typically found in metamorphic rocks with abundant carbonate minerals. It is flexible, but not elastic. The carbonation reaction of talc may be expressed as Mg3Si4O10(OH)2 + 3CO2=3MgCO3 + 4SiO2+H2O [24]. Molecular sieve (13X beads -12mesh) was purchased from Sigma Aldrich. It can be expressed by the chemical formula Na2O:1Al2O3 : 2.8±0.2 SiO2 :xH2O.
Experimental method
The CO2uptake induced by mechanochemical processing of landfilled coal ash with supplementary minerals in carbon dioxide environment was estimated as the difference in the loss on ignition (LOI) of similar products milled in CO2and in air. FTIR spectroscopy was also used to better understand the nature of the carbon dioxide incorporated into the hydraulic binder via mechanochemical means. The compressive strength achieved by hydration of hydraulic binders was assessed following the ASTM C109 procedures. Curing was carried out at room temperature, and testing was performed at 7 and 28 days of age.
The solidification/stabilization qualities of the hydraulic binders were assessed by testing of the hydrated binder and also the hydrated binder with mixing water spiked with copper. Testing of the hydrated binder (without spiking) was performed on solid pastes cured at room temperatures until 7 and 28 days of age. The Toxicity Characteristic Leaching Procedure (TCLP) was used for this purpose. This procedure is required for regulatory purposes under the Resource Conservation and Recovery Act (RCRA) Land Disposal Restrictions (LDRs). Metal ion extractions were evaluated using the US EPA TCLP procedure. The heavy metal concentrations of leachates were determined via inductively coupled plasma (ICP) analysis.
The heavy metal immobilization qualities of the hydraulic binder were assessed by spiking the mixing water with copper (30,000ppm). Samples of the resulting pastes were prepared per ASTM C109. After curing for 7 and 28 days at room temperature, toxicity tests were performed per US EPA TCLP procedures; ICP analysis was performed to determine the leachate copper concentration.
Results and Discussion
CO2 uptake
In a preliminary screening process, 10 wt. % of each additive was used with the base formulation comprising 5wt.% CaO and 5wt. % NaOH as the main alkali activators for land filled coal ash (90wt. %). Table 1 presents different formulations studied and their CO2 uptake and the corresponding pH. Additives were milled together with other raw materials over a two-hour period in the presence of carbon dioxide. CO2uptake was estimated as the difference in the loss on ignition (LOI) of the CO2-milled versus air-milled hydraulic cements. The highest CO2 uptake was achieved with the following additives (in decreasing order): Celestine [25], basalt, and GGBF slag. Compressive strength test results, however, indicated that celestine produced the lowest compressive strength. Hence, basalt and slag were investigated further in order to optimize their benefits towards CO2 uptake. Figure 2 presents the measured values of pH in solution and the corresponding CO2 uptake for hydraulic cements produced via mechanochemical processing in the presence of CO2 gas [26]. As previous observations indicated, a high CO2 uptake corresponds with a low alkalinity, which is expected from the incorporation of largely acidic carbonates into the alkali aluminosilicate structure (Figure 3).



Effect of basalt as an additive for increasing CO2 uptake
Basalt was further investigated as an additive in mechanochemical processing in CO2 environment of hydraulic cements formulated with land filled ash. This work was performed with 4wt.% basalt. Moisture was introduced during mechanochemical processing of raw materials in the presence of CO2. Table 2 presents the formulations considered at this stage of investigation, together with the CO2 uptake and the pH in solution of the resultant hydraulic cements. The presence of basalt is observed to raise the CO2uptake by 2.6%, with a corresponding drop in the pH of the cement in solution. The lower dosage of basalt used here produced results which were similar to those obtained with the higher dosage of basalt used in screening tests. Milling in nitrogen was performed to gain further insight into the mechanochemical phenomena that are responsible for the uptake of CO2 and the transformation of raw materials into hydraulic cement. The preliminary results generated in this study indicated that the hydraulic cement processed in nitrogen actually produced a lower pH in solution when compared with that processed in air. Processing in CO2 still produced the lowest pH value. Figure 4 compares the FTIR spectra of the CO2milled versus air-milled hydraulic cements. Significant enhancement of the peak intensity at 1400cm-1 was observed with milling performed in CO2, which points at the strong presence of carbonates. Appearance of a new peak around 2500cm-1 with CO2 milling may also reflect the presence of carbonates. In addition, the two peaks appearing near 1600cm-1 could be due to the formation of carbonate complexes with CO2milling.

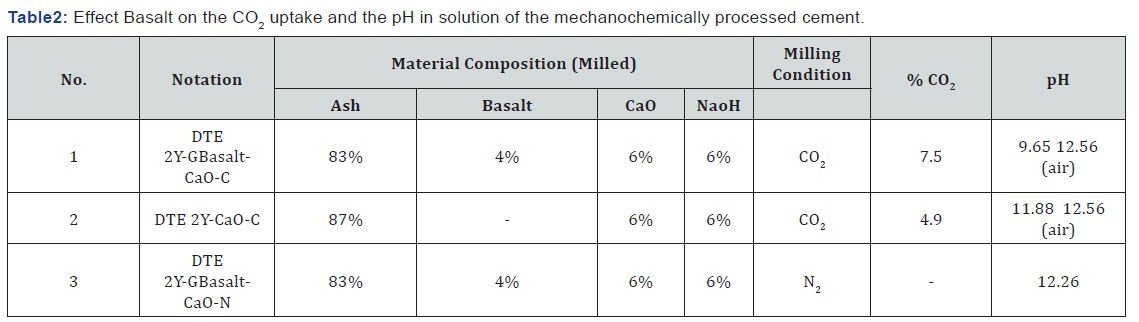
Effect of granulated blast furnace (GBF) slag on CO2 uptake
Table 3 introduces the formulations incorporating GBFS investigated in this work, and the CO2 uptake and pH in solution of the resulting hydraulic cement. The highest CO2 uptake was obtained with 20% slag. The CO2 uptake was estimated based on the difference in LOI between binders processed in CO2 and in air. The formulation DTE 2Y-Slag-CaO-C provided a higher CO2 uptake, but later studies indicated that this hydraulic cement produced a relatively low compressive strength. It was also found that the compressive strength obtained this particular cement could be increased, without lowering its CO2 uptake, by adding a relatively small dosage of sodium silicate.

FTIR spectroscopy was used to gain insight into the mechanism and extent of CO2 uptake for the hydraulic binders formulated with slag. The relative areas under peaks provide an indication of the extent of carbonation Figure 5. Compares the FTIR spectra of slag-based formulations milled in CO2 and in air. Similar to basalt-based hydraulic cements, the slag-based cement exhibited a significant rise in the intensity of a typical carbonate peak at 1400cm-1 after milling in CO2. Appearance of a new peak around 2500cm-1 may also be due to the formation of carbonates. The two new peaks around 1600cm-1 could also reflect the presence of carbonate environments.
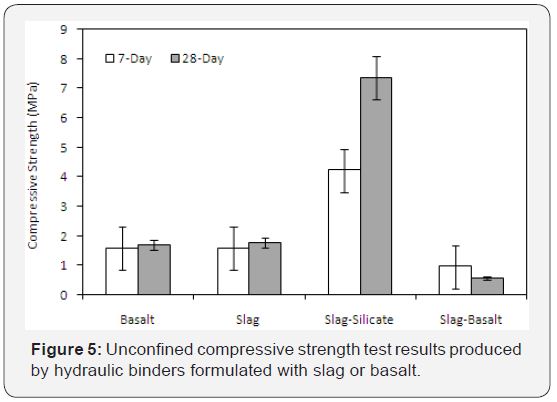
With the addition of 5% sodium silicate to the formulation, compressive strength increased significantly with only a slight drop in CO2uptake. Figure 6 shows the FTIR spectra for cements formulated with Slag-silicate with processing performed in air and in CO2. The area under the pead at 1400cm-1 increased, pointing at a notable CO2 uptake. A double peak around 1600cm- 1 could also be detected for the cement milled in CO2. The FTIR spectrum of the hydrated paste made with the formulation incorporating slag processed in CO2 points at the presence of the carbonate peak around 1400cm-1. The peak near 1064cm1 corresponds to Si-O and Al-O stretching bands. The bands appearing around 720-730cm-1 are associated with aluminumrich structures such as hydroxysodalite. The band at 640-630cm- 1 points at the presence of zeolitic structures. In order to confirm the formation of these zeolitic structures, more detailed studies involving x-ray diffraction spectrometry need to be carried out.

Unconfined compressive strength
Figure 7 shows the compressive strength test data for cement formulations incorporating slag and basalt. Both these formulations showed promising CO2 uptakes, but produced relatively low compressive strengths. This strength loss is probably due to the reduced alkalinity associated with CO2 uptake. Steps were taken to raise strength without compromising the CO2 uptake. Out of different approaches taken, introduction of sodium silicate seemed more promising for formulations incorporating slag. Addition of 5 wt.% sodium silicate increased the compressive strength by a factor of 2.7 at 7 days, and a factor of 4.1 at 28 days of age. Efforts were made to formulate a hybrid cement by combining basalt (4wt.%) and slag (20wt. %); the compressive strength produced by the resultant cement, however, was relatively low.

Hazardous waste solidification/stabilization
The hydraulic cement with slag-silicate formulation (65% DTE -2Y ash, 20% slag, 5% NaOH 5% CaO and 5% sodium silicate), produced via mechanochemical processing in CO2, was subjected to the TCLP procedure. Leachates of the hydrated cement milled in CO2were compared with those produced with the hydrated airmilled cement. Figure presents the heavy metal concentrations in leachates of hydrated pastes of slag-silicate binders processed in air and in CO2. Mechanochemical processing in CO2 (versus in air) produced notable reductions in the presence of hazardous metal cations in leachates. This finding highlights the value added to the hydraulic cement by the captured CO2. Besides reduction of the RCRA heavy metal ions (As, Ba, Cd, Cr, Pb, Se, Ag) in leachates, a reduction in Sr was also observed. Ag and Cd concentrations were below the detection limit of the instrument. The degree of immobilization was calculated for each formulation. The highest copper immobilization>99.9 % was obtained with the formulation comprising 65% land filled ash, 20% Slag, 5% NaOH, 5% CaO and 5% sodium metasilicate (processed in CO2).
Past research on GBFS indicates that alkali activation of GBFS can form zeolitic structures that are particularly effective in immobilization of heavy metals [27,28]. Formation of zeolites in alkali activated slag pastes is strongly dependent on the chemical composition of the slag, and requires a system with relatively high Al/Si ratio and a sufficiently low Ca/Si ratio [29]. The effective solidification/stabilization of heavy metals observed here can be attributed partly to immobilization in zeolite. Besides microstructure, the pH values of pastes also play an important role in the immobilization process. High pH values benefit the immobilization qualities of the paste. In an environment with high pH (>12), heavy metal hydroxides of very low solubility precipitate [30].
Summary and Conclusion
Landfilled coal ash was excavated two years after disposal, and was subjected to mechanochemical processing in the presence of diluted carbon dioxide gas and supplementary minerals. The dilute gas had a CO2 concentration of 20% to simulate combustion emissions. The supplementary minerals were used to introduce additional alkali and alkaline earth metals as well as alumino silicates for realizing a desired chemical balance for enhancing the CO2 uptake and also the quality of the resultant hydraulic cement. The primary application targeted for the hydraulic cement was stabilization/solidification of hazardous wastes. The selection of raw materials, the simple approach to processing and the direct use of combustion emissions (represented by the dilute CO2 gas) were intended to lower the cost and energy content of the process. Different formulations of raw materials were considered, and those offering a desired balance of CO2 uptake and the cement hydrate strength and heavy metal immobilization qualities were identified. Besides minerals supplying alkali/ alkaline earth metals and silicates, which summed up to at about 15% by weight of total raw materials, granulated blast furnace slag at about 20% by weight of raw materials was found to benefit the CO2 uptake and the performance of cement hydrates. Comparisons were made between mechanochemical processing in air versus CO2, which revealed the value added by capture ofCO2 towards achieving enhanced end product qualities (including heave metals immobilization). Carbon dioxide is thus a valuable (gaseous) raw material in production of the hydraulic cement.
Acknowledgment
Landfilled coal ash was excavated two years after disposal, and was subjected to mechanochemical processing in the presence of diluted carbon dioxide gas and supplementary minerals. The dilute gas had a CO2 concentration of 20% to simulate combustion emissions. The supplementary minerals were used to introduce additional alkali and alkaline earth metals as well as alumino silicates for realizing a desired chemical balance for enhancing the CO2 uptake and also the quality of the resultant hydraulic cement. The primary application targeted for the hydraulic cement was stabilization/solidification of hazardous wastes. The selection of raw materials, the simple approach to processing and the direct use of combustion emissions (represented by the dilute CO2 gas) were intended to lower the cost and energy content of the process. Different formulations of raw materials were considered, and those offering a desired balance of CO2 uptake and the cement hydrate strength and heavy metal immobilization qualities were identified. Besides minerals supplying alkali/ alkaline earth metals and silicates, which summed up to at about 15% by weight of total raw materials, granulated blast furnace slag at about 20% by weight of raw materials was found to benefit the CO2 uptake and the performance of cement hydrates. Comparisons were made between mechanochemical processing in air versus CO2, which revealed the value added by capture ofCO2 towards achieving enhanced end product qualities (including heave metals immobilization). Carbon dioxide is thus a valuable (gaseous) raw material in production of the hydraulic cement.
References
- Huijgen WJJ, Comans RNJ (2003) Carbon dioxide sequestration by mineral carbonation. Literature Review. Energy research Centre of the Netherlands.
- Huijgen WJ, Comans R (2007) Carbon dioxide sequestration by mineral carbonation.
- Dermatas D, Meng X (2003) Utilization of fly ash for stabilization/ solidification of heavy metal contaminated soils. Engineering Geology 70(3-4): 377-394.
- Qian G, Cao Y, Chui P, Tay J (2006) Utilization of MSWI fly ash for stabilization/solidification of industrial waste sludge. J Hazard Mater 129(1-3): 274-281.
- Ahmaruzzaman M (2010) A review on the utilization of fly ash. Progress in Energy and Combustion Science 36(3): 327-363.
- Fernández Bertos M, Simons SJ, Hills CD, Carey PJ (2004) A review of accelerated carbonation technology in the treatment of cement-based materials and sequestration of CO2. J Hazard Mater 112(3): 193-205.
- Silverstrim T (1997) Fly ash cementitious material and method of making a product. Google Patents.
- O Connor W (2005) Aqueous mineral carbonation. Final Report, DOE/ ARC-TR-04: 2.
- Olivares-MM, Drage TC, Maroto-VMM (2010) Novel lithium-based sorbents from fly ashes for CO2 capture at high temperatures. International Journal of Greenhouse Gas Control 4(4): 623-629.
- Kalinkin AM, Kalinkina EV, Zalkind OA (2009) Mechanosorption of carbon dioxide by Ca- and Mg- containing silicates and alumosilicates. Sorption of CO2 and structure-related chemical changes. Colloid Journal 71(2): 185-192.
- Gray ML (2004) CO2 capture by amine-enriched fly ash carbon sorbents. Separation and Purification Technology 35(1): 31-36.
- Song K (2012) Precipitation of calcium carbonate during direct aqueous carbonation of flue gas desulfurization gypsum. Chemical Engineering Journal 213: 251-258.
- Yang H (2008) Progress in carbon dioxide separation and capture: A review. Journal of Environmental Sciences 20(1): 14-27.
- Huijgen WJJ, Comans RNJ (2005) Carbon dioxide sequestration by mineral carbonation. Literature review update 2003-2004. Energy research Centre of the Netherlands ECN, Petten, Netherlands.
- Huijgen WJJ, Comans RNJ (2005) Mineral CO2 sequestration by carbonation of industrial residues. The Netherlands: Energy Research Centre of the Netherlands (ECN).
- Huijgen WJJ, Comans RNJ, Witkamp GJ (2006) Mechanisms of aqueous wollastonite carbonation as a possible CO2 sequestration process. Chemical Engineering Science61(13): 4242-4251.
- Sanna A (2012) Waste materials for carbon capture and storage by mineralisation (CCSM)–A UK perspective. Applied Energy 99: 545-554.
- Sano T, Miura S, Furusawa H, Kaneko S, Yoshida T, et al. (2013) Composition of inorganic elements and the leaching behavior of biomass combustion ashes discharged from wood pellet boilers in Japan. Journal of Wood Science 59(4): 307-320.
- Sipilä J, Teir S, Zevenhoven R (2008) Carbon dioxide sequestration by mineral carbonation Literature review update 2005-2007. Report VT 1: 2008.
- Kucęba MI, Nowak I. Development of Fly Ash-Based Sorbent to Capture CO2 from Flue Gas. Springer, pp. 596-602.
- Irvine TN (1974) Petrology of the Duke Island ultramafic complex southeastern Alaska. Geological Society of America Memoirs, 138: 1-244.
- Kuryaeva RG (2004) Effect of High Pressure on the Refractive Index and Density of Natural Aluminosilicate Glasses of Alkali Basalt Composition in the SiO2-Al2O3-TiO2-Fe2O3-P2O5-FeO-MnO-CaO-MgO-Na2O0-K2O System. Glass Physics and Chemistry 30(6): 523-531.
- Sprynskyy M, Buszewski B, Terzyk AP, Namieśnik J (2009) Study of the selection mechanism of heavy metal (Pb2+, Cu2+, Ni2+, and Cd2+) adsorption on clinoptilolite. Journal of colloid and interface science 304(1): 21-28.
- Springer Rk (1974) Contact metamorphosed ultramafic rocks in the western Sierra Nevada foothills, California. Journal of Petrology 15(1): 160-195.
- Dilmore RM (2009) Sequestration of CO2 in mixtures of caustic byproduct and saline waste water. Environmental Engineering Science 26(8): 1325-1333.
- Garrels RM (1983) The carbonate-silicate geochemical cycle and its effect on atmospheric carbon dioxide over the past 100 million years. Am J Sci: 283641-28683.
- Malolepszy JJ Deja (1988) The influence of curing conditions on the mechanical properties of alkali slag binders. Silicates industriels 53(11-12): 179-186.
- Wang SD, Scrivener KL (1995) Hydration products of alkali activated slag cement. Cement and Concrete Research 5(3): 561-571.
- Wang SD (2000) The role of sodium during the hydration of alkaliactivated slag. Advances in cement research 12(2): 65-69.
- Deja J (2002) Immobilization of Cr6+, Cd2+, Zn2+ and Pb2+ in alkaliactivated slag binders. Cement and Concrete Research 32(12): 1971- 1979.