Sustainable Transportation Utilizing Electric Arc Furnace (EAF) Steel Slags
Charles Ochola1, Kelly Cook2, John Yzenas3 and Keith Moo-Young4*
1President National Slag Association, USA
2Director Technical Marketing Edward C Levy Company, USA
3Principal Yzenas Consulting, USA
4Rensselaer Polytechnic Institute, USA
Submission: March 30, 2023; Published: April 11, 2023
*Corresponding Author: Keith Moo-Young, Rensselaer Polytechnic Institute, USA
How to cite this article: Charles O, Kelly C, John Y, Keith M-Y. Sustainable Transportation Utilizing Electric Arc Furnace (EAF) Steel Slags. Civil Eng Res J. 2023; 13(4): 555870. DOI 10.19080/CERJ.2023.13.555870
Introduction
Electric Arc Furnace (EAF) steel slag is a versatile renewable, sustainable, durable, and environmentally friendly aggregate produced during the steel making process at an electric arc steel making mill. EAF steel slag is the co-product of steel production that is used in a variety of applications including but not limited to construction, agricultural, and environmental remediation. Steel slag, or ferrous slag, is different from ferroalloy or non-ferrous slags from the ferroalloy, copper, nickel, zinc, and phosphorus industries. Ferrous slag, unlike ferroalloy and non-ferrous slags, is defined as a product or co-product, intentionally produced during the iron and steel making process. When EAF steel slag is processed properly, it is an excellent option for construction applications.
The focus of this paper will be on the utilization of EAF steel slag in road construction. There will be a discussion on the production, processing, and classification of EAF slag aggregates followed by a characterization of these products by considering both their physical and chemical/mineralogical properties. Next will be a discussion on the utilization of these products in road construction highlighting their performance in contrast to traditional materials. The paper will then conclude with a discussion on the sustainability aspect of the incorporation of EAF slag products into road construction by emphasizing the potential for carbon dioxide sequestration from the atmosphere.
Production and Processing
The production/processing of EAF steel slag aggregates is typically very similar, in both flow and equipment, to that of natural aggregates with only extraction being the primary difference. This has been highlighted in the 2017 Construction Aggregates - Product Category Rule (PCR), as well as the upcoming 2023 version. The processing differential of these materials is identified in the Life Cycle Analysis (LCA) as the extraction portion of the processes. These documents also identify Steel Slag as a Co-Product. This classification was determined by the steel industry, which stated that the slag is intentionally co-produced and is used to control chemistry within the hot metal Table 1. Illustrates the production and processing of EAF slag aggregates compared to natural aggregates.

As in all manufacturing processes, the maintenance of a consistent quality feed is essential to the production of a quality product. In the case of steel slag, the quality feed is governed by consistent cooling of the molten material and utilization of the appropriate slag feed for the desired application.
The proper characterization of any material utilized in road construction is essential to ensure proper placement and design. Just as natural aggregates such as limestones or gravels are required to meet certain specifications for road use, EAF steel slag aggregates in many cases must meet the same specifications and occasionally have additional requirements. Steel Slags can be produced to meet various ASTM AASHTO, and FHWA gradation requirements and are highly irregular in shape, typically having 80%+ crush count on two faces or more. They are non-liquid, nonplastic, highly durable with L.A. Abrasion results between 18-30, and historically result in <12% sodium sulfate soundness values.
Steel slags typically have a higher bulk density when compared to other aggregates and the high weight and interlocking properties give them a significant advantage over many aggregates for uses that require stable surfaces and a great bearing capacity. Additionally steel slags exhibit excellent skid-resistant properties in surface course bituminous concrete mixes.
The chemical composition of steel slags can vary depending on the production process or feedstock. For the most part, steel slag products are primarily air cooled and incorporate water sprays to assist in the cooling process. The cooling rate determines the types and quantities of minerals formed from elements that typically include calcium, silica iron, aluminum, magnesium, manganese, phosphorous, and sulfur. These minerals affect the volumetric stability of steel slag, one of the most important characteristics used to evaluate and ensure proper end use applications. Table 2 presents recommendations by the National Slag Association on the application of slag products based on autoclave expansion determined by ASTM D8378 [1].
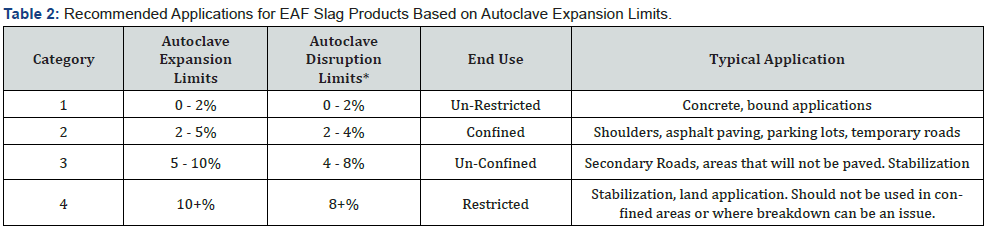
When EAF steel slag is processed properly, it is an excellent option for construction applications, in both bound and unbound applications. The use of slag as aggregates in bound and unbound mixtures particularly in road construction applications as shown in Figure 1 below is very well established. In fact iron and steel slags not only rival similar natural aggregate but in some instances are considered superior materials based on specific properties.
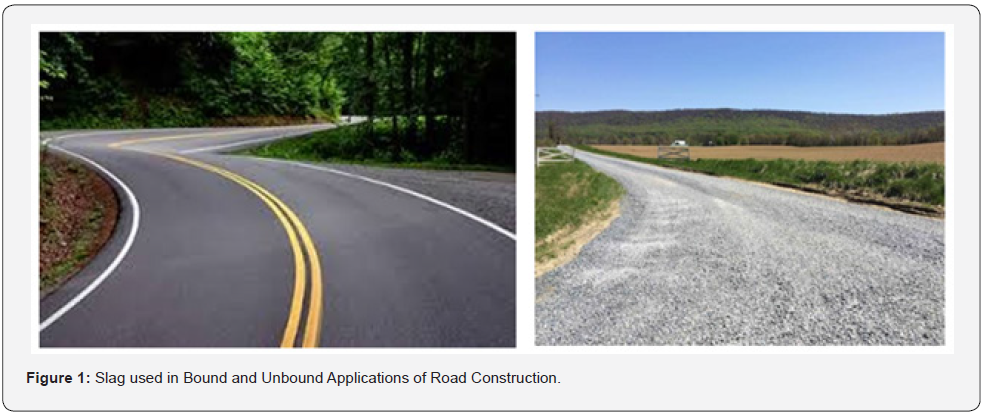
Bound applications One of the most famous uses of EAF slag aggregates in a bound application is in the Indianapolis speedway that used steel slag on its paved surface due to its inherent characteristics. In 2004 engineers were tasked with upgrading the speedway and one of their main challenges was ensuring that the track was constructed in a manner that ensured it could withstand the horizontal sheer stresses generated by cars travelling at over 200 miles per hour into the turns. The objectives were to design a track that had superior durability, was smooth but at the same time provided enhanced friction to ensure the race cars did not skid at the high speeds. The engineers chose a stone matrix asphalt (SMA) and incorporated steel slag as the coarse aggregate [2]. The use of steel slag in this manner has been such a success that today the use of steel slag stone mastic Asphalt (SMA) pavements is now commonplace in regular roads throughout Indiana and Chicago [3]. More recently in 2022 EAF steel slag aggregates were successfully incorporated by the Australian Grand Prix Corporation and Formula 1 onto racetracks in Adelaide and Melbourne Australia [4].
A testament to the durability of EAF steel slag is in its use in an SMA mix at what is known as the “World’s Strongest Intersection”. Thornton, IL is the home to one of the world’s largest limestone quarries and during the construction season ships 50,000 tons of aggregate per day. The only way in or out is through the intersection of Margaret and Williams Streets. Thornton quarry provides most of the mineral aggregates used throughout South Chicago and Northwest Indiana. The vast majority of these aggregates are shipped by truck through the intersection of Margaret and Williams Streets on their way to the marketplace. The intersection must be able to sustain the stresses of high load braking, stopping and turning as it endures around-the-clock pounding of thousands of fully loaded semi-trailers hauling stone and hot mix asphalt to construction sites and to other construction material producers. A forensic analysis of the intersection by Illinois DOT after nearly 20 years of use and nearly 20 million equivalent single axle loads (ESALs) revealed that the SMA mixture maintained the original cross section, continued to provide high friction, maintained stability, reduced fatigue, longitudinal and transverse crack, and maintained essentially the original gradation as installed [5].
Unbound Applications. The Juxtaposition between maintenance costs and lifecycle performance in road building, is a significant challenge facing many counties and townships tasked with addressing their transportation needs. Where costs considerations are paramount, there is a growing movement to convert paved roads into unpaved roads as evidenced by the fact that by 2015, road conversion projects had occurred in 27 states (including Minnesota, Michigan, Alabama, Pennsylvania, and others). Vitale [6] Nevertheless even with the transition from paved roads to unpaved roads, the overall objective does not change namely the construction of cost effective durable roads. This can only be done by utilizing materials or aggregates that inherently have the properties desirable to road engineers as well as county, municipal, and township officials.
An example of the successful use of EAF steel slag aggregates in unbound applications is found in partial depth reclamation and secondary stabilization in Noble County Indiana. Noble County is a mostly rural county with over 1000 miles of county roads maintained. Over the past decade, the county has utilized partial depth reclamation to reduce costs and extend the lifespan of the county roads, maintaining up to 15 miles in a year with this application. This process focuses on drastically improving a roadway’s base strength in contrast to improving the surface through traditional reconstruction techniques. The base is strengthened through the addition of EAF steel slag aggregate containing a specified level of free lime and chemically modified using Calcium Chloride. In this project unconfined compression testing was conducted to determine optimal blending ratios, and the results of the study are shown in Table 3 below.


Noble County typically utilizes a 30-40% blend range depending on the existing roadway and any modifications, such as widening or drainage, are factored into the construction. Reclamation in this case where EAF steel slag aggregate was used involved milling the roadway to a depth of approximately 8 inches, placing 3-4 inches of the slag aggregate product (Duraberm) and then blending these materials together. Overall, the stabilization resulted in vastly improved road structures in a cost-effective manner. Figure 2 depicts a section of the Noble County roadway in this project before reclamation and stabilization and after reclamation and stabilization.
Sustainability
The potential for steel slag to sequester carbon dioxide CO2 is well known and has been investigated extensively [7-12]. Laboratory scale studies utilizing active processes both direct and indirect have shown great promise for steel slag carbon capture and utilization, but most of these processes are far from ready for industrial application [13]. Investigations into the potential for steel slags to capture atmospheric CO2 in the form of stable carbonates in reaction vessels at elevated temperatures (>200 °C) and enriched CO2 gas concentrations (>10%) and pressures (20 bar), 17-21 reveal a theoretical CO2 sequestration capacity of 0.27 to 0.43 kg CO2 per kilogram of slag [14]. In the 2018 study by Mayes et al., the researchers examined atmospheric carbon dioxide sequestration within a greater than 20 million ton legacy deposit and determined that less than 1% of the maximum carbon-capture potential of the deposit had been realized. The poor conversion of the carbon capture potential was attributed to limited ingress of atmospheric CO2 into the slag bank [14].
After slag aggregates have been processed and are stockpiled prior to shipping, there is the potential for limited CO2 sequestration to occur within these piles due to the inability to penetrate deep into the piles. However, after shipment and placement in unencapsulated/unconfined applications such as in secondary unpaved roads, the passive sequestration of atmospheric carbon dioxide can be more effectively realized due to the increased surface area that would be exposed in this application. When you consider that in the United States alone the length of unpaved roadways can range anywhere from 1.6 to 2.1 million miles [15,16] the potential to passively sequester CO2 from the atmosphere is quite large if EAF slag aggregate was used for this application. Figure 3 below illustrates the cross section of a typical gravel road [17]. The road would be approximately 20 feet wide and have a gravel thickness of approximately 8 inches. With this design the sequestration potential of EAF slag aggregate can be more successfully exploited since the ingress limitation previously discussed would not exist. The US iron and steel industry produces approximately 17 million tons of EAF steel slag aggregate that can be potentially used for unpaved roadways which would be less than 1% of unpaved roadway coverage. Nevertheless, assuming a 0.3-ton CO2 sequestration capacity per ton of EAF slag aggregate [14] this minimal footprint would amount to a potential of 5.1 million tons of CO2 being removed from the atmosphere.
Conclusion
EAF steel slag aggregates utilized in road construction applications are an excellent alternative to natural aggregate utilized in the same manner. In paved roadways specifically with bituminous mixes these products improve the mechanical performance and durability as well as the long-term stability of roads. Tests have shown that in rutting, fatigue, skid resistance, creep and resilient modulus tests, the incorporation of steel slag into a stone matrix asphalt (SMA) mixture wearing course outperforms most conventional wearing courses. In properly designed unbound applications EAF steel slag aggregates are a cost effective and sustainable alternative to traditional materials providing the strength and durability required in this application. Probably more significant though is their potential to sequester atmospheric CO2. The ability to capture anywhere from 30 to 40 % of their weight in CO2 makes them an attractive alternative to conventional aggregates.
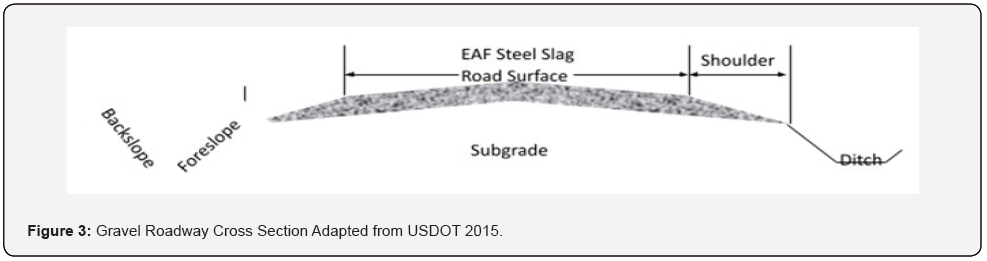
References
- ASTM International (2021) Standard Test Method for Potential Expansion of Steel Slag from Hydration Reactions by Autoclave (D8378/D8378M-21). West Conshohocken, PA, USA.
- NAPA (2005) Indianapolis Motor Speedway Gets a Paving Face Lift. Hot Mix Asphalt Technology 10(1): 12-22.
- American Chemical Society, Discover Chemistry (2013) INDY 500 Track continues to foster better technology for everyday driving.
- ASA (2023) Harsco Environmental Supports Australian Racetrack Projects.
- Wayne J, Timothy M (2018) World’s Strongest Intersection Leaves Teenage Years Behind Asphalt 33(2): 13-20.
- Vitale R (2021) The Key to Successfully Converting Paved to Unpaved Roads Gravel Roads, Road & Surface Management, Roads, Unpaved Roads.
- Wouter JJH, Geert JW, Comans RNJ (2005) Mineral CO2 Sequestration by Steel Slag Carbonation, Environmental Science & Technology 39(24): 9676-9682.
- Bodor M, Santos RM, Kriskova L, Elsen J, Vlad M, et al. (2013) Susceptibility of mineral phases of steel slags towards carbonation: mineralogical, morphological, and chemical assessment, European Journal of Mineralogy 25(4): 533-549.
- Shu YP, Rahul A, Yi HC, Ping L, Pen CC (2016) Integrated and innovative steel slag utilization for iron reclamation, green material production and CO2 fixation via accelerated carbonation. Journal of Cleaner Production 137: 617-631.
- Rushendra RTD, Palanivelu K, Ramachandran A (2016) Direct mineral carbonation of steelmaking slag for CO2 sequestration at room temperature. Environmental Science and Pollution Research 23(8): 7349-7359.
- Corey AM, Takao N, Kosei A (2019) Quantification of the CO2 mineralization potential of ironmaking and steelmaking slags under direct gas-solid reactions in flue gas, International Journal of Greenhouse Gas Control 87: 100-111.
- Shu YP, Yi HC, Liang SF, Hyunook K, Xiang G, et al. (2020) CO2 mineralization and utilization by alkaline solid wastes for potential carbon reduction. Nature Sustainability, Nature 3(5): 399-405.
- Qing Z, Chengjun L, Xiaohui M, Henrik S, Ron Z (2022) Research progress of steel slag-based carbon sequestration. Fundamental Research.
- William MM, Alex LR, Helena IG, Peter B, Joanna H, et al. (2018) Environmental Science & Technology 52(14): 7892-7900.
- United States Department of Transportation (USDOT) Federal Highway Administration (FHWA) (2017) Public Road Length Miles by Functional System.
- Center for Safety Equity in Transportation (CSET) (2019) Operational Safety of Gravel Roads in Rural and Tribal Communities: Vulnerability to Structural Failures and Geo-Hazards: Final Project Report.
- United States Department of Transportation (USDOT) Federal Highway Administration (FHWA) (2015) Gravel Roads Construction and Maintenance Guide FHWA Publication No: FHWA OTS-15-0002.