Residual effects of Lithium in Muscle and Organ Tissues of Sheep Post-Ingestion of Lithium Chloride
KB Groth1, JS Horne1, DE Konetchy*2, SL Gilbert3 and A Ahmadzadeh2/h3>
1Idaho Department of Fish and Game, 3316 16th Street, Lewiston, ID 83501, USA
2Department of Animal, Veterinary and Food Science, University of Idaho, Moscow, ID 83844 USA
3Department of Fish and Wildlife Sciences, University of Idaho, Moscow, ID 83844, USA
Submission:August 09, 2022; Published:September 19, 2022
*Corresponding author: DE Konetchy, Department of Animal, Veterinary and Food Science, University of Idaho, Moscow, ID 83844, USA
How to cite this article: KB Groth, JS Horne, DE Konetchy, SL Gilbert, A Ahmadzadeh. Residual effects of Lithium in Muscle and Organ Tissues of Sheep
Post-Ingestion of Lithium Chloride. Dairy and Vet Sci J. 2022; 15(2): 555910.DOI: 10.19080/JDVS.2022.15.555910
Abstract
Conditioned taste aversions (CTA) occur when animals associate gastrointestinal distress with a particular food source. CTA strategy can be used to reduce animal consumption of an undesirable feedstuff. Lithium chloride (LiCl) has been used in wild ungulates as a CTA and could be used in white tail deer (WTD) as a potential CTA. Consumption of LiCl by WTD may leave residue in meat which may be consumed by a human. The objectives of this study were to examine the effect of dietary LiCl on kinetics and depletion of lithium in muscle, kidney, and liver tissue in adult domestic sheep (model for WTD). In experiment1, eleven adult sheep orally received either 150 mg LiCl/kg BW (n = 8) or placebo (n = 3). Using aseptic procedure, muscle biopsies were taken at 4,8,12,24,48,96,192, and 240 hours post LiCl ingestion and lithium concentrations were measured. In experiment2, sixteen adult sheep received either 450mg LiCl/kg BW (n = 14) or placebo (n = 2). In experiment3, nine adult sheep orally received a single dose of 150mg/kg BW. Three-animal groups were euthanized at 7,24, and 96 hours post-LiCl ingestion and muscle, liver, and kidney samples were harvested to measure lithium concentrations. Low dose of LiCl reached a maximum level in muscle 24 hours post-ingestion and returning to basal levels (P = 0.72) by 192 hours. High mortality (12 of 14; 86%) occurred following high dose administration resulting in an inability to determine maximum concentration levels or appreciate differences between muscle, organ tissue types and over time. Lithium concentrations were greater (P<0.01) in liver and kidney compared to muscle at 7 and 24 hours post ingestion, but no difference in lithium concentration was detected in the three tissues at 96 hours (P > 0.05). It appears that a withdrawal period in muscle tissue for low dose LiCl in domestic sheep is 192 hours. The toxic threshold for domestic sheep, and likely other small ruminants, occurs between 150-450mg LiCl/kg body weight.
Keywords:Crop depredation, Deer, Lithium chloride, Lithium chloride toxicity, Small ruminants, Taste aversion
Abbreviations:CTA: Conditioned Taste Aversion; WTD: White-tailed deer; LiCl: Lithium Chloride
Introduction
White-tailed deer (Odocoileus virginianus; WTD, hereafter) are one of the most widespread large mammal species of North America, with correspondingly large impacts on society, both positive (e.g., hunting, wildlife viewing) and negative (e.g., car collisions, crop depredation). White-tailed deer inhabit a variety of areas, occurring almost where digestible forage is available and accessible habitat cover is nearby. In recent years, population numbers have drastically increased in many areas of the Western United States, potentially due to their extreme adaptability and versatility [1]. High population densities have been thought to increase dispersal and movement rates, likely causing them to travel further across the landscape in search of available resources [2]. As deer movement and dispersal rates increase, more encounters with agricultural fields containing nutritious crops occur [2], resulting in an increase in crop depredation rates.
To mitigate costs associated with abundant deer while maintaining recreational and economic benefits, there is a pressing need to find effective deer deterrents. In the past, multiple deterrent methods targeted at reducing deer damage have been tested, including propane exploders and other frightening devices, fencing, and lethal removal [3-5]. Although previously tested deterrents have resulted in a wide range of effectiveness, wildlife managers are still searching for a deterrent method that is cost-effective with high efficacy rates across a multitude of wildlife species. One promising method yet to be tested in an open field
setting for deterring WTD is the use of lithium chloride (LiCl), a
gastrointestinal toxicant that has successfully been used to create
taste aversions to specific food items in both carnivores and
ruminants.
Previous studies have shown high efficacy in reducing the
amount of food consumed after LiCl was ingested as treated
animals associated targeted food sources with gastrointestinal
distress [6-9]. However, most of these studies were conducted
in controlled, captive feeding trials where ruminants, as well as
carnivores, were given the choice to consume food items pre- and
post-ingestion of LiCl [8-11]). Due to LiCl creating strong taste
aversions across multiple species, it has a potential of being a
successful deterrent method in reducing WTD crop depredations.
Before implementation of LiCl as a depredation deterrent
in an open field setting can be utilized, key issues regarding
toxicity and accumulation in deer tissues needs to be addressed.
One challenge with using LiCl is that crop depredation season
overlaps with hunting season in many parts of WTD habitat range
(i.e., late summer through fall). As a result, it is important to first
understand withdrawal factors in different types of animal tissues
that may be consumed by humans. Information regarding LiCl and
pharmacokinetic data in small ruminants to LiCl is lacking, which
compelled the need for this study prior to using LiCl as a deterrent
in an open field setting.
Although the eventual intent is to use LiCl as a deterrent on
WTD, domestic sheep were used in this study as a surrogate due
to logistics and cost. Domestic sheep have been used in a variety of
feeding trials to test the efficacy and necessary dosage needed of
LiCl to create an effective aversion [12-14]. Higher dosages often
result in a greater aversion effect [15], but toxicity levels and tissue
withdrawal times have yet to be reported. Thus, we addressed the
following research questions:
1) What are the concentration levels of LiCl over time in
differing body tissues at a realistic dose range that may be
consumed by a deer in an open field setting based on LiCl
withdrawal in the sheep model?
2) What is the maximum realistic dosage that could be
consumed in a field setting and is this toxic for small ruminants?
Material and Methods
Animal use and protocols were approved by the Institutional
Animal Care and Use Committee at the University of Idaho
(IACUC-2017-70). The kinetics and toxicity of LiCl was tested
using adult domestic sheep located at the University of Idaho Sheep
Center in Moscow, Idaho. Suffolk, Targhee, and Targhee/Polypay
crossbred sheep were used in this study, and all experiments were
conducted at the University of Idaho Sheep Center. All animals
were housed in an indoor/outdoor covered barn; feed and water
were available ad libitum. Grain was provided once a day after
biopsy samples had been collected.
Before each experiment began, sheep were weighed on an
electric platform scale (+/- 1 kg), so that the appropriate dosage
of LiCl for each experiment and animal could be determined on a
per-kg of body weight basis. Subsequently, the appropriate amount
of LiCl was dissolved in 240 mLs of cold water, and administered
via drenching (i.e., orally inserting a lubricated stomach tube to
the level of the abomasum). Control animals were drenched only
with 240mLs of cold water minus the LiCl. Three experiments
were conducted to analyze and compare lithium concentration in
kidney, liver, and muscle tissues at a low (150 mg/kg) and high
(450 mg/kg) dosage. In all experiments animals were visually
observed for behavioral changes. All tissue samples were analyzed
at the University of Idaho toxicology lab.
In the first experiment, eleven adult sheep were used to assess
the kinetics and depletion of LiCl in muscle tissue at a 150mg
LiCl/kg of body weight dosage, which was considered a low dose
[7,9,16]. On the first experimental day each treated sheep (n =
8) was weighed and orally drenched with a single dose of LiCl
[7,9]. Controls (n = 3) received a drench of water only. Muscle
biopsy samples (~1g per sample) were extracted from the triceps
and upper thigh muscle (biceps femoris, vastus lateralis, and
semitendinosus) for lithium concentration analysis. Animals were
physically restrained during muscle biopsy. Once restrained, the
area of biopsy was surgically prepared, and a local anesthetic
(Lidocaine 1%) was administered within the area to affect. The
skin was incised, and a punch biopsy tool (MiltexS® 6mm, Princeton,
NJ.) was used to remove approximately 1g of muscle sample. Each
1g sample of muscle tissue was placed into a sterile, labeled Whirl-
Pak® and frozen until analyses for lithium quantification.
It has been reported that the maximum level of lithium in
blood occurs 4-8 hours post-ingestion [17,18], and animals were
completely cleared of lithium after 240 hours [18]. Collection of
muscle biopsy samples were made at 4, 8, 12, 24, 48, 96, 192,
and 240 hours post LiCl ingestion to cover the entire time span
between maximum peak levels and complete lithium metabolism.
In the second experiment, sixteen adult sheep were used to
assess the kinetics and depletion time at 3x the recommended
150mg LiCl/kg body weight dosage. On the first day of the
experiment each sheep was weighed and orally drenched with
450mg LiCl/kg body weight in cold water (n = 14) or just cold
water (n = 2). Muscle biopsies were once again collected following
the protocol previously described for experiment 1. If an animal
died during the trial a necropsy was immediately conducted and
1g of kidney, liver, and muscle samples were each collected from
the deceased animal. During the necropsy all other major organs
and muscle groups were observed by a veterinarian to determine
if the ingested LiCl had resulted in reportable necropsy findings.
In the third experiment, nine adult sheep were used to analyze
lithium concentrations within kidney, liver, and muscle tissues,
at time intervals surrounding the peak lithium concentration
for low dose (150 mg/kg) ingestion. Based on the results from experiment 1, the peak lithium concentration occurred ~25
hours post-ingestion. On day one all sheep were weighed and
orally received a single dosage of 150 mg LiCl/kg body weight
mixed with 240 mL of cold water. Sheep were terminated using a
penetrating cap and bolt system with exsanguination at intervals
surrounding peak lithium concentration times. Group 1 (n = 3)
were terminated 7 hours post LiCl ingestion, group 2 (n = 3) 24
hours post LiCl ingestion, and group 3 (n = 3) 96 hours post LiCl
ingestion. Tissue samples (1g) from the kidney, liver, and muscle
were collected from each animal. Field necropsies were conducted
to assess any notable findings that may have been related to LiCl
ingestion.
To measure lithium concentrations in tissues, a Perkin Elmber®
Optima 8300 Inductively Coupled Plasma-Optical Emission
Spectroscopy (ICP-OES) was used. The ICP-OES equipment
determined the lithium concentration within each tissue sample
using plasma and a spectrometer (operating conditions; plasma:
15 L/min, auxiliary: 0.2 L/min, nebulizer: 0.73 L/min, flow rate:
1.5 mL/min, and wash rate: 2.00 mL/min) [19]. Equipment
was calibrated with concentrated redistilled trace metal grade
nitric acid and water [19]. Tissue samples were frozen until all
samples for the trial had been collected and all samples were
tested consecutively to avoid recalibrating equipment multiple
times. All samples were analyzed on a wet weight basis, and 1g
of tissue sample was added to, and mixed with, 3 mL trace metal
grade nitric acid in a 10 mL test tube [19]. The tubes were then
heated for 6 hours at 30 °C, then 1 hour at 70 °C, and finally for
8 hours at 120 °C [19]. The tubes were then cooled, vortexed,
and centrifuged as needed to produce transparent solutions to
prevent clogs from occurring within the nebulizer [19]. If particles
remained within the solution a 0.45 Acrodisc filter was used to
eliminate the remaining particles [19].
Data on the effects of LiCl on tissue lithium concentration
were analyzed using a general linear model (GLM) procedure in
SAS [20]. The model included the fixed effect tissue types (muscle,
live, kidney), time and their two-way interaction with significance
declared at P < 0.05. Using SAS GLM, the effect of low dose LiCl
on muscle tissue concentration was also analyzed using GLM. The
model included the effect of time.
Results and Discussion
Experiment 1:
Feeding low dose (150 mg/kg) of LiCl caused an increase
(P < 0.01) in muscle Lithium at hours 4, 8, 12, 24, 48, and 96 as
compared with baseline lithium concentrations. At hours 192 and
240 the muscle concentration of lithium was not different from
baseline levels at (P ≤ 0.9). Lithium concentration in muscle tissue
peaked at (~24 hours post-ingestion (7.8 μg/g, Figure 1). Lithium
concentrations declined thereafter and reached baseline level at
192 hours post-ingestion.
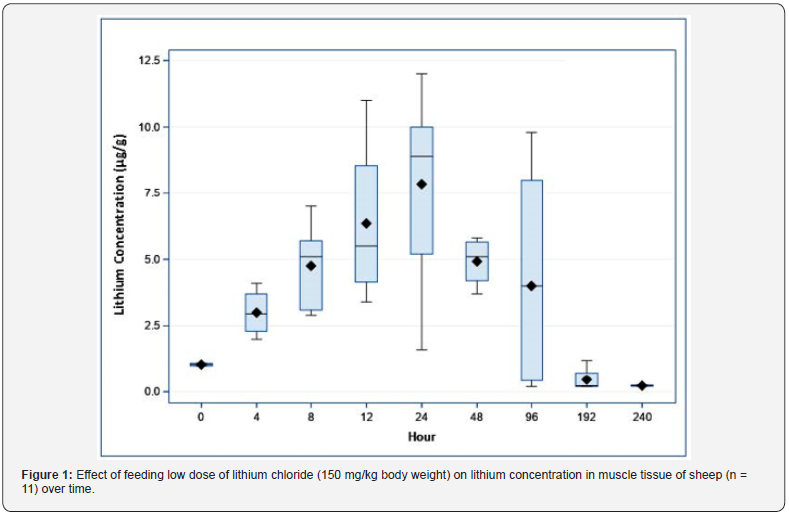
Experiment 2:
Based on the predicted lithium concentration in the muscle
tissues after feeding a high dose of LiCl (450 mg/kg), lithium
concentration peaked at approximately 100 hours post-ingestion
(Figure 2). Lithium concentrations slowly declined thereafter,
and never reached basal level by the end of the experiment (240
hours). A high mortality rate at this dosage was observed (12 out
of 14 total treated, ~86% mortality) with most of the mortalities
occurring after 73 hours post-ingestion. Approximate death
times post ingestion of 450 mg/kg LiCl were as follows; 48h - 1
dead; 73h - 2 dead; 97h - 2 dead; 145h - 2 dead; 169h - 5 dead.
Kidney, liver, and muscle tissue samples were obtained from all
the animals that died. Behavioral observations were once again
recorded for treated animals following LiCl ingestion. Treated
animals appeared unaffected until 24 hours post-ingestion when
they stopped eating, drinking, and moving around the containment
area. Because of the high mortality rate, we were unable to
construct a complete depletion curve for this concentration.
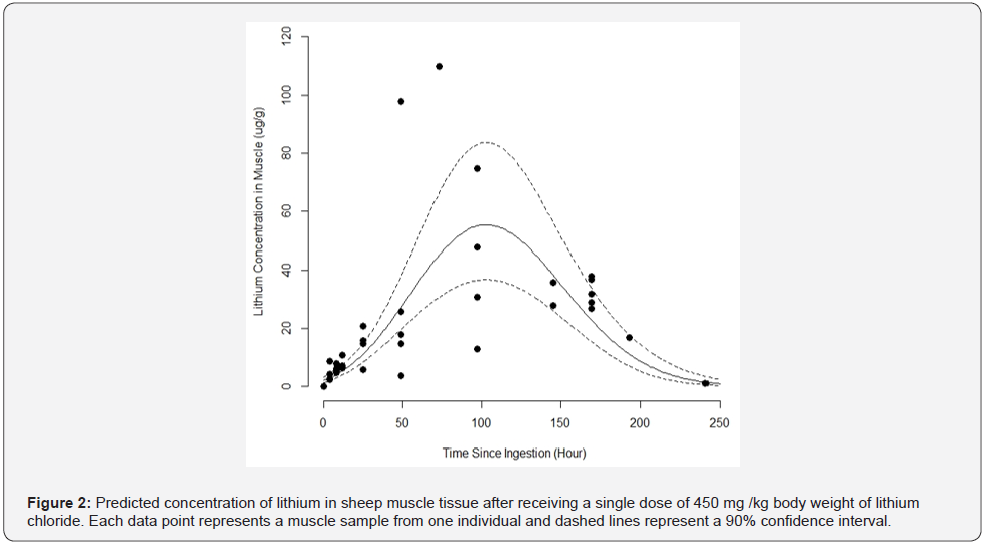
Experiment 3:
Mean lithium concentrations were different among muscle,
kidney, and liver tissues within 7 hours after ingestion. Mean
lithium concentrations in both liver and kidney tissues were
greater than muscle at 7 and 24 hours post ingestion (P < 0.01;
Table 1). There was no difference in lithium concentrations
between the three tissues at 96 hours (P > 0.05) (Table 1). Mean
lithium concentrations remained elevated (P < 0.01) in both
liver and kidney in the first 24 hours after LiCl ingestion but
returned to basal level at 96 hours after ingestion. Although, the
overall concentration of lithium was less in muscle tissue, lithium
concentrations remained elevated (P < 0.01) in muscle in the first
24 hours after LiCl ingestion and returned to basal level at 96
hours after ingestion (Table 1). There was not a tissue type by
time interaction effect on Lithium concentrations.

a,b Means with different superscripts within a column differ (P< 0.05)
x,y Means with different superscripts within a row differ (P<0.05)
A 150 mg LiCl/kg body weight was selected as the low dose
based on previous reports of effectiveness in creating taste aversion
in domestic sheep, cattle, and caribou [9,22,23]. Administering
LiCl dosages greater than 300 mg/kg body weight is rare within
the literature, and an exact toxic dosage in small ruminants has
yet to be determined. The LiCl toxicity in mice occurred at a 600
mg LiCl/kg body weight [24], and to avoid exceeding the toxic
threshold for ruminants the high dosage was reduced to 450
mg LiCl/kg body weight in the current study. However, with the
multiple mortalities occurring post-ingestion the toxic threshold
apparently was exceeded in the sheep indicating the LiCl toxic
threshold maybe even less than 450 mg LiCl/kg body weight.
Maximum lithium concentration levels and withdrawal periods
within muscle tissue may vary by dosage, and among animals to
an extent. Most notably in experiment 1, one sheep at 96 hours
had a greater Lithium concentration (9.8 μg/g) causing a larger
variation in the data at that time point (Figure 1). Interestingly,
mean muscle lithium concentration at 96 hours post ingestion
was similar to the pre-ingestion concentration when the data from
that sheep was not included in the data analysis. As indicated, at
the low dosage, lithium concentration increased within muscle
tissue starting with the first biopsy samples taken at 4 hours and
continued to increase until the maximum concentration value
occurred at approximately 24 hours. Following the peak, lithium
concentrations quickly declined and returned to basal levels by
192 hours. (Figure 1) Although lithium in kidney and liver samples
did not return to baseline concentrations from the low dose, at 96
hours post-ingestion, there was not a difference between lithium
concentrations among the three different tissues suggesting most
of the lithium had been metabolized and excreted leaving behind
small residual amounts in all tissues. These results are similar
to withdrawal periods of lithium in different types of excreta in
sheep and goats previously reported [18].
In this study feed and water intake pre- and post-ingestion
were not directly quantified, but treated animals were observed
for behavioral changes. Although previous studies have observed
signs of malaise (head droop and inactivity) [21] and an aversion
to food post LiCl ingestion [7,15], we did not observe either of
these behavior changes. Treated sheep were consuming provided
alfalfa immediately following LiCl drenching and continued to do
so throughout the study period. The low dose LiCl may not have
been high enough to produce the taste aversion in sheep, and
perhaps a greater dose of LiCl (200-300 mg/kg) may produce the
taste aversion without producing the toxic effects seen at a LiCl
dose of 450 mg/kg.
Only 2 of the 14 individuals that received high dosage (450
mg/kg BW) did not succumb to toxicity, and after 240 hours postingestion
muscle tissue samples from the surviving animals had
yet to reach basal level. Thus, a complete withdrawal time for a
dosage of 450 mg LiCl/kg body weight was not determined. Despite
supportive treatment for dehydration animals succumbed within a
few hours of clinical signs. As indicated, majority of the mortalities
occurred between 36- and 193-hours post-ingestion. Multiple
symptoms of toxicity were observed including lack of appetite,
malaise, severe dehydration, hypoglycemia, muscular tremors,
increased heart rate, and extreme diarrhea. Necropsies were
conducted by a veterinarian, and cause of death was determined
for each deceased animals. In the absence of any additional postmortem
findings, it was determined that all animals had died due
to LiCl overdose, and that 450 mg LiCl/kg body weight appears to
be a lethal dose for small ruminants.
Although treated animals only received a single dosage of LiCl,
the high-level potency of the chemical compound resulted in death
as the physiological responses in the body, and especially the
kidneys, were not able to process and excrete excess LiCl resulting
in accumulation and eventual death [17,25]. Kidneys are the main
processing organ that excretes LiCl [17,25], and excess lithium can
disrupt the absorption of salt and water, often leading to polyuria
[26]. If the kidneys are not able to process and excrete the ingested
amount of lithium, excess amounts begin to accumulate in other
tissues [17]. This is likely what occurred in the high dose trial
and why our results show no difference in lithium concentrations
among the tissue types. Once lithium levels in the kidney exceeded
maximum intake, surplus lithium may have deposited in the liver
and muscle tissues, resulting in all 3 tissue types containing high
concentration levels. However, in the low dose, the highest lithium
concentrations were in the kidneys, followed by liver, and the least
amount of lithium concentration was in muscle tissue. This was
likely because of the kidneys being able to function correctly with
a manageable intake of lithium. Overdosing was not an issue as
the amount of ingested lithium was processed and excreted by the
kidneys without excess accumulation.
Conclusion
The muscle concentration of lithium at a low dose of 150 mg/
kg body weight of LiCl administration reached baseline lithium
in muscle tissues by 192 hours post-ingestion. Although, the
withdrawal period within the liver and kidney for this dosage was
not established, the lack of difference in lithium concentration
between the three tissues (muscle, liver, kidney) at 96 hours
suggests lithium concentrations of liver and kidney would not
differ from baseline by 192 hours. Likewise, high dose withdrawal
periods for all 3 tissue types were undetermined due to 450 mg
LiCl/kg body weight being lethal for many sheep. It appeared
that kidney tissues retain the greatest amount of lithium, followed
by liver tissues, and lastly muscle tissues. It is important to
acknowledge the toxic threshold for domestic sheep, and likely
for other small ruminants, lies between 150-450 mg LiCl/kg body
weight.
Although we didn’t notice any instant food aversion after
ingesting LiCl, this chemical could be a useful deterrent for
lowering WTD crop destruction. Although sheep and deer have similar body sizes and rumen capacities, it should be noted that toxicity effects and withdrawal times for each tissue type may
differ between species. Based on the results of the current study,
a 192-hour withdrawal period in muscle tissue for a low dose of
LiCl in domestic sheep may be taken into consideration; however,
the analyses for other tissue types at low dosages and for all tissue
types at high dosages were inconclusive, hence withdrawal period
cannot be recommended.
Therefore, we suggest that before field implementation and
human consumption of an animal that has ingested LiCl, more
trials are necessary that include using LiCl at a dose range of 200 -
300 mg/kg for longer time periods, with larger samples sizes, and
incorporate a variety of ruminant species.
Acknowledgement
The authors thank University of Idaho Sheep Center manager
and staff and Washington State University Veterinarian students
for helping conduct the experiments.
Conflicts of Interest
No potential conflict of interest was reported by the authors.
References
- Idaho Department of Fish & Game (2013) White-tailed deer. Surveys and Inventories. Statewide Report. Boise, ID.
- Lesage L, M Crete, J Huot, A Dumont, J Ouellet (2000) Seasonal home range size and philopatry in two northern white-tailed deer populations. Canadian Journal of Zoology 78: 1930-1940.
- Hyngstrom SE, Craven SR (1988) Electric fences and commercial repellents for reducing deer damage in cornfields. Wildlife Society Bulletin 16: 291-296.
- Gilsdorf JM, SE Hygnstrom, KC VerCauteren, EE Blankenship, RM Engeman (2004) Propane exploders and Electronic Guards were ineffective at reducing deer damage in cornfields. Wildlife Society Bulletin 32: 524-531.
- Williams SC, AJ DeNicola, IM Ortega (2008) Behavioral responses of white-tailed deer subjected to lethal management. Canadian Journal of Zoology 86(12): 1358-1366.
- Olsen JD, MH Ralphs, MA Lane (1989) Aversion to eating poisonous larkspur plants induced in cattle by intraruminal infusion with lithium chloride. Journal of Animal Science 67(8): 1980-1985.
- Du Toit JT, FD Provenza, A Nastis (1991) Conditioned taste aversions: how sick must a ruminant get before it learns about toxicity in foods? Applied Animal Behavior Science 30(1-2): 35-46.
- Ralphs MH (1997) Persistence of aversions to larkspur in naïve and native cattle. Journal of Range Management 50: 367-370.
- Brown WK, WK Hall, LR Linton, RE Huenefeld, LA Shipley (2000) Repellency of three components to caribou. Wildlife Society Bulletin 28: 365-371.
- Burns RJ (1980) Evaluation of conditioned predation aversion for controlling coyote predation. The Journal of Wildlife Management 44(4): 938-942.
- Burritt EA, FD Provenza (1991) Ability of lambs to learn with a delay between food ingestion and consequences given meals containing novel and familiar foods. Applied Animal Behaviour Science 32: 179-189.
- Burritt EA FD Provenza (1989) Food aversion learning: conditioning lambs to avoid a palatable shrub (Cercocarpus montanus). Journal of Animal Science 67(3): 650-653.
- Scott CB, FD Provenza, RE Banner (1995) Dietary habits and social interactions affect choice of feeding location by sheep. Applied Animal Behaviour Science 45: 225-237.
- Wang J, FD Provenza (1997) Dynamics of preference by sheep offered foods varying in flavors, nutrients, and a toxin. Journal of Chemical Ecology 23: 275-288.
- Launchbaugh KL, FD Provenza (1994) The effect of flavor concentration and toxin dose on the formation and generalization of flavor aversions in lambs. Journal of Animal Science 72(1): 10-13.
- Pacίfico da Silva I, B Soto-Blanco (2010) Conditioning taste aversion to Mascagnia rigida (Malpighiaceae) in sheep. Research in Veterinary Science 88(2): 239-241.
- Okusa MD, Crystal LJT (1994) Clinical manifestations and management of acute lithium intoxication. The American Journal of Medicine 97(4): 383-389.
- Manuelian CL, E Albanell, M Rovai, G Caja (2016) How to create conditioned taste aversion for grazing ground covers in woody crops with small ruminants. Journal of Visualized Experiments 110: 1-6.
- Anderson K, G Farwell, P Gibson, B Ricks, T Case (2018) Total recoverable elements in biological, plant, and animal tissue and feed samples. Analytical Sciences Laboratory Standard Methods. Moscow, ID.
- SAS Institute (2015) SAS Version 9.4. SAS Institute Inc. Cary, NC, USA.
- Manuelian CL, E Albanell, M Rovai, AAK Salama, G Caja (2014) Effect of breed and lithium chloride dose on the conditioned aversion to olive tree leaves (Olea europaea L.) of sheep. Applied Animal Behaviour Science 155: 42-48.
- Ralphs MH (1992) Continued food aversion: training livestock to avoid eating poisonous plants. Journal of Range Management 45(1): 46-51.
- Provenza FD, JV Nolan, JJ Lynch (1993) Temporal contiguity between food ingestion and toxicosis affects the acquisition of food aversions in sheep. Applied Animal Behaviour Science 38(4): 269-281.
- Zakaria NA, N Embi, HM Sidek (2010) Suppresion of Plasmodium berghei parasitemia by LiCl in an animal infection model. Tropical Biomedicine 27(3): 624-631.
- Timmer RT, JM Sands (1999) Lithium intoxication. Journal of the American Society of Nephrology 10: 666-674.
- Myers JB, TO Morgan, SL Carney, C Ray (1980) Effects of lithium on the kidney. Kidney International 18: 601-608.
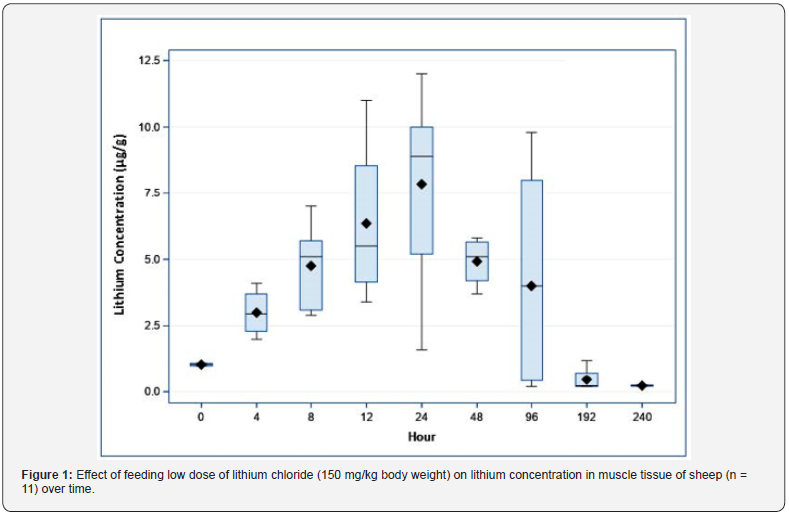
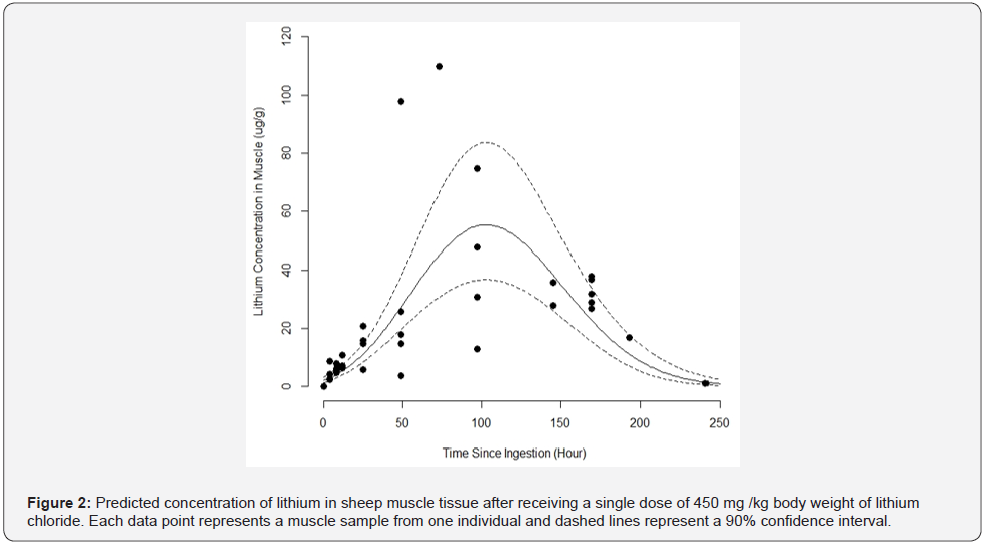
