Electrical Storm in Patients with Inappropriate Implantable Cardioverter-Defibrillator Therapy: Current Trends in Clinical Practice between Guidelines and Technology Progress
Maurizio Santomauro1*, Mario Petretta2, Carla Riganti3, Mario Alberto Santomauro1, Giovanni D’Angelo2, Alessandra Cuomo2, Francesco Barillà4, Gabriele Iannelli1 and Domenico Bonaduce2
1Department of Cardiovascular Emergency, Internal Medicine and Geriatric, Medical School, Federico II University of Naples, Italy
2Department of Translational Medical Sciences, Federico II University of Naples, Italy
3General Direction, Medical School, Federico II University of Naples, Italy
4Department of Cardiology, Sapienza University of Rome, Italy
Submission: February 18, 2020; Published: February 25, 2020
*Corresponding author: Maurizio Santomauro, Department of Cardiovascular Emergency, Internal Medicine, Geriatric Medical School, Federico II University, Via Sergio Pansini 5- 80131 Naples, Italy
How to cite this article:Santomauro M, Petretta M, Riganti C, Santomauro MA, D’Angelo G, Cuomo A, Barillà F, Iannelli G and Bonaduce D. Electrical Storm in Patients with Inappropriate Implantable Cardioverter-Defibrillator Therapy: Current Trends in Clinical Practice between Guidelines and Technology Progress. J Cardiol & Cardiovasc Ther. 2020; 15(5): 555925. DOI: 10.19080/JOCCT.2020.15.555925
Abstract
The term “Electrical Storm” (ES) indicates cardiac electrical instability manifested by several episodes of ventricular tachyarrhythmias within a short time. In the contemporary implantable cardioverter-defibrillator (ICD) era, ES is defined as recurrent (three or more separate episodes) of ICD discharges over 24 hours. Some ESs are due to inappropriate repetitive shock and are potentially lethal for the patient. The most common causes of inappropriate ICD shock include supraventricular tachycardia with high ventricular response and oversening of peaked T waves or R wave, myopotentials or electrical noise. Appropriate diagnosis and treatment are critical in the Emergency Department. To approach these patients systematically, it is important to understand, that there are four causes of shock. Modern ICD incorporate sophisticated tachycardia detection algorithms within their programming designed to minimize devices’ detection mistakes and ICD-related information can also be checked using remote home monitoring systems. However, these opportunities are often not utilized to their full benefit. Thus, careful attention should be paid to the programming of the device. Fine tuning of the detection and differentiation algorithms is critical, and best done by a practitioner who understands the subtle differences among the different manufacturers. The racommendations for those problems should be revised.
Keywords: Implantable cardioverter defibrillator; Electrical Storm; Inappropriate shock
Abbreviations: ES: Electrical Storm; VT: Ventricular Tachycardia; VF: Ventricular Fibrillation; ATP: Antitachycardia Pacing; SCD: Sudden Cardiac Death; HF: Heart Failure; SVA: Supraventricular Arrhythmias; CRT-D: Cardiac Resynchronization Therapy; AF: Atrial Fibrillation; SVT: Supraventricular Tachycardia
Background
Current definition of Electrical Storm (ES) is the occurrence of three or more episodes of sustained ventricular tachycardia (VT) or ventricular fibrillation (VF) within 24 h requiring appropriate medical intervention. The same definition applies in ICD carriers in which ES is defined by three or more appropriate and separate (at least 5 min) device interventions in 24 hours, either with antitachycardia pacing (ATP) or shock [1-4]. Current guide lines recommend ICD implantation for secondary prevention of sudden cardiac death (SCD) in patients with documented VF or haemodynamically not tolerated VT in the absence of reversible causes or within 48 h after myocardial infarction who are receiving chronic optimal medical therapy and have a reasonable expectation of survival with a good functional status >1 year [5]. They also recommend ICD implantation for primary prevention in patients with ischemic or non-ischemic dilated cardiomyopathy and ejection fraction equal or lower than 35 % after at least 3 months of optimized medical therapy and in other less frequent inherited arrhythmogenic syndromes. For these reasons, due to the increasing number of ICD implantations, ES is an increasingly frequent cause of access to emergency department (ED). It is estimated that about 25 % of ICD carriers for secondary prevention of SCD experience at least one ES episode per year of follow-up [6-8]. Sometimes multiple recurrent ICD discharges are not associated with ES but are due to device malfunctioning. The most common causes of inappropriate ICD shock include supraventricular tachycardia with high ventricular response, device oversensing and mechanical malfunctions. Recurrent ICD shocks can cause myocardial damage by cell injury due to direct electrocution, and by activation of heart failure (HF) molecular signaling pathways, above all the adrenergic neurohormonal system. Adrenergic iperactivity may then synergize with recurrent ventricular arrhythmias in exacerbating ventricular dysfunction and worsening HF. Sweeney et al. [9] demonstrated that electrical shocks were associated with an increased risk of death independently from underlying ventricular arrhythmia. Authors esteemes that for every delivered shock, whether appropriate or not, the risk of death increases by 20%. On the other hand, no increased risk was associated with antitachycardia pacing (ATP) therapies. ES does not only cause myocardial damage, but can deplete a full device battery within hours, potentially leaving the patient unprotected from life-threatening arrhythmic events [10-12]. ES should be treated by immediate intervention to suppress ICD shocks. Moreover, inappropriate discharges from ICD should be avoided at all cost by an optimal device programming [13].
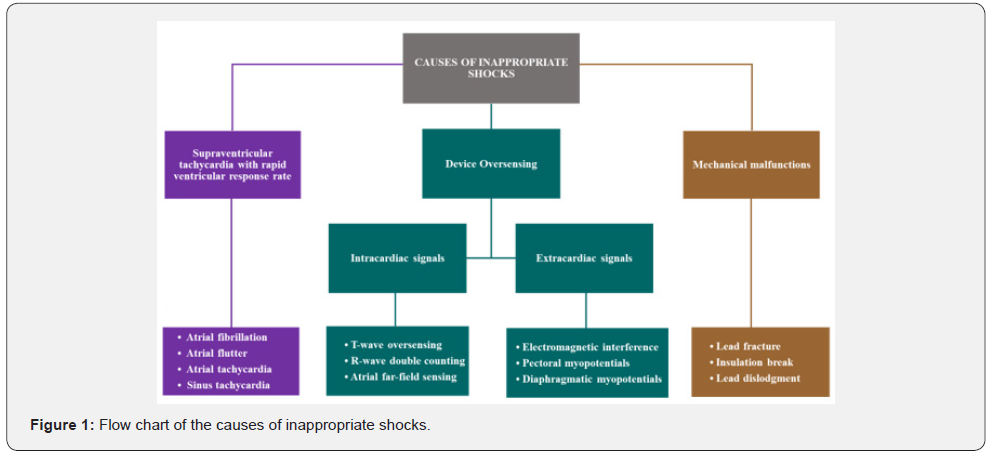
Implantable Device
The ICD is a implantable device able to monitor cardiac rhythm and terminate potentially life-threatening arrhythmias. It consists of two main components: the generator, that contains the battery, all the circuits that run the device, and the operator communicating system; the leads, that reach heart chambers through the venous system, allowing the device to monitor heart electrical activity and to deliver therapies. The ICD has a lead implanted in the right ventricle apex able to record ventricular activity and release therapies like pacing and/or direct current shock. Moreover, some ICD have another lead implanted in the right atrium to record atrial electrical activity, improving discrimination between supraventricular arrhythmias (SVA) and ventricular arrhythmias and to pace the atrium (ICD-DR). ICD with cardiac resynchronization therapy (CRT-D) have a third lead that paces the left ventricle (through the coronary venous system) synchronously to the right ventricle improving contractility. ICD use mathematical algorithms defined by the manufacturer to discriminate life-threatening ventricular arrhythmias from supraventricular arrhythmias and to deliver appropriate therapy. Modern ICD store information from various diagnostic features including intracardial ECG registrations during arrhythmia and can transmit these data using remote monitoring technology. Furthermore, ICD can generate audible alarms in the case of device malfunction, low battery capacity and lead failure. Sometimes correct recognition fails, and, in this case, the therapy delivered is defined inappropriate. VT recognition is primarily based upon tachycardia cycle length and duration. Both of these parameters are tailored on the patient’s characteristics. Thus, ICD use ventricular rate zones for rhythm classification. The boundaries between zones are defined by two main principles: the recognition of unstable fast VT/VF must be highly sensitive even at the cost of inappropriate rapid SVA treat ment; the recognition of slower VT has to be more specific to avoid inappropriate therapies even if it leads to delay in detection. The ICD treats ventricular tachyarrhythmias with two modalities: antitachycardia pacing (ATP) and direct current shock. ATP is a brief ventricular pacing (6-8 beats) with a cycle length slightly lower (thus at a faster rate) compared to the one of the arrhythmia, in the attempt of resetting the reentrant circuit and of interrupting the arrhythmia; sometimes the paced cycle shortens from beat to beat and in this case it is referred as ATP ramp. Direct current shock is a biphasic electrical shock provided between the generator case and the coil localized on the right ventricular lead; the energy released may vary, reaching up to 41 J, with the latest generation high-energy devices. Basing on several studies [14-21], ICD programming should empirically involve the use of three rate zones: a slow VT zone up to 320 ms cycle length (<188 bpm); a fast VT zone from 320 to 240 ms (188–250 bpm); a VF zone from 240 ms (>250 bpm). In VT zones a variable number of ATP attempts precede the shocks delivery. In the slow VT zone, a greater number of ATP attempts are usually programmed compared to fast VT zone, since fast arrhythmias are usually less tolerated. In the VF zone, the hemodynamic instability of the arrhythmia and its high life-threatening potential require an immediate shock delivery. In modern devices an ATP is delivered during capacitor charging, avoiding the shock in the case of interruption of arrhythmia. VT/VF detection is not only based on ventricular rate but also requires a defined duration of the arrhythmic event, to avoid detection of non-sustained episodes. Usually a VT/VF is detected when a certain percentage of ventricular sensed beats meets cycle length criteria. The type of counting used varies between detection zones and between manufacturers. In order to improve sensibility, according to some manufacturers, the arrhythmia is detected when a certain percentage of beats falls in VF zone, while consecutive interval counting is required in the VT zone to increase specificity. The time of detection in the VT zone should be longer enough to allow spontaneous termination of non-sustained episodes. Figure 1 shows the causes of inappropriate shocks.
Implantable Device
The ICD is a implantable device able to monitor cardiac rhythm and terminate potentially life-threatening arrhythmias. It consists of two main components: the generator, that contains the battery, all the circuits that run the device, and the operator communicating system; the leads, that reach heart chambers through the venous system, allowing the device to monitor heart electrical activity and to deliver therapies. The ICD has a lead implanted in the right ventricle apex able to record ventricular activity and release therapies like pacing and/or direct current shock. Moreover, some ICD have another lead implanted in the right atrium to record atrial electrical activity, improving discrimination between supraventricular arrhythmias (SVA) and ventricular arrhythmias and to pace the atrium (ICD-DR). ICD with cardiac resynchronization therapy (CRT-D) have a third lead that paces the left ventricle (through the coronary venous system) synchronously to the right ventricle improving contractility. ICD use mathematical algorithms defined by the manufacturer to discriminate life-threatening ventricular arrhythmias from supraventricular arrhythmias and to deliver appropriate therapy. Modern ICD store information from various diagnostic features including intracardial ECG registrations during arrhythmia and can transmit these data using remote monitoring technology. Furthermore, ICD can generate audible alarms in the case of device malfunction, low battery capacity and lead failure. Sometimes correct recognition fails, and, in this case, the therapy delivered is defined inappropriate. VT recognition is primarily based upon tachycardia cycle length and duration. Both of these parameters are tailored on the patient’s characteristics. Thus, ICD use ventricular rate zones for rhythm classification. The boundaries between zones are defined by two main principles: the recognition of unstable fast VT/VF must be highly sensitive even at the cost of inappropriate rapid SVA treat ment; the recognition of slower VT has to be more specific to avoid inappropriate therapies even if it leads to delay in detection. The ICD treats ventricular tachyarrhythmias with two modalities: antitachycardia pacing (ATP) and direct current shock. ATP is a brief ventricular pacing (6-8 beats) with a cycle length slightly lower (thus at a faster rate) compared to the one of the arrhythmia, in the attempt of resetting the reentrant circuit and of interrupting the arrhythmia; sometimes the paced cycle shortens from beat to beat and in this case it is referred as ATP ramp. Direct current shock is a biphasic electrical shock provided between the generator case and the coil localized on the right ventricular lead; the energy released may vary, reaching up to 41 J, with the latest generation high-energy devices. Basing on several studies [14-21], ICD programming should empirically involve the use of three rate zones: a slow VT zone up to 320 ms cycle length (<188 bpm); a fast VT zone from 320 to 240 ms (188–250 bpm); a VF zone from 240 ms (>250 bpm). In VT zones a variable number of ATP attempts precede the shocks delivery. In the slow VT zone, a greater number of ATP attempts are usually programmed compared to fast VT zone, since fast arrhythmias are usually less tolerated. In the VF zone, the hemodynamic instability of the arrhythmia and its high life-threatening potential require an immediate shock delivery. In modern devices an ATP is delivered during capacitor charging, avoiding the shock in the case of interruption of arrhythmia. VT/VF detection is not only based on ventricular rate but also requires a defined duration of the arrhythmic event, to avoid detection of non-sustained episodes. Usually a VT/VF is detected when a certain percentage of ventricular sensed beats meets cycle length criteria. The type of counting used varies between detection zones and between manufacturers. In order to improve sensibility, according to some manufacturers, the arrhythmia is detected when a certain percentage of beats falls in VF zone, while consecutive interval counting is required in the VT zone to increase specificity. The time of detection in the VT zone should be longer enough to allow spontaneous termination of non-sustained episodes. Figure 1 shows the causes of inappropriate shocks.
Inappropriate therapies due to Supraventricular tachycardia
One of the main causes of inappropriate shock are failure in discriminating SVA [21-28]. Frequently SVA are associated with a fast-ventricular response that leads ventricular rate to fall into VT/VF detection zone causing inappropriate therapy release. This problem occurs more frequently with single-chamber ICD that does note have atrial sensing capabilities. Current guidelines do not provide a clear stepwise approach to managing patients at high risk for recurrent shock. For this reason, appropriate diagnosis and treatment are critical. Modern ICD incorporates sophisticated tachycardia detection algorithms within their programming designed to minimize detection mistakes (Table 1) [29-33]. Thus, attention should be paid to the programming of the device. Fine tuning of the detection and differentiation algorithms are critical and best done by a practitioner who understands the subtle differences among the different manufacturers. Placing an atrial pacing lead and upgrading a single-chamber system to a dual-chamber system for improved SVT discrimination is sometimes necessary and points out the importance of a carefull screening for history of SVT prior to initial ICD implant. ICD uses a variety of algorithms to discriminate SVA from VT. The major ones are listed below: -Atrio ventricular rate comparison applies only in dual-chamber ICDs; when the ventricular rate is faster, the diagnosis is VT. When atrial and ventricular rates are equal, additional criteria are required for discrimination. -Onset: useful for discrimination of gradually accelerating sinus tachycardia from sudden-onset VT; it applies when the RR interval shortens by a programmed percentage if compared to the average number of preceding beats. May fail in case of VT occurring during sinus tachycardia. -Stability: useful to discriminate fast response atrial fibrillation (AF). when RR interval variability is greater than a programmed percentage, AF is suspected. It may fail in the case of very fast AF in which there is a pseudo-regularization of ventricular rate, of atrial flutter or of irregular VT. -Morphology: it compares endocavitary electrocardiograms recorded during sinus rhythm and during VT. It is useful in single-chamber ICD lacking atrial information but may fail in case of intraventricular conduction delays and of rate-dependent conduction delays. -Rate duration: it is an extreme lifesaving measure. It consists in shock delivery after a programmable time interval even if the episode is classified as SVA; this algorithm is usually activated when there is a high risk of undertreatment of VT erroneously recognized as SVA, but it increases the risk of overtreatment.
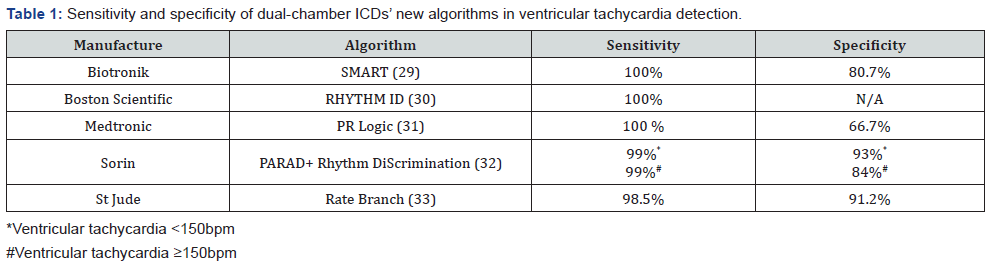
Inappropriate Shock due to Oversensing
Signal misinterpretation is another big important cause of inappropriate shocks. Inappropriate shock may be caused by misdiagnosis of supraventricular tachycardia (SVT) as VT/VF or inappropriate sensing originating from internal and external sources [34-44]. The major intracardiac are listed below: T wave oversensing: it happens when a high amplitude T wave is erroneously recognized as an R wave. It can be due to low ventricular sensing threshold necessary to recognize low-amplitude VF. This problem can be solved increasing sensing threshold, lengthening refractory period or changing sensing decay parameters to suppress T wave detection. Double-counted R waves: it may occur as a result of local ventricular delay in the baseline state or conduction delay caused by drugs or electrolyte abnormalities. It may also occur in patients with a double or triple lead ICD, long PR interval and loss of RV pacing capture. The ICD may count both the paced ventricular event and the spontaneous R wave conducted from the atrium. Finally, another common cause of double counting is loss of RV capture in CRT-ICD: the device counts both the paced ventricular event and the RV depolarization originated from the LV lead. R-wave double counting results in alternation of 2 ventricular cycle lengths. The second component of the R wave is usually sensed as soon as the blanking period terminates and is always classified in the VF zone. The classification of the first one depends on the programming of the tachy-zones and on the heart rate. The double counting can manifest during sinus rhythm, only during precocious ventricular complex (PVC) or during slow VT misclassified as VF, being overestimated the actual heart rate and possibly leading to shocks. Prolongation of the ventricular blanking period from the nominal value corrects ventricular double counting in the majority of cases and must be proposed as the first step if possible, keeping in mind that a common concern is true VF undersensing when the blanking period is over-extended. Similarly, the decrease of the programmed ventricular sensitivity may resolve the problem in a certain number of cases, but this option requires that reliable sensing of VF is confirmed at the reduced level of sensitivity. Moreover, lowering ventricular sensitivity may be dangerous and useless since the amplitude of the 2 signals may be too high. Furthermore, programming of very high VF zone seems to be inappropriate to solve the problem. Atrial far-field sensing: generated by inappropriate detecting of an atrial paced event in the ventricular chamber related to the sensing of events from one chamber in another chamber. Cross-chamber blanking periods are an integral part of the ICD and CRT-D sensing systems. They are used to suppress detection of device-generated artifact as well as certain intrinsic signal artifacts. Events occurring during refractory and cross-chamber blanking periods are ignored for the purposes of pacing timing cycles and ventricular tachycardia detection. Each refractory and fixed cross-chamber blanking period includes a re-triggerable noise window, which helps to detect and classify persistent noise. Cross-chamber blanking periods are designed to promote appropriate sensing of chamber events and prevent oversensing of activity in another chamber. Cross-chamber blanking periods are initiated by paced and/or sensed events in an adjacent chamber. Residual energy on the defibrillation lead after shock delivery can increase the likelihood of crosstalk / farfield sensing. As this residual energy dissipates over time after shock delivery, the potential for crosstalk / far-field sensing also decreases. To reduce oversensing after shock delivery, a longer fixed value is automatically applied for all cross-blanking periods during the Post-Therapy Period. The extracardiac signals are listed below: Electromagnetic interference (EMI): is fortunately fairly infrequent with bipolar leads, but can still occur [45-50]. There are many causes of EMI, the most common include magnetic resonance imaging (MRI), large magnetic fields, arc welding, improper copper wiring in a shower, carrying stereo speakers, working on a running car engine, and lingering in a store’s surveillance gating. To diagnose the cause of shock from EMI often involves a certain amount of detective work. Once the cause is identified, the patient must avoid the culprit, or in some cases, the device can be reprogrammed to prevent recognition of the EMI. Pectoral Myopotentials: farfield myopotential recording may lead to inappropriate arrhythmic detection. In the past, with unipolar leads using large sensing fields this problem was more frequent, but now is largely avoided thanks to modern bipolar leads, recording only more localized signals. These high-frequency, variable amplitude signals are prominent on electrograms that include the ICD can, including shock electrograms and leadless ECG. They may be reproduced by pectoral muscle exercise. However, since ICD do not use these signals as primary sensing channels, pectoral myopotentials do not cause oversensing if the lead is intact. However, they may cause misclassification of exercise-induced sinus tachycardia in VT because algorithms that discriminate VT from SVT based on ventricular electrogram morphology use the RV coil-can vector as the default signal. Pectoral myopotentials might also interfere with algorithms that evaluate lead integrity by comparing near-field and far-field signals. Diaphragmatic Myopotentials: these low-amplitude, high-frequency signals are more prominent on the sensing electrogram than the shock electrogram because the sensing bipole is closer to the source. Their amplitude varies with respiration, but not with cardiac cycle. Oversensing is most common in integrated bipolar sensing at the RV apex and rare in dedicated bipolar sensing or leads in the RV outflow tract. It occurs when sensitivity is maximal, after long diastolic intervals or ventricular paced events, and often ends with a sensed R wave, which reduces sensitivity abruptly. Thus, it commonly occurs in pacemaker-dependent patients, in whom inhibition of pacing maintains high ventricular sensitivity, resulting in persistent oversensing and inappropriate detection of VF. It may present as syncope because of pacing inhibition followed by an inappropriate shock. With chronically implanted leads, oversensing may first occur after the dominant rhythm changes from ventricular sensed to ventricular paced, such as upgrade to CRT-ICD or AV junction ablation. Oversensing may be reproduced by monitoring real-time electrograms during deep breathing or straining in different positions, after programming VF detection off. The mechanical malfunctions are Lead failure. It can have many different causes, but among it is important to include fractured leads, dislodged leads, loss of capture after ICD shock, redundant loops of endocardial leads, chatter in active fixation lead, loose set screew or adapter. Management of this category of inappropriate shock involves fixing the implanted system, either with device reprogramming or reoperation [51- 56]. In these case lead extraction and/or new lead insertion is the only choice. Moreover, modern devices usually provide alerts for lead integrity. The patient should be questioned about positional muscle twitching suggestive of possible lead malfunction. If present, or if nonphysiologic noise is seen on the interrogation strips, active manipulation of the arm and device pocket should be performed while recording a rhythm strip with device channel markers through the interrogation box to determine if it is reproducible.
Device programming to avoid unnecessary therapies
In some patients ES are treated only with ATP, which may remain unnoticed by the patients and be found due to the remote monitoring or during a routine follow-up in a cardiology clinic. Unnecessary therapies are described as therapies delivered within a very short period of time from VT/VF onset, therefore, preventing non-sustained VT/VF from self-terminating. For these reasons, optimization of ICD programming in order to avoid unnecessary shocks is mandatory in patients experiencing ES due to inapproprites shock therapy. As stated above, arrhythmic detection and treatment by ICD is a step process including several variables such as heart rate threshold, number of intervals to detect, discrimination of SVA, and type and number of therapies released. Each of these steps can be tailored upon patient characteristics to avoid unnecessary treatment. A patient who receives multiple shocks is difficult to identify by ispecting data stored in the ICD. These patients usually go to ED with the specific complaint that their ICD has fired several times. At that point, it is critical to define the etiology of the shocks (Figure 2). First step should be performing an initial evaluation as above. The device needs to be fully interrogated, with careful analysis of all of the stored EGM recorded from the recent therapies and specific troubleshooting must be performed as well (Figure 3). The single most important diagnostic test to be performed is the interrogation of the patient’s device. If device malfunction is suspected, therapy (antitachycardia pacing and shock) can be immediately suspended by placing a magnet over the ICD can (Figure 4). Unlike pacemakers, this will not alter the device’s pacing capabilities. Furthermore, if subsequently true ventricular arrhythmiais diagnosed, removing the magnet will immediately reactivate all device functions. Subsequent treatment will depend on the underlying cause. Fortunately, device safety alerts are available, and they are more common in ICD than pacemakers. However, prophylactic removal or replacement of a generator or lead on alert is generally not recommended unless the patient is pacer dependent. All device manufactures with products on alert published management guidelines available for physicians, which should be updated as new data are collected. However, in some patients intracardiac shock does not interrupt VF and multiple sequential shocks are needed. Modern ICD can erogate shocks up to 41 Joule. In these cases, some authors suggested [57-59] the use of a second defibrillator with separate pairs of electrodes pads allowing 400 Joules of biphasic energy or 720 Joules of monophasic energy to depolarize a critical amount of myocardium (Figure 5 & Figure 6). High energy defibrillation is performed by attaching a second set of electrode pads attached from a second defibrillator, ensuring a second vector established through the heart (Figure 6). At the time of defibrillation, both shock buttons from the two different defibrillators are depressed as near-simultaneously as possible. There is not sufficient data to make any widespread recommendation, but the idea of double sequential defibrillation may be another tool in limited ACLS bags as a trick for patients who simply cannot come out of VF. The EMS experiences apply two sets of defibrillator pads to the patient; one in traditional sternum/apex configuration and the other in anterior/ posterior configuration [57-60]. On the contrary, in patients with ICD the two different sets of electrode pads should both be placed in anterior/posterior position, in order to avoid device damages (Figure 6). The first time that double sequential defibrillation was mentioned in human cardiology literature was made by some electrophysiology teams [58-60]. The Authors theorized that double- sequential shocks could reduce the VF threshold, overriding the relative refractory period of cardiac muscle, or possibly could decrease transthoracic impedance, leading to a more effective electrical delivery. They also believe that the change in vectors played an important role, using the first shock to set up for the subsequent shock. However, current advanced cardiac life support (ACLS) guidelines do not report dual-dose technique. Infact, American Heart Association and European Resuscitation Council ACLS guidelines [61,62] recommend administering a maximum of 200 Joule for biphasic (truncated exponential or rectilinear) waveform defibrillators or 360 Joule for monophasic ones. On the other hand, for the purposes of defibrillation, a 2015 ACLS guideline update recognize a knowledge gap regarding optimal waveform use, paddles or electrode pads placement, and ideal dose of subsequent and maximum energy delivery. Indeed, hospitals and EMS agencies should develop guidelines to define appropriate use of dual-dose shocks and to provide reliable subsequent device function in the context of manufacturer-specific warranty practice, considering that the true efficacy of dual-dose defibrillation has yet to be demonstrated with high-quality evidence and it still remains an offlabel use. One possibility could be creating a protocol for routine device testing to evaluate device functionality after each off-label use. Neverthless, high energy shocks increase the likelihood of successful defibrillation, but, on the other hand, the severity of postresuscitation myocardial dysfunction increases with the magnitude of electrical energy delivered by the shock. Recently it has been investigated whether it is possible to predict the occurence of ES based on daily stored ICD summaries [63,64]. For this purpose, Shakibfar used a machine learning approach on a large dataset [65]. The Authors used daily ICD summaries from 19.935 patients to construct and evaluate two models, logistic regression and random forest for predicting the short-term risk of ES. The models were validated on the parts of the data not used for model development. A total of 2.367 ES events occurred in 1.410 patients during an average monitoring period of 1206 days/patient. Random forest performed significantly better than logistic regression, achieving a test accuracy of 0.96 and an area under the curve of 0.80. The most important variables for prediction were the percentage of ventricular pacing and reduced daytime activity, variables known to be indicators of deteriorating heart disease. Thus, machine learning prediction could potentially initiate an alert on the remote monitoring system and prompt the clinician to take action to prevent ES.
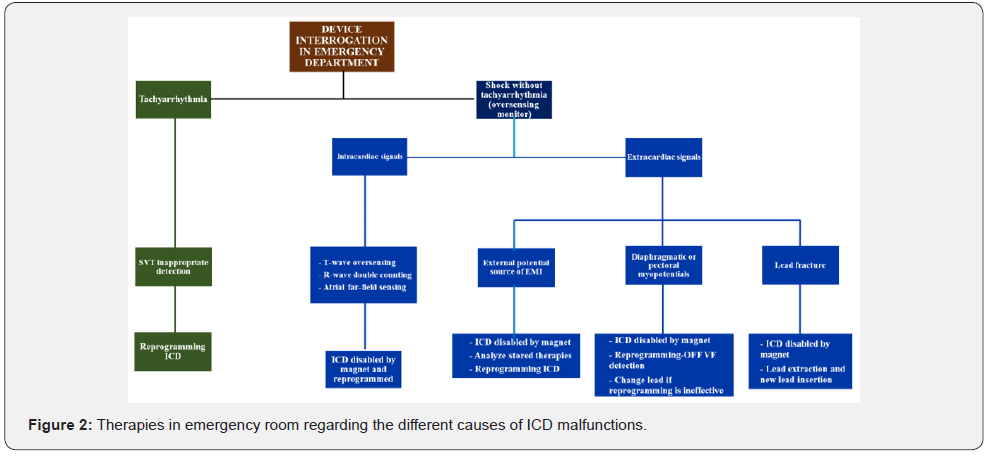
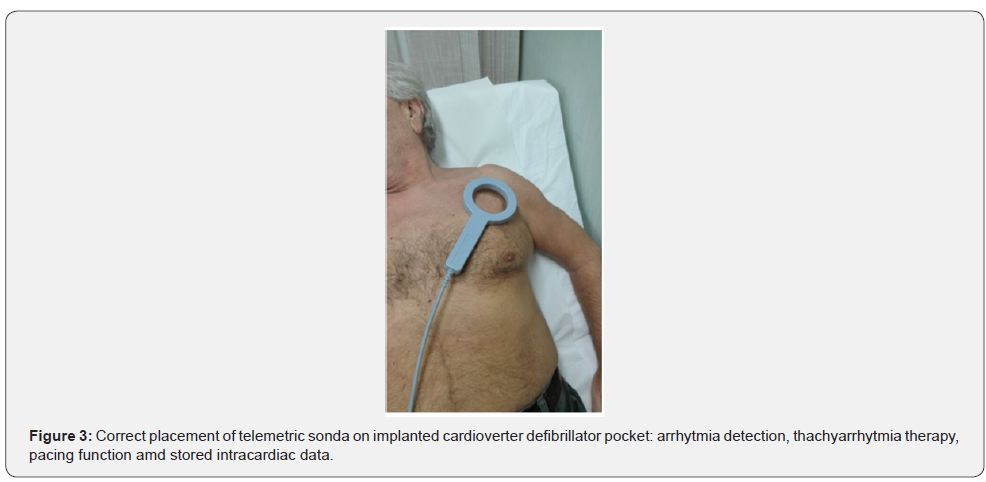
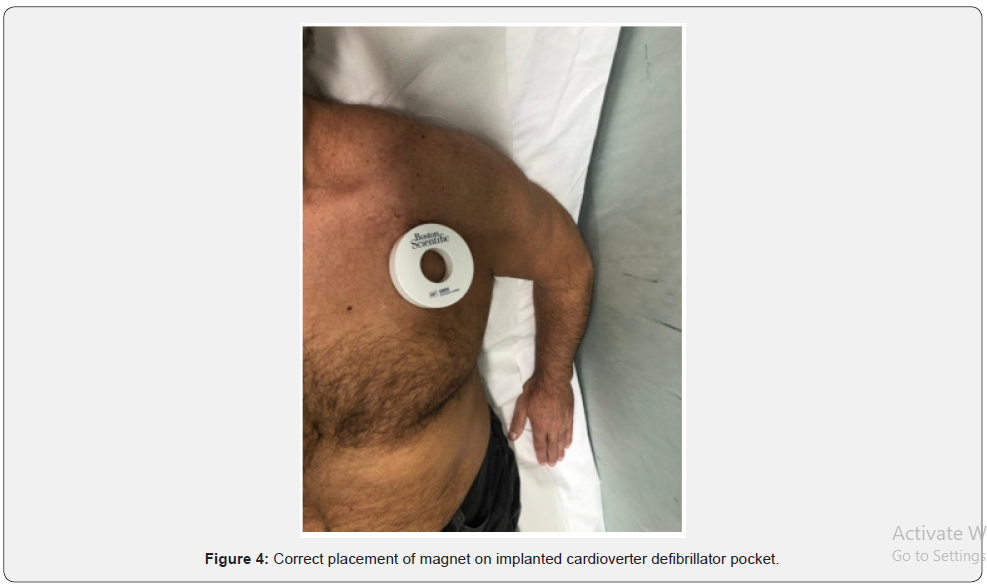

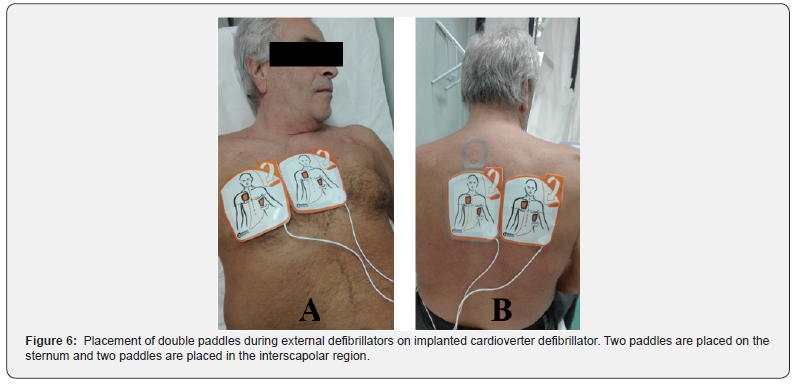
Conclusion
Inappropriate therapies (especially shocks) are one of the main issues to be avoided because they cause patient discomfort, are potentially proarrhythmic and reduce ICD battery life. The two main causes of ES caused by inappropriate shock therapy are failure in discriminating SVA and signal misinterpretation. ES are a life-threatening syndrome and the appropriateness of acute management determines the patient’s survival. Despite the difficulties associated with a comprehensive evaluation of this critical condition, a diagnostic approach based on the type of arrhythmia and the signals of device malfunction facilitates the mechanism-directed of inappropriate shocks. Recent advances in ICD reprogramming algorithm have greatly improved the clinical outcomes.
Conflict of Interest
The author has no conflict of interest to disclose.
Acknowledgments
The authors thank the engineers Luigi Campanile and Francesco Ricca (Boston Team) for their excellent technical support.
References
- Sagone A (2015) Electrical Storm: Incidence, Prognosis and Therapy. J Atr Fibrillation 8(4): 1150.
- Hohnloser SH, Al-Khalidi HR, Pratt CM, Brum JM, Tatla DS, et al (2006) Electrical storm in patients with an implantable defibrillator: incidence, features, and preventive therapy: insights from a randomized trial. Eur Heart J 27(24): 3027-3032.
- Israel CW, Barold SS (2007) Electrical storm in patients with an implanted defibrillator: a matter of definition Ann Noninvasive Electrocardiol 12(4): 375-382.
- Pedersen CT, Kay GN, Kalman J, Borggrefe M, Della-Bella P, et al. (2014) EHRA/HRS/APHRS expert consensus on ventricular arrhythmias. Heart Rhythm 11(10): e166-e196.
- McMurray JJV, Adamopoulos S, Anker SD, Auricchio A, Böhm M, et al (2012) ESC Guidelines for the diagnosis and treatment of acute and chronic heart failure 2012: The Task Force for the Diagnosis and Treatment of Acute and Chronic Heart Failure 2012 of the European Society of Cardiology. Developed in collaboration with the the Heart Failure Association (HFA) of ESC. Eur Heart J 33(14): 1787-1847.
- Priori SG, Blomström‐Lundqvist C, Mazzanti A, Blom N, Borggrefe M, et al. (2015) 2015 ESC Guidelines for the management of patients with ventricular arrhythmias and the prevention of sudden cardiac death: the Task Force for the Management of Patients with Ventricular Arrhythmias and the Prevention of Sudden Cardiac Death of the European Society of Cardiology (ESC) Endorsed by: association for European Paediatric and Congenital Cardiology (AEPC). Eur Heart J36(41): 2793-2867.
- Jimenez-Candil J, Hernandez J, Martin A, Moríñigo J, Perdiguero P, et al (2015) Differences in ventricular tachyarrythmias and antitachycardia pacing effectiveness according to the ICD indication (primary versus secondary prevention): an analysis based on the stored electrograms. J Interv Card Electrophysiol 44(2): 187-195.
- Conti S, Pala S, Biagioli V, Del Giorno G, Zucchetti M, et al. (2015) Electrical storm: A clinical and electrophysiological overview World J Cardiol7(9): 555-561.
- Sweeney MO, Sherfesee L, DeGroot PJ, Wathen MS, Wilkoff BL (2010) Differences in effects of electrical therapy type for ventricular arrhythmias on mortality in implantable cardioverter defibrillator patients. Heart Rhythm 7(3): 353-360.
10. Tan VH, Wilton SB, Kuriachan V, Sumner GL, Exner DV (2014) Impact of programming strategies aimed at reducing nonessential implantable cardioverter defibrillator therapies on mortality: a systematic review and meta-analysis. Circ Arrhythm Electrophysiol 7(1): 164-170.
11. Van Rees JB, Borleffs CJ, de Bie MK, Stijnen T, van Erven L, et al. (2011) Inappropriate implantable cardioverter defibrillator shocks: incidence, predictors, and impact on mortality. J Am Coll Cardiol 57(5): 556-562.
12. Noda T, Kurita T, Nitta T, Chiba Y, Furushima H, et al (2018) Significant impact of electrical storm on mortality in patients with structural heart disease and an implantable cardiac defibrillator Intern J of Cardiol 255: 85-89.
13. Guerra F, Shkoza M, Scappini L, Flori M, Capucci A (2014) Role of electrical storm as a mortality and morbidity risk factor and its clinical predictors: a meta-analysis. Europace 16(3): 347-353.
14. Zhu Y, Gu X, Xu C (2017) An inappropriate pacing threshold increase after repeated electrical storm in a patient with implantable cardioverter defibrillator BMC Cardiovascular Disorders 17(1): 265-270.
16. Guerra F, Accogli M, Bonelli P, Carbucicchio C, Catto V, et al. (2017) IntErnationaL eLeCTRicAl storm registry (ELECTRA): Background, rationale, study design, and expected results. Contemp Clin Trials Commun 7: 69-72.
- Wilkoff BL, Williamson BD, Stern RS (2008) PREPARE Study Investigators. Strategic programming of detection and therapy parameters in implantable cardioverter-defibrillators reduce shocks in primary prevention patients: results from the PREPARE (Primary Prevention Parameters Evaluation) study. J Am Coll Cardiol 52(7): 541-550
- Rao A, Barker D, Ng Kam Chuen MJ (2017) Shock rates in an unselected real-world cohort of patients with Medtronic Protecta implantable cardioverter defibrillators Cardiovasc Disord Med 2(4): 1-6.
- Wilkoff BL, Fauchier L, Stiles MK, Morillo CA, Al-Khatib SM, et al. (2016) 2015 Document Reviewers: 2015 HRS/EHRA/APHRS/SOLAECE Expert Consensus Statement on Optimal Implantable Cardioverter-Defibrillator Programming and Testing: Developed in partnership with and endorsed by the European Heart Rhythm Association (EHRA), the Asia Pacific Heart Rhythm Society (APHRS), and the Sociedad Latinoamericana de Estimulacion Cardiaca y Electrofisiologia (SOLAECE)-Latin American Society of Cardiac Pacing and Electrophysiology. Endorsed by the American College of Cardiology (ACC) and American Heart Association (AHA) Europace 18(2): 159-183.
20. Boriani G, Fauchier L , Aguinaga L, Beattie JM, Blomstrom Lundqvist C, et al. (2019) European Heart Rhythm Association (EHRA) consensus document on management of arrhythmias and cardiac electronic devices in the critically ill and post-surgery patient, endorsed by Heart Rhythm Society (HRS), Asia Pacific Heart Rhythm Society (APHRS), Cardiac Arrhythmia Society of Southern Africa (CASSA), and Latin American Heart Rhythm Society (LAHRS) Europace 21(1): 7-8.
- Wathen MS, DeGroot PJ, Sweeney MO, Stark AJ, Otterness MF, et al. (2004) Prospective randomized multicenter trial of empirical antitachycardia pacing versus shocks for spontaneous rapid ventricular tachycardia in patients with implantable cardioverter- defibrillators: Pacing Fast Ventricular Tachycardia Reduces Shock Therapies (PainFREE Rx II) trial results. Circulation 110(17): 2591-2596.
- Jiménez-Candil J, Durán O, Núñez J, Bravo L, Hernández J, et al. (2019) Effectiveness of first versus successive antitachycardia pacing attempts: predictors and clinical consequences. J Interv Card Electrophysiol 56(3): 349-357.
- Gunderson BD, Abeyratne AI, Olson WH, Swerdlow CD (2007) Effect of programmed number of intervals to detect ventricular fibrillation on implantable cardioverter-defibrillator aborted and unnecessary shocks. Pacing Clin Electrophysiol 30(2): 157-165.
- Santomauro M, Duilio C, Tecchia LB, Di Mauro P, Iapicca G, et al. (2010) Management of electrical storm in implantable cardioverter-defibrillator recipients. G Ital Cardiol (Rome) 11(10 Suppl 1): 37S-41S.
- Gasparini M, Proclemer A, Klersy C, Kloppe A, Lunati M, et al. (2013) Effect of long-detection interval vs standard detection interval for implantable cardioverter-defibrillators on antitachycardia pacing and shock delivery: the ADVANCE III randomized clinical trial. JAMA 309(18): 1903-1911.
- Martins RP, Blangy H, Muresan L, Freysz L, Groben L, et al. (2012) Safety and efficacy of programming a high number of antitachycardia pacing attempts for fast ventricular tachycardia: a prospective study. Europace 14(10): 1457-1464.
- Madhavan M, Friedman PA (2013) Optimal programming of implantable cardiac-defi Circulation 128(6): 659-672.
28. Saeed M, Hanna I, Robotis D, Styperek R, Polosajian L, et al. (2014) Programming implantable cardioverter-defibrillators in patients with primary prevention indication to prolong time to first shock: results from the PROVIDE study. J Cardiovasc Electrophysiol 25(1): 52-59.
- Theuns D, Klootwijk AP,Kimman GP, Szili-Török T, Roelandt JR, et al. (2001) Initial Clinical Experience With a New Arrhythmia Detection Algorithm in Dual Chamber Implantable Cardioverter Defibrillators. Europace 3(3): 181-186.
- Gold MR, Shorofsky SR, Thompson JA, Kim J, Schwartz M, et al. (2002) Advanced Rhythm Discrimination for Implantable Cardioverter Defibrillators Using Electrogram Vector Timing and Correlation, J Cardiovasc Electrophysiol 13(11): 1092-1097.
- Wilkoff BL, Ku ̈hlkamp V, Volosin K, Ellenbogen K, Waldecker B, et al. (2001) Critical analysis of dual-chamber implantable cardioverter-defibrillator arrhythmia detection: results and technical considerations. Circulation 103(3): 381-386.
- Mletzko R, Anselme F, Klug D, Schoels W, Bowes R, et al. (2004) Enhanced Specificity of a Dual Chamber ICD Arrhythmia Detection Algorithm by Rate Stability Criteria. Pacing Clin Electrophysiol 27(8): 1113-1119.
- Zdarek J, Israel CW (2016) Detection and discrimination of tachycardia in ICDs manufactured by St. Jude Medical. Herzschrittmachertherapie Elektrophysiologie 27(3): 226-239.
- Scott PA, Silberbauer J, McDonagh TA, Murgatroyd FD (2014) Impact of prolonged implantable cardioverter-defibrillator arrhythmia detection times on outcomes: a meta-analysis. Heart Rhythm 11(5): 828-835.
35. Zeitler EP, Sanders GD, Singh K, Greenfield RA, Gillis AM, et al. (2018) Single vs. dual chamber implantable cardioverter-defibrillators or programming of implantable cardioverter-defibrillators in patients without a bradycardia pacing indication: systematic review and meta-analysis Europace 20(10): 1621-1629.
36. Regoli F, Graf D, Schaer B, Duru F, Ammann P, et al. (2019) Arrhythmic episodes in patients implanted with a cardioverter-defibrillator: results from the Prospective Study on Predictive Quality with Preferencing Pain Free ATP therapies (4P). BMC Cardiovascular Disorders 19: 146-158.
- Fontenla A, Martinez Ferrer JB, Alzueta J, Vinolas X, Garcia Alberola A, et al. (2016) Incidence of arrhythmias in a large cohort of patients with current implantable cardioverter defibrillators in Spain: results from the UMBRELLA registry. Europace 18(11): 1726-1734.
- Cao J, Gillberg JM, Swerdlow CD (2012) A fully automatic, implantable cardioverter-defibrillator algorithm to prevent inappropriate detection of ventricular tachycardia or fibrillation due to T-wave oversensing in spontaneous rhythm. Heart Rhythm 9(4): 522-530.
39. Moss AJ, Schuger C, Beck CA, Brown MW, Cannom DS, et al. (2012) Reduction in Inappropriate Therapy and Mortality through ICD Programming. N Engl J Med 367: 2275-2283.
40. Auricchio A, Schloss EJ, Kurita T, Meijer A, Gerritse B, et al. (2015) Low inappropriate shock rates in patients with single- and dual/triple-chamber implantable cardioverter-defibrillators using a novel suite of detection algorithms: Pain Free SST trial primary results. Heart Rhythm 12(5): 926-936.
- Peterson PN, Greenlee RT, Go AS, Magid DJ, Cassidy Bushrow A, et al. (2017) Comparison of Inappropriate Shocks and Other Health Outcomes Between Single‐ and Dual‐Chamber Implantable Cardioverter‐Defibrillators for Primary Prevention of Sudden Cardiac Death: Results From the Cardiovascular Research Network Longitudinal Study of Implantable Cardioverter‐ J Am Heart Assoc 6(11): e006937.
- Kolb C, Sturmer M, Sick P, Reif S, Davy JM, et al. (2014) Reduced risk for inappropriate implantable cardioverter-defibrillator shocks with dual-chamber therapy compared with single-chamber therapy: results of the randomized OPTION study. JACC Heart Fail2(6): 611-619.
- Hofer D, Steffel J, Hurlimann D, Haegeli L, Duru F, et al. (2017) Long-term incidence of inappropriate shocks in patients with implantable cardioverter defibrillators in clinical practice-an underestimated complication? J Interv Card Electrophysiol 50: 219-226.
- Ruiz Granell R, Dovellini EV, Dompnier A, Khalighi K, Garcia Campo E, et al. (2019) Algorithm-based reduction of inappropriate defibrillator shock: Results of the Inappropriate Shock Reduction with PARAD+ Rhythm Discrimination-Implantable Cardioverter Defibrillator Study. Heart Rhythm16(9): 1429-1435.
45. Powell BD, Asirvatham SJ, Perschbacher DL, Jones PW, Cha YM, et al. (2012) Noise, artifact, and oversensing related inappropriate ICD shock evaluation: ALTITUDE noise study. Pacing Clin Electrophysiol 35(7): 863-869.
46. Santomauro M, Petretta M, Riganti C (2019) Pseudo-Electrical Storm in Patients with Implanted Cardioverter Defibrillator. J Cardiovas Res 2(4): 1-5.
47. Mozes A, DeNofrio D, Pham DT, Homoud MK (2011) Inappropriate implantable cardioverter-defibrillator therapy due to electromagnetic interference in patient with a HeartWare HVAD left ventricular assist device. Heart Rhythm 8(5): 778-780.
48. Camsari A, Yilmaz DC, Ozcan T (2010) Inappropriate shock delivery by implantable cardioverter defibrillator due to electrical leak from bathroom tap. Clin Res Cardiol 99(11): 761-763.
49. Ginwalla M, Battula S, Dunn J, Lewis WR (2010) Termination of electrocution induced ventricular fibrillation by an implantable cardioverter defibrillator. Pacing Clin Electrophysiol. 33(4): 510–512.
50. Swerdlow CD, Asirvatham SJ, Ellenbogen KA (2014) Troubleshooting Implanted Cardioverter Defibrillator Sensing Problems I. Circulation: Arrhythmia and Electrophysiology 7(6): 1237-1261.
51. Hashmani S, Khan AH (2017) Twiddler’s syndrome presenting as life threatening electrical storm. J Pak Med Assoc 67(10): 1612-1614.
52. Ferretto S, Brunzin K, Pettenuzzo K (2019) Inappropriate implantable cardioverter defibrillator shocks due to atrial far-field on the tip-to- ring channel for lead dislodgement. J Arrhythm 35(3): 562-564.
53. Ellenbogen KA, Gunderson BD, Stromberg KD (2013) Performance of Lead Integrity Alert to assist in the clinical diagnosis of implantable cardioverter defibrillator lead failures: analysis of different implantable cardioverter defibrillator leads. Circ Arrhythm Electrophysiol 6(6): 1169-1177.
- Swerdlow CD, Kalahasty G, Ellenbogen KA (2016) Implantable Cardiac Defibrillator Lead Failure and Management.J Am Coll Cardiol 67(11): 1358-1368.
- Providência R, Kramer DB, Pimenta D, Babu GG, Hatfield LA, et al. (2015) Transvenous Implantable Cardioverter-Defibrillator (ICD) Lead Performance: A Meta-Analysis of Observational Studies.J Am Heart Assoc 4(11): e002418.
56. Santomauro M, Petretta M, Riganti C, Francesco Elia, Riccardo Franco (2019) Emergency in False-Electrical Storm in Patients with Implanted Cardioverter Defibrillator. Journal of Cardiology and Clinical Practice 2(1): 1-6.
- BeckLR, Ostermayer DG, Ponce JN , Srinivasan S, Wang HE (2019) Effectiveness of Prehospital Dual Sequential Defibrillation for Refractory Ventricular Fibrillation and Ventricular Tachycardia Cardiac Arrest. J Prehosp Emerg Care 23(5): 597-602.
- Hajjar K, Berbari I, El Tawil C, Bou Chebl R, Abou Dagher G (2018) Dual defibrillation in patients with refractory ventricular fibrillation. Am J Emerg Med 36(8): 1474-1479.
- Merlin MA, Tagore A, Bauter R, Arshad FH (2016) Case Series of Double Sequence Defibrillation. Prehosp Emerg Care 20(4): 550-553.
60. Cabañas JG, Myers JB, Williams JG, De Maio VJ, Bachman MW (2015) Double sequential external defibrillation in out-of-hospital refractory ventricular fibrillation: A report of ten cases. Prehosp Emerg Care 19(1): 126-130.
61. Link MS, Berkow LC, Kudenchuk PJ, Halperin HR, Hess EP (2015) American Heart Association Guidelines Update for Cardiopulmonary Resuscitation and Emergency Cardiovascular Care - Part 7: Adult Advanced Cardiovascular Life Support. Circulation 132(18): S444-S464.
62. Soar J, Callaway CW, Aibiki M, Böttiger BW, Brooks SC (2015) Part 4: advanced life support: 2015 International Consensus on Cardiopulmonary Resuscitation and Emergency Cardiovascular Care Science With Treatment Recommendations. Resuscitation 95: e71-e120.
- Baranchuk A, Refaat MM, Patton KK, Chung MK, Krishna (2018) Cybersecurity for Cardiac Implantable Electronic Devices: What Should You Know? J Am Coll Cardiol 71(11): 1284-1288.
- Sandgren E, Rorsman C, Engdahl J (2015) Low rate of and rapid attention to inappropriate ICD shocks with remote device and rhythm monitoring: a qualitative study. BMJ Open Heart 2(1): e000249.
- Shakibfar S, Krause O, Lund-Andersen C, Aranda A, Moll J (2019) Predicting electrical storms by remote monitoring of implantable cardioverter-defibrillator patients using machine learning.Europace 21(2): 268-274.