- Review Article
- Abstract
- Colon Cancer
- Colon Cancer Epidemiology
- Colon Cancer Diagnosis
- Colon Cancer Signs and Symptoms
- Stages of Colon Cancer
- Colon Cancer Risk Factors
- Prognosis
- Signaling Pathways Involved in Colon Cancer Angiogenesis and Progression
- Colon Cancer Treatment
- Role of Immune System in Colon Cancer
- Conclusion and Future directions
- References
Colon Cancer, Angiogenesis and Therapy
Bou Hamdan M*
Department of Biological Sciences, Faculty of Science, Beirut Arab University, Debbieh, Lebanon; Medical Laboratory Sciences Program, Division of Health Professions, Faculty of Health Sciences, American University of Beirut, Beirut, Lebanon
Submission: September 16, 2021; Published: October 20, 2021
*Corresponding author: Bou Hamdan M, Department of Biological Sciences, Faculty of Science, Beirut Arab University, Debbieh, Lebanon; Medical Laboratory Sciences Program, Division of Health Professions, Faculty of Health Sciences, American University of Beirut, Beirut, Lebanon
How to cite this article: Bou Hamdan M. Colon Cancer, Angiogenesis and Therapy. Ann Rev Resear. 2021; 6(5): 555700. DOI: 10.19080/ARR.2021.06.555700
- Review Article
- Abstract
- Colon Cancer
- Colon Cancer Epidemiology
- Colon Cancer Diagnosis
- Colon Cancer Signs and Symptoms
- Stages of Colon Cancer
- Colon Cancer Risk Factors
- Prognosis
- Signaling Pathways Involved in Colon Cancer Angiogenesis and Progression
- Colon Cancer Treatment
- Role of Immune System in Colon Cancer
- Conclusion and Future directions
- References
Abstract
Colon cancer is the fourth most leading causes of cancer-related deaths worldwide accounting for nearly 7-10% of all cancers. Although, screening methods aided in the early detection of colon cancer and thus in the prevention of cancer-related deaths, several factors result in the prognosis of colon cancer such as stage of disease, site of metastasis, type of treatment given and tumor genetic mutations. Various signaling pathways have been implicated in colon cancer progression and thus, they were the targets of multiple anticancer therapeutic agents. There are many types of therapeutic agents approved or discovered for colon cancer treatment. These include surgery, chemotherapy, immunotherapy, and others. Immunotherapy is the most advanced type of colon cancer treatment, and it includes multiple subtypes. In conclusion, this review presents almost all of the available data in relation to colon cancer disease-related diagnosis, prognosis, treatment, and angiogenic signaling pathways. The presented data can be further utilized by scientists to build upon in their future research studies.
Keywords: Capecitabine; Xeloda; 5-fluorouracil; Irinotecan; Bevacizumab
Abbreviations: CRC: Colorectal cancer; FS: Flexible sigmoidoscopy; CTC: Computed tomographic colonography; CEA: Carcinoembryonic antigen; TPS: Tissue polypeptide specific antigen; HGFs: Hematopoietic growth factors; MAC: Modified Astler-Coller; NSAIDs: Non-steroidal anti-inflammatory drugs; ARIC: Atherosclerosis Risk in Communities; FAP: Familiar adenomatous polyposis; APC: adenomatous polyposis coli; HNPCC: Hereditary nonpolyposis colorectal cancer; NAFLD: Non-alcoholic fatty liver disease; CEA: Carcinoembryonic antigen; VEGF: Vascular endothelial growth factor; PDGF: Platelet-derived growth factor; FGF: Fibroblast growth factor; TGF: Tumor growth factor beta; NSCLC: Non-small cell lung cancer; MAPK: Mitogen-activated protein kinases; ERK: Extracellular signal-regulated kinase; EGFR: Epidermal growth factor receptor; RTKs: Receptor tyrosine kinases; CRC: Colorectal cancer; APC: Adenomatous polyposis coli; EMT: Epithelial-mesenchymal transition; MMPs: Matrix metalloproteinases; SHH: Sonic Hedgehog; TS: Thymidylate synthase; FdUTP: Fluorodeoxyuridine triphosphosphate; PDGFR: Platelet-derived growth factor receptor; RCC: Renal cell carcinoma; HCC: Hepatocellular cell cancer; TKI: Tyrosine kinase inhibitor; RAF: Rapidly accelerated fibrosarcoma; KIT: Tyrosine kinase type III; RET: Rearranged during Transfection; FGFRs: Fibroblast growth factor receptors; GISTs: Gastrointestinal stromal tumors; FDA: Food and Drug Administration; NK: Natural killer; APCs: Antigen-presenting cells; STING: Stimulator of interferon genes; ACT: Adoptive cellular therapy; CARs: Chimeric antigen receptors; MHCs: Major histocompatibility complexes; TNFR: Tumor necrosis factor receptor; JNK: c-Jun N-terminal Kinases; IBD; Inflammatory bowel disease
- Review Article
- Abstract
- Colon Cancer
- Colon Cancer Epidemiology
- Colon Cancer Diagnosis
- Colon Cancer Signs and Symptoms
- Stages of Colon Cancer
- Colon Cancer Risk Factors
- Prognosis
- Signaling Pathways Involved in Colon Cancer Angiogenesis and Progression
- Colon Cancer Treatment
- Role of Immune System in Colon Cancer
- Conclusion and Future directions
- References
Colon Cancer
Colon cancer is considered the fourth most leading causes of cancer-related deaths worldwide [1,2], second most common cancer in females, third in males, and the third worldwide [3] accounting for nearly 7-10% of all cancers [4,5]. In USA, colon cancer was the third leading cause of cancer-related deaths as it is considered a significant resistant to the main treatment methods [6]. The causes of this disease involve an interaction between both environmental and genetic factors. The process of colon cancer development requires several steps: the latter aid in the progression of benign colorectal adenoma to malignant carcinoma. Factors involved in this progression include accumulation of chromosomal abnormalities, genetic mutations, and epigenetic changes leading to the inhibition of tumor suppressor genes and DNA mismatch repair genes or the stimulation of oncogenes [7,8].
Polyp histology is critical for the determination of the malignant potential of colorectal cancer (CRC) [9]. The two common types of colon cancer include the hyperplastic and the adenomatous polyps. Hyperplastic polyps usually contain large number of glandular cells with small amount of mucus in the cytoplasm, in addition to that, they mostly lack nuclear hyperchromatism, stratification, or atypia.
However, adenomatous nuclei are generally hyperchromatic, large, cigar-shaped, and clustered together to form a palisade pattern. Adenomas are classified as tubular or villous [9]. These 2 types of polyps were reported to be the originators of hereditary and sporadic types of colorectal cancer [10]. Therefore, the frequency of colorectal polyps and the prevalence of colorectal cancer has been increasing worldwide [11]. It was previously reported in a cross-sectional study that the high serum insulin levels resulted in an increased prevalence of adenomatous and hyperplastic polyps among 343 CRC patients who did colonoscopy. Moreover, further studies conducted among the general population also reported the role of hyperplastic polyps as markers for the development of adenomas [12-15]. Some studies also reported the correlation between hyperplastic polyps and colon cancer [9]. Therefore, colon cancer is characterized by the development of adenomatous polyps and malignant cells in the colon; the latter are known to undergo uncontrolled replication and metastasize. Molecular changes may also result in colon cancer development. These include mutations in Kirsten-ras, p53, and adenomatous polyposis coli [7,8]. Several factors result in the prognosis of colon cancer such as stage of disease, site of metastasis, type of treatment given and tumor genetic mutations [16].
- Review Article
- Abstract
- Colon Cancer
- Colon Cancer Epidemiology
- Colon Cancer Diagnosis
- Colon Cancer Signs and Symptoms
- Stages of Colon Cancer
- Colon Cancer Risk Factors
- Prognosis
- Signaling Pathways Involved in Colon Cancer Angiogenesis and Progression
- Colon Cancer Treatment
- Role of Immune System in Colon Cancer
- Conclusion and Future directions
- References
Colon Cancer Epidemiology
CRC is one of the leading causes of cancer mortality in the United States [3,4,6], the second most common cancer in Europe [16], and the fourth most common cancer worldwide [17]. The incidence of colon cancer is 20% higher among males as compared to females [18]. In 2020, the American Cancer Society estimated the number of newly diagnosed patients with colon cancer to 104,610 in the US. They also estimated the number of deaths in the US due to colon cancer to be 53,200 including 3,640 men and women who are younger than 50 years old [18]. In contrast, colon cancer estimated new cases in the US were 97,220 in 2018, 49,690 males and 47,530 females, and the estimated number of deaths were 50.630 [4]. To our interest, the estimated number of deaths in 2020 and 2018 was higher than that encountered in 2017 (50, 260 deaths) [19]. It is also important to note that further to the evolution of screening methods in the period of time between 2004 and 2013, the colon cancer incidence rates were able to decrease 3% per year [19].
The risk of CRC increases with age [18].
The median survival of patients with metastatic colorectal carcinoma and are not responding to treatment and surgery surgery was reported to be less than 3 years [16,19]. Birenda et al. reported an increased survival rate of around 5 years among 20-40% of those whose colon was resected [19]. Early- Onset Colorectal cancer (EoCRC) was reported among people between 20 and 39 years of age in the US between 2013 and 2018. The collected data showed that EoCRC was found to occur predominantly in the Caucasian and female population with an overall rate of 18.9/100000 [20]. The most commonly occurring colorectal cancer is the sporadic type rather than the inherited (5% of cases) one [21]. Various studies were conducted on the incidence of colon cancer among migrating individuals. The former reported a higher incidence of colorectal cancer among populations who were translocated from an area of low disease risk (e.g. Japan) to an area of high disease risk (e.g. USA). For instance, Japanese people who were born in the US were at a higher risk than the white population to develop CRC [22].
- Review Article
- Abstract
- Colon Cancer
- Colon Cancer Epidemiology
- Colon Cancer Diagnosis
- Colon Cancer Signs and Symptoms
- Stages of Colon Cancer
- Colon Cancer Risk Factors
- Prognosis
- Signaling Pathways Involved in Colon Cancer Angiogenesis and Progression
- Colon Cancer Treatment
- Role of Immune System in Colon Cancer
- Conclusion and Future directions
- References
Colon Cancer Diagnosis
Screening methods aided in the early detection of colon cancer and thus in the prevention of cancer-related deaths. Colon cancer awareness campaigns have advised adults and recommended a regular colon cancer screening for adults who are 50 years and above since they are considered at higher risk of developing CRC [23]. Current colorectal cancer screening methods are divided into invasive and noninvasive methods. Invasive tests include the flexible sigmoidoscopy (FS) and colonoscopy. These methods can detect the presence of polyps in the colon through visualizing the pathological sample. Colonoscopy was considered as the primary screening tool in various countries worldwide. The noninvasive tests include stool-based tests (guaiac fecal occult blood test, fecal immunochemical test and fecal DNA testing), blood-based tests (Epi procolon for the PCR detection of mutated methylated septin9 DNA) and radiological tests (double contrast barium enema, capsule endoscopy and Computed tomographic colonography (CTC)) [5]. Molecular tests are known to be better than the current screening methods in terms of sensitivity and specificity especially since they provide specific details in relation to the tumor progression. Research is still ongoing to identify and predict noninvasive biomarkers that might aid in early CRC diagnosis using blood and stool samples [24]. Moreover, researchers are still searching for serological markers that might aid in the early detection and diagnosis of colorectal cancer. Although several potential tumor markers (proteins, glycoproteins and cellular and humoral substances) have been studied, none of these has been reported to be specific for colorectal cancer diagnosis [22]. To date, the most widely studied marker is carcinoembryonic antigen (CEA) [25]; the latter was reported to be beneficial for following-up on patients with large bowel cancer before and after undergoing surgery however, it was less sensitive and specific in the diagnosing the disease among asymptomatic patients [22]. It was also reported that new carbohydrate antigens such as CA19-9 along with CEA were the most common markers for colon cancer diagnosis prior to and after undergoing surgery [26]. Moreover, several biochemical markers have been reported to aid in colorectal cancer diagnosis including tissue polypeptide specific antigen (TPS), tumor-associated glycoprotein-72 (TAG- 72), hematopoietic growth factors (HGFs), macrophage-colony stimulating factor (M-CSF), granulocyte-macrophage-colony stimulating factor (GM-CSF), interleukin-3, interleukin-6, and some enzymes such as alcohol dehydrogenase and lysosomal exoglycosidases. However, none of these showed the required accuracy and specificity. Therefore, it was reported that the best markers to analyze during monitoring colorectal cancer treatment should be the metastasizing cancer cells [27]. Another recent study used autoantibodies and was able to report a panel of markers that can be used to differentiate colon cancer patients from healthy individuals. These markers included p53, ERP44, TALDO1, CHCHD3, CTTNBP2NL, FKBP4, MGST3, THSD7A, and TRIM29 [28].
- Review Article
- Abstract
- Colon Cancer
- Colon Cancer Epidemiology
- Colon Cancer Diagnosis
- Colon Cancer Signs and Symptoms
- Stages of Colon Cancer
- Colon Cancer Risk Factors
- Prognosis
- Signaling Pathways Involved in Colon Cancer Angiogenesis and Progression
- Colon Cancer Treatment
- Role of Immune System in Colon Cancer
- Conclusion and Future directions
- References
Colon Cancer Signs and Symptoms
Colon cancer symptoms include bloody stool, tiredness, weight loss, and changes in the bowel movement such as diarrhea and constipation [2]. Signs start to become obvious at later stages of the disease [9]. Moreover, Glover et al. have shown some symptoms in patients with EoCRC that include hematochezia, anemia, decreased appetite along with higher prevalence rates of medical comorbidities such as diabetes and obesity [20]. Moreover, other signs and symptoms may appear including hypoalbuminemia, peripheral edema, ascites, anasarca, hypoactive or high-pitched bowel sounds, and gastrointestinal obstructions. Some rare findings may also appear including palpable abdominal mass, palpable Virchow’s lymph node in the left supraclavicular space, hepatomegaly from hepatic metastases, and temporal or intercostal muscle wasting from cancer cachexia [9].
- Review Article
- Abstract
- Colon Cancer
- Colon Cancer Epidemiology
- Colon Cancer Diagnosis
- Colon Cancer Signs and Symptoms
- Stages of Colon Cancer
- Colon Cancer Risk Factors
- Prognosis
- Signaling Pathways Involved in Colon Cancer Angiogenesis and Progression
- Colon Cancer Treatment
- Role of Immune System in Colon Cancer
- Conclusion and Future directions
- References
Stages of Colon Cancer
The older Dukes or the Modified Astler-Coller (MAC) classification schema reported that stages should be defined by the TNM classification [22,29]. TNM signifies a dual system that consists of both clinical (pretreatment) and pathological (postsurgical histopathological) classification. The clinical classification is referred to as cTNM while the pathological is termed pTNM [22,29]. Early-stage colon cancer can be defined as the localized type of the disease that has no evidence of reaching the adjacent organs, lymph nodes or distant sites [30]. This type of localized disease is at higher risk for developing colon polyps and metachronous colon cancer signifying the importance of using screening tools to manage the disease at early stages [30]. Colon cancer staging is defined as such: Stage 0 when the tumor is confined to the mucosa; Stage I may be equivalent to Dukes’ A or MAC A or B whereby the tumor is limited to bowel wall (mucosa, muscularis mucosae, submucosa, and muscularis propria); Stage II may be equivalent to Dukes’ B or MAC B2 or B3 whereby tumor has spread to extramural tissue; Stage III may be equivalent to Dukes’ C or MAC C1-C3 whereby tumor metastases to regional nodes; and Stage IV when tumor metastasizes to distant body sites [22,30]. Therefore, defining the stage of CRC disease accurately and precisely is essential in order to assess the requirement for treatment and for surveillance reporting [30].
- Review Article
- Abstract
- Colon Cancer
- Colon Cancer Epidemiology
- Colon Cancer Diagnosis
- Colon Cancer Signs and Symptoms
- Stages of Colon Cancer
- Colon Cancer Risk Factors
- Prognosis
- Signaling Pathways Involved in Colon Cancer Angiogenesis and Progression
- Colon Cancer Treatment
- Role of Immune System in Colon Cancer
- Conclusion and Future directions
- References
Colon Cancer Risk Factors
It is highly essential to identify the risk factors associated with colorectal cancer and screen the population for the presence of colonic polyps to decrease colon cancer progression and raise awareness among the general population [31]. Risk factors to colon cancer are many. These include smoking, inappropriate diet, alcohol, old age, inflammatory factors like inflammatory bowel disease, lack of physical activity, diabetes, and inherited syndromes like familial adenomatous polyposis and hereditary non-polyposis colorectal cancer (Lynch syndrome) [32]. Historically, diet was one of the most important factors contributing to colorectal cancer progression. Interestingly, it was reported by the World Cancer Research Fund and the American Institute for Cancer Research that colorectal cancer is mostly preventable by appropriate diets and associated factors [33]. It was also reported that red meat, processed meat, substantial consumption of alcoholic drinks, body fatness and abdominal fatness, and greater adult height related factors are major risk factors of colorectal cancer. On the other hand, foods containing dietary fiber, folate, selenium, calcium, or vitamin D as well as garlic, non-starchy vegetables, fruits, and fish are probably protective against colorectal cancer [32,34]. Moreover, several studies reported on the effect of physical activity in protecting against colorectal cancer [34]. Moreover, non-dietary factors have been reported to increase the risk of colon cancer occurrence. These include smoking tobacco, chronic use of non-steroidal anti-inflammatory drugs (NSAIDs), aspirin, genetic predispositions, and metabolic syndromes [35]. A positive correlation between smoking and large colorectal adenomas leading to colorectal cancer was reported. Thus, being exposed to tobacco components can be a contributing factor to the initiation of colorectal cancer [36].
A systematic review has reported the effect of nonsteroidal anti-inflammatory drugs in preventing or decreasing colon cancer progression. The former showed that three randomized trials reported the significant role of aspirin in decreasing the recurrence of sporadic type pf colon cancer polyps, specifically adenomatous polyps. However, it was not supportive in inhibiting or preventing the development of these polyps in the familial type of adematous [22,37]. Interestingly, inflammatory bowel diseases, Crohn’s disease and ulcerative colitis, are risk factors for colon cancer. It was reported there is an increased risk of colon cancer occurrence among people who have Crohn’s disease [38]. Moreover, a study by Eaden et al. reported a positive correlation between ulcerative colitis and colorectal cancer [39]. Patients who have other types of cancer are also at higher risk to develop CRC. The metabolic syndrome (≥3 of the following components: high blood pressure, increased waist circumference, hypertriglyceridemia, low levels of high-density lipoprotein cholesterol, or diabetes/hyperglycemia) has been reported by the Atherosclerosis Risk in Communities (ARIC) to be a risk factor for colorectal cancer occurrence among men [40]. Moreover, postmenopausal estrogen and/ or progesterone hormone use have been associated with lowere incidence of colon cancer development [41].
Genetic predisposing factors to colon cancer resulted in either polyposis or nonpolyposis syndromes. Among these, the main polyposis syndrome is familiar adenomatous polyposis (FAP); the latter is characterized by mutation or loss of FAP (also called the adenomatous polyposis coli (APC)) gene. Hereditary nonpolyposis colorectal cancer (often referred to as HNPCC) syndrome is related to germline mutations in six DNA mismatch repair genes [35]. Non-alcoholic fatty liver disease (NAFLD) is one of the most common metabolic disease of the liver worldwide with an increased prevalence in Asian and Western countries [42].
Interestingly, higher levels of insulin, insulin resistance status and chronic inflammation conditions were noticed among NAFLD patients [43-45]. It has been proposed that insulin activates colorectal cell proliferation and increases bioactive insulin growth factor-1 (IGF-1); the latter activates cell cycle progression via inducing mitogenic and antiapoptotic effects [46]. Several studies suggested that NAFLD was associated with an increased risk for colorectal adenomas and advanced neoplasms [47-49]. Hwang et al. suggested via a study conducted on 2917 subjects (556 adenomatous polyps and 2361 polyp-free) that NAFLD was a significant independent risk factor for colorectal adenoma [48]. In addition, in a retrospective observational study, Touzin et al. investigated the relationship between biopsy-proven NAFLD and colorectal adenoma however, they were unable to prove that patients with biopsy-proven NAFLD had an increased prevalence of colorectal cancer [50]. Therefore, the correlation between NAFLD and colorectal polyps may be controversial.
- Review Article
- Abstract
- Colon Cancer
- Colon Cancer Epidemiology
- Colon Cancer Diagnosis
- Colon Cancer Signs and Symptoms
- Stages of Colon Cancer
- Colon Cancer Risk Factors
- Prognosis
- Signaling Pathways Involved in Colon Cancer Angiogenesis and Progression
- Colon Cancer Treatment
- Role of Immune System in Colon Cancer
- Conclusion and Future directions
- References
Prognosis
The prognosis of colon cancer is proportional to the degree of tumor penetration through the bowel wall and to the adjacent lymph nodes. Additional factors contributing to disease prognosis include grading, angio- or venous-invasion and perineural invasion, lymphoid inflammatory response and tumor involvement of resection margins [22,51]. Moreover, the degree and number of node involvement is considered as a prognostic factor [51]. Several other prognostic factors also exist including p53, KRAS, BRAF, bcl- 2, B-catenin, c-MET, TGF-alpha, EGF, and others [52-58]. Tumor location was reported to be a strong prognostic CRC factor [59]. For instance, node involvement was reported to higher among right colon tumors as compared to the left ones and therefore, left colon tumor location had better prognosis [59]. Bowel obstruction was also correlated with disease prognosis affected by the tumor location. Similarly, bowel obstruction in the right side resulted in poor prognosis and less ability to survive disease free as compared to obstructions that occur at the left colon side [22,60]. Also, perforation is a clinical indicator of a poor prognosis [61]. High serum levels of carcinoembryonic antigen (CEA) and of carbohydrate antigen 19-9 (CA 19-9) have been associated with poor prognosis [26].
- Review Article
- Abstract
- Colon Cancer
- Colon Cancer Epidemiology
- Colon Cancer Diagnosis
- Colon Cancer Signs and Symptoms
- Stages of Colon Cancer
- Colon Cancer Risk Factors
- Prognosis
- Signaling Pathways Involved in Colon Cancer Angiogenesis and Progression
- Colon Cancer Treatment
- Role of Immune System in Colon Cancer
- Conclusion and Future directions
- References
Signaling Pathways Involved in Colon Cancer Angiogenesis and Progression
Angiogenesis is defined as the equilibrium between pro- and antiangiogenic factors and between multiple signaling pathways in the body. Once cancer is initiated, the need of nutrient supply by tumor cells will cause a disruption in this equilibrium known as “angiogenic switch”; the latter is critically involved in the development and spread of CRC. Moreover, tumor growth and development will cause limited oxygen distribution and thus, tumors will rely on intrinsic blood supply. Proangiogenic proteins determine the proliferation of endothelial cells and the growth of the tumor vasculature. Members of the VEGF (vascular endothelial growth factor) family and their receptors have been recognized as facilitators of angiogenesis. The binding of VEGF to their receptors situated on endothelial cells induces chain reactions that are mostly mediated by MAP (mitogen-activated protein) kinase and PI3K/Akt/mTOR. During tumor progression, major angiogenic players include the vascular endothelial growth factor (VEGF), the platelet-derived growth factor (PDGF), the FGF (fibroblast growth factor), and the tumor growth factor beta (TGF𝛽) [24].
The VEGF/VEGFR Pathway involves vascular endothelial growth factor (VEGF) signaling molecule and its cognate receptor (VEGF receptor 2 [VEGFR-2]). It is the most important angiogenetic pathway involved in the formation and branching of new tumor blood vessels, the development of rapid tumor growth, and the metastatic potential of tumor cells [62,63]. VEGF-A and VEGF-B have a significant role in angiogenesis through binding to their corresponding tyrosine kinase receptors (VEGFR-1 and VEGFR-2). Upon binding of VEGF ligand to VEGFRs, the latter will be activated via phosphorylation. The phosphorylation of VEGFR will result in the activation of K-RAS (Kirsten rat sarcoma viral oncogene homolog) which in turn activates 2 different pathways the KRAS/BRAF/MEK/ERK pathway and the PI3K/ AKT/mTOR pathway leading to tumor cell progression, growth and proliferation [64,65].
i. The PI3K/Akt/mTOR pathway is one of the important pathways involved in tumor development and progression [66]. The product of phosphoinositide 3-kinase (PI3K) activity is one of the important factors required for the activation of cellular growth and malignant transformation [67]. Moreover, it was reported that high PI3K level can lead to tumor formation and therefore, it is considered an essential trait in human cancer [67]. The mammalian target of rapamycin (mTOR), also known as the mechanistic target of rapamycin, was discovered, and shown to combine signals from nutrients and PI3K [67]. Studies showed the important roles for this complex pathway in contributing to growth control of cells and organs. Studies that involve mouse genetic models has shown the importance of having balance in the signaling PI3K pathways and responses for proper immune cell development and responses against invading pathogens [67]. During the past three decades, research showed that the PI3K family members participate in a wide range of processes involved in cellular regulation, including cell growth and proliferation, metabolism, migration, and secretion (Figure 1).
Moreover, abnormalities or dysregulations in PI3K signaling pathway result in multiple human diseases, such as cancer, immunological disorders, neurological disorders, diabetes, localized tissue overgrowth and cardiovascular disease [67]. It was reported that various activating mutations of the catalytic class I isoform of PI3K (phosphatidylinositol-4,5-bisphosphate 3-kinase catalytic subunit alpha, referred to as PIK3CA), have been identified in different types of human tumors [67,68]. Sub-clonal mutations in PIK3CA were reported in several cancer subtypes, including non-small cell lung cancer (NSCLC), colorectal cancer, breast cancer, and others [69-72]. Interestingly, it was also reported that mutations in the PI3K-AKT-mTOR pathway were more prevalent than mutations in the RAS-MAPK pathway although the latter was known to be expressed in almost all types of tumor subclones [66,68,70]. Mutations in other PI3K genes were rare in the tumor environment. PI3K signaling was reported to be abnormally activated in the majority of human cancers and therefore PI3K/mTOR pathway inhibitors were reported to play a major role in cancer therapy [66,67].
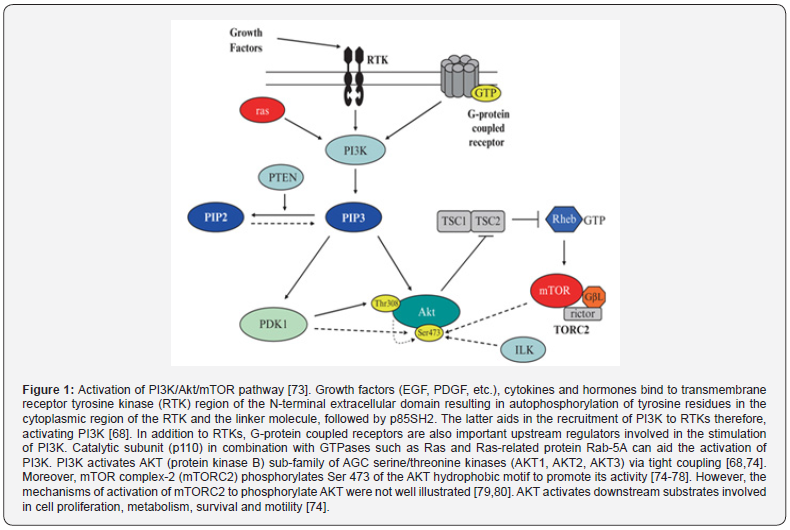
ii. The MAPK/ERK pathway, also known as the Ras-Raf- MEK-ERK pathway, is a signaling pathway that involves many proteins such as mitogen-activated protein kinases (MAPK), originally called ERK. Activating and inhibitory signals among this pathway occur via phosphorylation of a neighboring protein in a multistep process [81,82]. MAPKs are a family of serine/threonine protein kinases implicated in different cellular processes including proliferation, differentiation, motility, stress response, apoptosis, and survival. The most common MAPK families involved in this pathway include extracellular signal-regulated kinase (ERK), Jun kinase (JNK/SAPK) and p38 MAPK. Activated Ras activates the protein kinase activity of RAF kinase. RAF kinase phosphorylates and activates MEK (MEK1 and MEK2). MEK phosphorylates and activates ERK [81,82]. Various therapeutic agents were used to specifically target this pathway in cancer treatment [83]. Raf kinases are serine/threonine kinases in the Raf/ mitogen extracellular kinase (MEK)/extracellular signal-related kinase (ERK) signaling pathway involved in regulating cellular proliferation and survival. Raf kinase isoforms (wild-type Raf-1 or the b-raf V600E oncogene) are over-expressed in a variety of solid tumor types, [84]. Raf-1 is involved in increasing cell survival via inhibiting apoptosis; the latter is achieved via various mechanisms involving its translocation to the mitochondria.
Dysregulation of this process was reported to be present in various tumors and interfering in tumor formation and resistance to chemotherapeutic agents. Activation of the intrinsic apoptotic pathway disrupts the mitochondrial membrane potential, leading to apoptosome stimulation and activation of the terminal caspase cascade therefore, activation of cell death and apoptosis. Mitochondrial release of cytochrome c involved in apoptotic pathway is regulated by other factors such as apoptosis signalregulated kinase [ASK-1], Bcl-2 antagonist of cell death [BAD]), Bcl-2 and Mcl-1. Raf-1 phosphorylates and inactivates BAD and activates Bcl-2 to prevent cytochrome c release and prolong cell survival. Interestingly, this direct anti-apoptotic effect of Raf- 1 is independent of MEK/ERK. Raf-1 also directly inhibits the degradation of the anti-apoptotic protein Mcl-1 to prolong cell survival in cancer cells and can directly inhibit the proapoptotic protein ASK-1 by a MEK/ERK-independent mechanism [85,86]. Raf-1 has also shown to inhibit mammalian sterile 20-like kinase 2 (MST2) involved in apoptosis by a direct protein–protein interaction [87,88]. In vivo studies have shown that Raf-1 has been implicated in the onset and progression (angiogenesis, invasiveness, and metastatic spread) of solid tumors [84]. The abnormal increase in wild-type Raf-1 activity is common in solid tumors and may be due to several factors including the stimulation of Raf-1 by oncogenic Ras in solid tumors.
The Ras proto-oncogene family, which includes N-Ras (neuroblastoma cell line), H-Ras (Harvey murine sarcoma virus), and K-Ras (Kirsten murine sarcoma virus), has played an essential role in both normal cellular function and cancer. Oncogenic ras mutations or increased levels of expression of wild-type Ras were reported to result in DNA synthesis and therefore, malignant transformation, increased cell survival, and invasion/metastasis [84]. The most common ras gene mutations seen in human cancer include point mutations at codons 12, 13, or 61 of the kras gene [89]. Oncogenic k-ras mutations have been reported in pancreatic carcinomas whereby inhibition of k-ras in pancreatic tumor cells (Panc-1 and Mia-PaCa2) by RNA interference (RNAi) resulted in a decrease in the malignant transformation of those cells [90]. It was also reported that the expression of k-ras in pancreatic cancer was correlated with poor disease prognosis [91]. Oncogenic k-ras mutations was also shown to be related to colon cancer disease and cancer invasiveness in vitro, and therefore, poor disease prognosis [92,93]. An abnormal overexpression of Raf-1 and its increased activity can be due to certain mutations in oncogenes occurring in upstream RTKs [89]. Increased expression levels and activity of Raf-1 due to an increase in growth factor receptors were reported in some tumors such as NSCLC (non-small cell lung cancer), RCC (renal cell cancer), and HCC (hepatocellular carcinoma) [84,89].
iii. The epidermal growth factor receptor (EGFR) is a member of the ErbB family of receptor tyrosine kinases (RTKs). It plays an important role in epithelial cell physiology. It was reported to be mutated or highly expressed various types of human cancers therefore, being a potential target of cancer therapies [94]. Upon binding to its ligand, EGFR forms a dimer and resulting in structural rearrangements in the cytoplasmic domain; the latter would result in the activation of the signaling cascade due to the formation of a dimer between the two catalytic domains, the activation of the EGFR kinase, and trans-autophosphorylation of critical tyrosine residues in the cytoplasmic receptor tail. This ligand-dependent stimulation of EGFR would further stimulate various downstream signaling pathways including the Ras/MAPK pathway, the PI3K/AKT pathway, and the phospholipase C (PLC)/ protein kinase C (PKC) signaling cascade. EGFR signaling has been involved in cell survival, proliferation, differentiation, and motility [94].
The PDGF/PDGFR pathway is one of the important pathways implicated in CRC angiogenesis and cancer progression. Cellular effects because of this pathway are due to the binding between the PDGF isoforms (PDGF-A, PDGF-B, PDGF-C, and PDGF-D) and their corresponding homodimeric and heterodimeric PDGF receptors. All PDGF ligands except PDGF-DD can stimulated homodimeric PDGFR-𝛼𝛼 whereas all PDGF isoforms except PDGF-AA can activate the heterodimeric PDGFR-𝛼𝛽. Interestingly, only PDGFBB and PDGFDD can stimulate PDGFR-𝛽𝛽. The activated receptors will result in the formation of the complex Ras/MAP-kinase signaling cascade via the activation of downstream pathways such as PI3K/AKT/mTOR, JNK, GAP, and STATs pathways [24]. PDGFR/ PDGF system has been implicated in vital mechanisms including growth of mesenchymal cells, embryogenesis, and tissue repair and wound healing in adults [24]. In normal circumstances and during development, PDGFR-α activates the pathway needed for the development of the lung, intestinal villus, and facial skeleton, hair follicles, sperms, oligodendrocytes, and astrocytes; whereas PDGFR-β activates pathways implicated in maturation of blood vessels, white adipocytes, and kidneys [24].
Receptor mutations and up-regulations of PDGF/PDGFRs members were reported to cause cancer; the latter resulted in poor cancer prognosis and was implicated in cancer growth, diagnosis, and treatment resistance [24]. The increased expression levels of PDGFRs was reported in various tumors including CRC resulting in angiogenesis and metastasis due to poor response to therapy [95-98]. Wehler et al. reported the expression of PDGFR-α/β in the majority of CRC specimens investigated in 99 cohort patients and the significant association of their expression with lymphatic dissemination and cancer metastasis [99]. Similarly, Steller et al. reported the correlation of high PDGFR-β expression with CRC metastasis [98]. Moreover, Erben et al. reported a significantly increased expression of PDGFR-β mRNA in local rectal cancers in advanced stages compared to normal tissues [100]. Moreover, Kitadai et al. showed that the decreased expression of PDGFR and other angiogenic molecules can be targeted in therapeutic agents to overcome resistance to antiangiogenic agents [101,102]. Therefore, based on the above, PDGFR-𝛼 and PDGFR-𝛽 are important players involved in tumor angiogenesis, growth, invasion, and metastasis. Similarly, PDGF-A, B, C, D, & F played an important role in CRC progression and metastasis [24]. Interestingly, PDGF-B is an important oncogene involved in the binding and activation of Ras/MAP-kinase signaling via its simian sarcoma virus oncogene (v-sis); the latter results in the transcription of target genes needed for cell survival, proliferation, invasion, and metastasis (Figure 2) [24].
The wingless-type mouse mammary tumor virus integration site family (Wnt) pathway plays a major role in cancer cell formation in colorectal cancer (CRC) [103]. An aberrant Wnt-signaling pathway is generally involved in the development of colorectal cancer (CRC). WNT proteins are cysteine-rich glycoproteins secreted by cells into the extracellular matrix, resulting in the activation of receptor-mediated cell signaling [104]. WNT binds to the N-terminal extra-cellular cysteine-rich domain of a Frizzled G-protein coupled receptors; the latter inhibits the destruction complex of β-catenin (a tertiary complex formed by axin, adenomatous polyposis coli (APC), CK1α, and GSK3β) and leads to the accumulation of β-catenin in the cytoplasm (Figure 3) [104]. Wnt/β-catenin signaling pathway was implicated in cellular regulations such as proliferation, differentiation, migration, genetic stability, apoptosis, and stem cell renewal [104]. A stable β-catenin, a core component of the cadherin protein complex, is required for activating Wnt/β-catenin signaling pathway. In the nucleus, β-catenin affects transcription, proliferation and dedifferentiation of the colonic mucosa resulting in epithelialmesenchymal transition and eventually cancer development and metastasis [103].
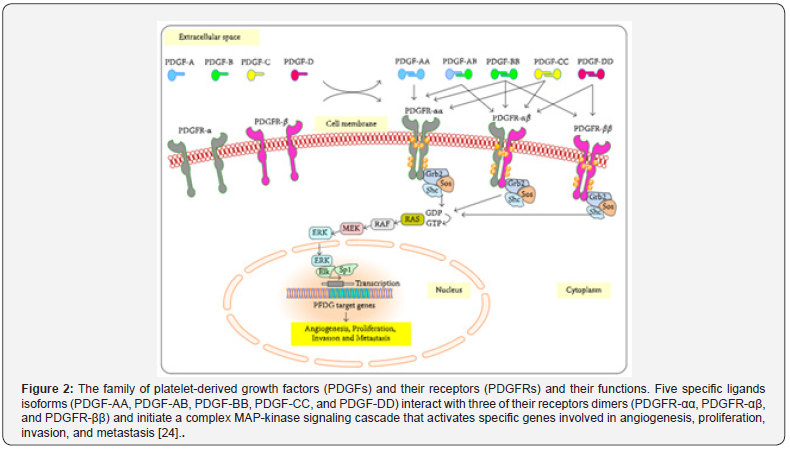
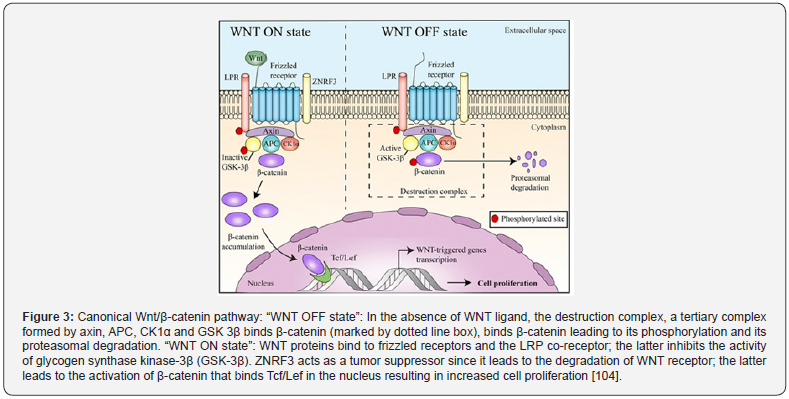
It w as proposed that the accumulation of β-catenin in the nucleus is one of the crucial events noticed in colorectal cancer (CRC). In addition to Wnt-1, the adenomatous polyposis coli (APC) gene is another important effector of the Wnt signaling pathway; the former is a negative regulator of β-catenin stability. Therefore, mutations leading to the overexpression of β-catenin and/ or loss of APC function can result in the development of CRC [103].Activation of the pathway would result in further stimulation of transcription factors involved in the epithelial-mesenchymal transition (EMT) and therefore, in cell migration and invasion. Moreover, β-catenin is able to alter the expression of some metastatic/ regulatory factors such as matrix metalloproteinases (MMPs) and therefore, regulating cancer cell progression, migration and metastasis through various downstream effectors [103].
- Review Article
- Abstract
- Colon Cancer
- Colon Cancer Epidemiology
- Colon Cancer Diagnosis
- Colon Cancer Signs and Symptoms
- Stages of Colon Cancer
- Colon Cancer Risk Factors
- Prognosis
- Signaling Pathways Involved in Colon Cancer Angiogenesis and Progression
- Colon Cancer Treatment
- Role of Immune System in Colon Cancer
- Conclusion and Future directions
- References
Colon Cancer Treatment
Colon cancer treatment includes mainly surgery, chemotherapy as primary treatment or adjunct to surgery, and recently immunotherapy was advanced [105]. Determining the suitable adjuvant chemotherapy for colon cancer patients depends on the tumor stage, presence of high-risk pathologic features, microsatellite instability status, patient age, and performance status. Systemic treatment for colon cancer patients with metastatic disease includes chemotherapy, targeted therapy, and immunotherapy [105]. In case of metastatic cancer, drugs and biological agents are used to angiogenic pathways such as VEGF pathway and EGFR pathway in addition to immunotherapeutic agents. Certain biomarkers were used to monitor treatment effectiveness and disease prognosis including cancer location (right versus left), microsatellite instability-high (MSI-high), and certain gene mutations (e.g. KRAS, NRAS, and BRAF mutations) [105]. Colon Cancer was treated by cancer drugs in the past years. These include blocking transduction pathways leading to the cell death through apoptosis and triggering of the immune system or delivering anticancer drugs to cancer cells and reducing the side effects. There major signaling pathways that could be targeted during novel colon cancer therapy include Sonic Hedgehog (SHH), Wnt/β-catenin, transforming growth factor-β (TGF-β)/ SMAD, EGFR, VEGFR, PDGFR, and Notch pathways [7,105]. Thus, inhibiting these pathways could be useful treatment against angiogenic tumors.
a) Chemotherapy
The most commonly used chemotherapeutic agents in treating CRC patients during early cancer stages as first and second line treatment include 5-fluorouracil (5FU), capecitabine (Xeloda), and oxaliplatin (Eloxatin). 5FU, a nucleotide analogue, works on suppressing the thymidylate synthase (TS) and thus, suppressing the formation of pyrimidine nucleotides. Fluorodeoxyuridine triphosphosphate (FdUTP) is the 5FU metabolite known to suppress RNA synthesis. 5FU can be given in combination with Leucovorin or Bevacizumab as first line therapy against angiogenic types of colon cancer [105,106]. Oxaliplatin, a platinum drug, is an alkylating agent known to suppress DNA synthesis. The former can be intravenously administered to CRC patients along with either 5FU or capecitabine [105]. A better disease prognosis was shown when stage II colon cancer patients were given chemotherapeutic drugs compared to others [105]. On the other hand, surgery is the main therapy for Stage III colon cancer patients; chemotherapeutic drugs can be administered after surgery to prevent disease recurrence [105]. Irinotecan a DNA topoisomerase I inhibitor also used in the first and second line of treatment and leads to DNA damage and cell death [105].
The median survival time for patients with CRC depends on the stage of the disease and treatment level/typ. For instance, the median survival time of patients with advanced colorectal cancer who are not undergoing treatment is approximately 5–6 months whereas the median survival time for those who are on chemotherapeutic drugs such as 5FU is around 10–12 months. It was reported that the response rate among CRC patients following 5FU treatment is around 20–30%. It is important to start treating metastatic diseases as soon as they are diagnosed. Throughout the years, advancing new chemotherapeutic drugs such as CPT- 11 and oxaliplatin was able to increase the patient’s response to chemotherapy to 50% and the survival rate to 18–24 months. There are several factors influencing the effectiveness and responsiveness to chemotherapy. These include: a. Factors related to the patient (age, gender in that females have longer median survival times than males, the performance status of the patient, and presence of tumor-related symptoms) [107,108]; b. Factors related to the disease (disease correlation with the probability of response and survival, degree of disease metastasis, number of lesions within each metastatic site, and percent liver involvement or, indirectly, by baseline LDH and WBC values); and Factors related to the treatment (resistance to second-line treatment due to prior chemotherapy for advanced disease resistance to adjuvant treatment, and survival analysis of responding vs non-responding to chemotherapy patients) [22]. Recently, it was proposed that adding the targeted therapy such as bevacizumab, cetuximab and panitumomab to the cytotoxic or chemotherapy increased the survival rate among patients with advanced type of CRC [106].
Sorafenib Treatment: Angiogenesis and signaling cascades are important for tumor growth, survival, invasion and metastasis. Activation or inhibition of VEGF, PDGFR-mediated, the PI3K/ Akt pathway, MAPK/ERK pathways plays a crucial role in tumor pathogenesis. Therefore, a treatment that targets these angiogenic pathways may be a beneficial therapy against cancer. Sorafenib inhibits tumor growth, tumor cell proliferation, angiogenesis and induces tumor cell apoptosis via targeting serine/threonine Raf kinases, MAPK signaling pathway and inhibiting VEGFRs and platelet-derived growth factor receptor (PDGFR) auto-phosphorylation. It is FDA-approved for treating hepatocellular carcinoma and advanced renal cell cancer in adults. However, the number of research studies on the activity and effect of sorafenib in treating CRC is limited [106]. The effect of sorafenib on angiogenesis was evaluated in a preclinical model whereby a significant reduction in tumor vascularity was shown.
Gunningham et al showed that VEGFR and angiogenesis play a central role in renal cell carcinoma (RCC) and sorafenib showed beneficial outcomes [109]. In addition, sorafenib has shown advantages to placebo in patients with refractory metastatic RCC [106]. In another preclinical study performed by Liu et al., sorafenib increased tumor cell apoptosis and decreased tumor vascularization in PLC/PRF/5 hepatocellular cell cancer (HCC) xenograft model [110].
Regorafenib is an orally administered tyrosine kinase inhibitor (TKI) that was produced from sorafenib optimization further to undergoing changes to its molecular structure. Regorafenib is characterized by having a broader range of inhibition and more efficient activity as compared to sorafenib due to the presence of the unique fluorine atom in the central phenyl ring of its structure [111]. Both regorafenib and sorafenib are inhibitors of tyrosine kinases that are mediators of cancer cell generation and disease progression. Tyrosine kinases inhibited by these 2 drugs include the isoforms of rapidly accelerated fibrosarcoma (RAF) RAF-1, B-RAF, vascular endothelial growth factor receptor (VEGFR), and platelet-derived growth factor receptor (PDGFR) β. However, a more advantaged function of regorafenib is that it is also able to inhibit more important and essential factors required for cancer development, maintenance, and progression [111,112]. For instance, regorafenib can suppress the mutant isoform of B-RAF, B-RAFV600E, the receptor tyrosine kinase type III (KIT), Rearranged during Transfection (RET) tyrosine kinase receptor, angiopoietin 1 receptor (TIE2), PDGFRα and fibroblast growth factor receptors (FGFRs) [111,113].
However, it was proposed that higher doses of sorafenib can achieve the inhibition of B-RAFV600E, KIT and RET kinases [111]. When comparing the ability of both drugs to inhibit tyrosine kinases, sorafenib was found to have a weaker effect since it has a weaker affinity to tyrosine kinases. Moreover, the ability of regorafenib to block and inhibit larger number of tyrosine kinases involved in cancer progression and angiogenesis had proved that regorafenib is a more stronger tyrosine kinase inhibitor and can give a more promising results in treating various types of cancer when other chemotherapeutic agents fail to do so [111]. For instance, clinical trial studies has evidenced that regorafenib was an approved second-line therapy for advanced hepatocellular carcinoma (HCC) and a third-line therapy for other cancer types such as colorectal cancer (CRC) in advanced stage and gastrointestinal stromal tumors (GISTs) [111]. Despite its effectiveness, there are some reported intrinsic and acquired resistances against regorafenib in colon cancer [114]. A recent study has combined regorafenib with Nivolumab, an immunotherapeutic agent using monoclonal antibodies, for more effective treatment among patients with Advanced Gastric or Colorectal Cancer [115].
Moreover, it was recently reported that the addition of targeted therapy such as bevacizumab, cetuximab and panitumumab to the traditional chemotherapeutic agents, resulted in a prolonged survival among patients with CRC. For instance, combination of sorafenib and erlotinib cetuximab was able to produce an effectiveness activity against human colorectal and lung cancer cells [106]. Interestingly, the combined drugs produced a synergistic cytotoxic activity against cancer cells. Furthermore, the combination of sorafenib with radiation was reported in 2 different studies. In the first study, Plastaras et al observed the ability of sorafenib to suppress cancer progression among cells prior to radiotherapy by producing a reversible day in G1 phase of the cell cycle. Applying radiotherapy to these cells after sorafenib treatment resulted in delayed growth of tumor cells [116]. In the other study, however, Suen and colleague studied the effect of sorafenib and radiation therapy on HT29 and SW48 colorectal cancer cells in vitro using MTT assays. They were able to predict the best timing required for the effectiveness of radiation and sorafenib against colorectal cancer cells. Then, they were able to report daily the growth delay among colorectal cancer cells for a period of 3 weeks.
The results of this study showed that the combination of radiation and sorafenib inhibited tumor proliferation and angiogenesis on human colorectal cancer cell lines. Also, this combination was synergistic however, the effect was stronger in vivo as compared to in vitro results [117]. Sorafenib was also combined with platinum derivatives such as cisplatin and oxaliplatin in vitro against colorectal cancer cell lines. Sorafenib has the ability to interact with the transporter of platinum compounds, and therefore, it can lead to a decreased uptake of these compounds by the cells. When sorafenib is combined with platinum derivative, it caused a marked antagonistic effect and resulted in stopping the cell cycle at the level of G1 or G2 phases [118]. Despite of the better therapeutic results achieved while combining chemotherapy with targeted agents like monoclonal antibodies, radiotherapy and others, disease prognosis has been also reported [106]. Thus, better and more advanced treatments should be developed.
b) Immunotherapy
Cancer immunotherapy has switched the model and given some flavor for the treatment of various types of cancers; these therapies target the anti-tumor immune responses to directly kill tumor cells with few different targets than other types of therapies including chemotherapy [119]. In cancer immunotherapy, the mode of action of used agents depends on the activation of natural immune cells to attack or kill cancer cells. Interestingly, cancer progression may occur due to cancer cells’ ability to escape immune responses [120]. Thus, immunotherapy is recognized as a promising strategy to treat, and even cure, certain types of cancer. The first antitumor immunotherapeutic drugs found in the market were the recombinant versions of the cytokine interferon-α (IFNα), which were approved by the US Food and Drug Administration (FDA) in 1986 for hairy cell leukemia [121,122]. A partial disease remission was reported among some patients who were administered with IFNα. Later, IFNα was replaced by purine analogues, the latter was used as primary treatment for hairy cell leukemia. After that, recombinant interleukin-2 (IL-2) was studied as an immunotherapeutic agent for treating cancer and it was then approved by the FDA for metastatic renal cancer in 1992 and for metastatic melanoma in 1998 [120,122]. Researchers were more excited and eager to study the effect of IL-2 therapy because of the complete responses it achieved among most of the patients [123,124]. However, IL-2 has a shorter half-life which required the administration of higher doses; the latter resulted in serious adverse effects including cytokine release syndrome and vascular leak syndrome, and others [123]. Despite these promising results in cancer immunotherapy, studies in this field were decreased as of year 2000 until 2010- the year marking a decade of reported failure among various clinical trials involving vaccine development [125].
After that, in 2010, sipuleucel-T, an autologous dendritic cell therapy, was the first successful therapeutic approved cancer vaccine for treating prostate cancer. However, it was reported that this cancer vaccine resulted in lots of complexities and health issues among patients [126]. After that, in 2011, the pioneering checkpoint inhibitor ipilimumab, a monoclonal antibody (mAb) that targets cytotoxic T lymphocyte antigen 4 (CTLA4), was approved for treating advanced melanoma [119,127]. In the past years, new immunotherapies were also developed. The latter include checkpoint inhibitor mAbs that target programmed cell death 1 (PD-1) or its ligand, PD-1 ligand 1 (PD-L1) [128] and the first chimeric antigen receptor (CAR) T cell therapies [129,130]. However, a shifting and marking out in cancer immunotherapy was the development of ipilimumab and CAR T cell therapies highlighted by Science as the breakthrough of the year in 2013 [131]. There are several immunotherapies developed. These are classified into different classes that include checkpoint inhibitors, lymphocyte-activating cytokines, CAR T cells and other cellular therapies, agonistic antibodies against co-stimulatory receptors, cancer vaccines, oncolytic viruses, and bispecific antibodies [119].
Despite these major advances, the clinical use of immunotherapies faces several challenges related to both efficacy and safety. These include difficulty in predicting the efficacy of those therapies due to challenges in predicting patients’ response since only some patients were able to respond to immunotherapies [119,132]. Here comes the interest of researchers to work on developing patient-specific immunotherapies by targeting the expression of certain cancer cell biomarker and to evaluate the effect of combination treatment strategies on improving response rates [119,133,134]. Interestingly, in the last couple of years, FDA approved several immunotherapies, including activating cytokines and mAbs for checkpoint blockade, as treatments for solid tumor therapy [135]. However, CAR T cell therapies have not yet been approved by the FDA for solid tumors, but researchers are developing CAR T cells that have high specificity towards cells in solid tumors such as breast cancer [136] and glioblastoma [137]. Immunotherapeutic agents are safe to patients however, it was also reported that they can induce autoimmune attack to healthy tissues in some patients [119]. As described above regarding IL-2 therapy, many immunotherapies may cause cytokine release syndrome and vascular leak syndrome, which lead to severe hypotension, fever, renal dysfunction, and other adverse lethal effects [124,138,139].
Newer studies that focus on administering these immunotherapeutic agents in a safe and more controlled strategy would increase the effectiveness of those agents and decrease their adverse effects and toxicity levels. Interestingly, strategical plans may include using certain agent administration techniques to deposit and accumulate the agents in the diseased target tissues for effective direct result in killing the tumor cell and with less adverse effects [119]. There are lots of studies taking place to study different new modes of delivering immunotherapeutic agents to the targeted cells; the latter include nanoparticles, implants, scaffolds, biomaterials and cell-based platforms [140].
i. Classes of cancer immunotherapy
a) Checkpoint Inhibitors: Checkpoint inhibitors are the most studied types of immunotherapeutic agents. Among these, the two most common checkpoint inhibitors: those that block PD-1/PD-L1 blockade and those that block CTLA4 [119, 141, 142]. The role of immune checkpoints is to maintain appropriate immune responses and protect healthy tissues from being attacked by immune cells [143]. Upon T cell activation (e.g. in response to infection/inflammation), PD-1 are expressed on them; the latter enables them to recognize abnormal and cancerous cells [144, 145]. Tumor cells express PD-L1; the latter binds to PD-1 on T cells to escape the T cell immune response and render those cells inactive [142, 144, 146]. Therefore, the use of monoclonal antibodies that target the PD-1/PD-L1 interaction can activate tumor cell death by T cells [119]. CTLA4 is a checkpoint inhibitor that suppresses T cell activity via its interactions with ligands CD80 and CD86 leading to tumor progression [142, 147]. The blockage of interaction between CTLA4 and these ligands keeps the T cells active and retains their ability to kill tumor cells. Research is still ongoing to study mechanisms involved in CTLA blockage using CTLA4-specific antibodies [148].
In the past few years, studies were able to show the significant effect of blocking PD-1, PD-L1 or CTLA4 checkpoint inhibitors at the clinical level [141, 142]. Until this date, five PD-1 or PDL1 inhibitors and one CTLA4 inhibitor were used and approved to treat various cancers. These newly discovered inhibitors were approved for treatment use based on the positive impact they produced including the increased survival rate among cancer patients as compared to traditional chemotherapeutic agents [149]. Many clinical trials and research studies involving checkpoint inhibitors in combination with chemotherapy or other targeted agents (>700 trials) are still ongoing. However, these treatments induce certain severe side effects that might affect various body organs [139] and lead to multiple factors involved in patients’ unresponsiveness to treatment [132]. These Factors include low numbers of tumor-infiltrating T cells, deregulation of checkpoints in both tumor cells and T cells, and adapted resistance to checkpoint inhibition [150,151]. Therefore, new studies and novel approaches for new treatments are required for different types of tumors since different tumors involve different immunosuppressive mechanisms and thus, different treatment targets [152].
b) Cytokines: Historically, cytokines were the first discovered types of immunotherapeutic agents for clinical use. The first approved therapeutic cytokine was the recombinant IFNα in 1986 [119]. The main difference between cytokines and checkpoint blockade inhibitors is that cytokines are directly injected into the body and subsequently, they activate the immune cells. The three main types of cytokines that have been implicated in immunotherapy are interferons, interleukins, and granulocyte– macrophage colony-stimulating factor (GM-CSF) [124]. Interferons are produced by immune cells upon the invasion of microbial pathogens in order to induce immune response. The latter is produced through stimulating the growth and maturation of various immune cells involved in natural and acquired immunity such as macrophages, natural killer (NK) cells, lymphocytes and dendritic cells [153]. Interestingly, the stimulation of immune response by interferons would suppress angiogenesis in tumor microenvironment [124,154,155]. Moreover, interleukins result in CD4+ T cells and CD8+ T cells growth and activation [156]. Finally, GM-CSF activates and maintains immune responses through two mechanisms: 1. Enhancing and maintaining T cell homeostasis; the latter would prolong T cell survival; 2. Activating dendritic cell differentiation so that these cells would express tumorspecific antigens to enhance immunity [157]. It was proposed that granulocyte colony-stimulating factor (G-CSF) and GM-CSF are used after chemotherapy to enhance and increase the rate of granulocyte recovery. However, GM-CSF can be more proinflammatory than G-CSF [157]. Research involving agonists and ligands that might activate immune cells intercellularly is still ongoing. These include TGFβ receptor type 1 (TGFβR1) inhibitors, such as SD-208, that restore T cell function and improve immune responses [158], small-molecule agonists of TLR7/TLR8 that directly activate antigen-presenting cells (APCs) to promote antitumor activity, and stimulator of interferon genes (STING) agonists that have been used to induce pro-inflammatory cytokine production and other type I interferon responses [159,160].
Moreover, there are three recombinant cytokines involved in immune activation in cancers are in clinical development and have been approved for use in cancer immunotherapy such as IL-17 and IL-15 [161]. However, due to the short half of these cytokines, treatment usually relies on injecting high bolus doses; the latter would result in vascular leakage and cytokine release syndrome [124]. Moreover, it was reported that cytokine therapy can increase the survival rate of regulatory T cells resulting in the death of stimulated T cells, and eventually leading autoimmune response through the activation of immune cells against healthy tissues [138]. Recent research is ongoing to study the effect of cytokine combination therapy using two or more cytokines (for example, interleukins and interferons together), cytokines and checkpoint inhibitors, cytokines and chemotherapies, etc. as a strategical step to reduce or eliminate the adverse effects resulting from high-doses of cytokine injections when the latter are used independently [154].
Adoptive T cell Therapy or Engineered T cells (chimeric antigen receptor T and T cell receptor T cells): In addition to immunecheckpoint inhibition and cytokines, cell-based immunotherapies referred to as adoptive cellular therapy (ACT) have shown efficacy in treating patients with cancer. Broadly, ACT can be achieved in two forms: 1. Isolating tumor-infiltrating T cells from a patient’s tumor, expanding it ex vivo and subsequently introduce activating factors specific for T cells to enhance T cell growth and activity and to increase the survival rate of expanded cells [120,162]; 2. isolating autologous T cells to be genetically modified and eventually express chimeric antigen receptors (CARs) encoding an antigen-specific single-chain variable fragment of an antibody and various co-stimulatory molecules. Interestingly, the specificity of CAR T cells is does not rely on recognizing the MHC antigens or peptides produced by the tumor of tumor therefore, activation of the former is different than that of T cell receptor-transgenic T cells [163]. Therapies involving T cells have shown to be effective in some cancer treatments such as hematological malignancies [164]. However, the effectiveness of those treatments among solid tumors was not well studied [165]. Recently, the combination of immune-checkpoint inhibitors and adoptive T cell therapy in the form of ex vivo expanded tumor-infiltrating T cells showed a promising result in a single patient with chemo-refractory hormone receptor-positive breast cancer [166].
The reported successes of CAR T cells at the clinical level increased the interest of researchers and accelerated FDA approvals. In the CAR T cell, T cells are isolated form the patient’s blood to be genetically modified in the lab to express CARs specific to the tumor cell antigen [119,142]. These lab-modified T cells are then injected to the same patient whereby, the injected CAR T cells can induce tumor cell death via recognizing the targeted antigen on tumor cells [142,167]. The advantage of the CAR T cell therapy among others is that CAR T cells once present, they are able to maintain their activity for decades and therefore, it is a onetime injection therapy [130]. Until now, good results were achieved while using the CAR T cell especially in prolonging the survival rate among cancer patients however, the effect of this treatment on long-term run should be further investigated [129]. Moreover, several cons are implicated in the slow use of this treatment. These include treatment complexity, time-consumption, and high costs [168]. In addition, in some circumstances (particularly solid tumors with harsh microenvironments), the injected cells do not stay in the body and therefore, novel studies include combination therapies or strategies to directly deliver the injected cells into the tumor microenvironment to maintain their activity and increase their survival rates [119].
CD19, expressed on B cell leukemia and lymphomas was considered as the primary target of CAR T cells. However, it is mainly expressed by B cells in normal tissues therefore, in an attempt to prevent B cell aplasia side effect caused by dysregulation of target activity, immunoglobulin replacement therapy can be used [169]. Currently, there are 2 FDA approved CD19-targeting CAR T cell therapies for clinical use. These include axicabtagene ciloleucel for diffuse large B cell lymphoma and tisagenlecleucel for acute lymphoblastic leukemia and diffuse large B cell lymphoma [170,171]. The clinical success of CD19 CAR T cells has encouraged scientists to exert more efforts in using lab engineered CAR T cells to target different antigens on cancer cells for a more inclusive and generalized cancer therapy [167]. However, these efforts encountered two main challenging factors: First, the ability of both CAR T and TCR T cells to induce cytokine release syndrome and neurotoxicity [172]; second the factor involved in their ability to use these engineered cells to effectively treat solid tumors [173,174]. Until now, few solid tumors were successfully treated with CAR T cells. For instance, CAR T cells were effective in treating EGFRvIII-expressing glioblastoma since in this case the target antigen was highly expressed on tumor cells as compared to normal cells [173].
TCR T cells are another example of engineered cells. These cells were studied in in clinical trials for their effectiveness against hematological and solid cancers. TCRs target the tumorassociated intracellular antigens that are presented by major histocompatibility complexes (MHCs) [175]. Shared antigens can be used as potential targets TCR T cells including cancertestis antigens, or patient-specific neoantigens that result from tumor mutations. However, here the case is different than that of CAR T cells in that MHC must be matched with the patient [176]. Researchers are working to develop new modes of delivery to decrease or eliminate the toxicity that might result from using both CAR T cells and TCR T cells and increase their implication and effectiveness against solid tumors [119]. Co-stimulatory receptor agonists: Agonistic antibodies are developed with a capability of binding T cell receptors thus, activating intracellular pathways involved in T cell growth, survival and activation against tumor cells [177]. The most commonly targeted T cell receptors are costimulatory receptors (namely CD28) and several members of the tumor necrosis factor receptor (TNFR) family, such as 4-1BB (also known as TNFRSF9 or CD137), OX40 (also known as TNFRSF4) and glucocorticoid-induced TNFR-related protein (GITR; also known as TNFRSF18), that are expressed on the surface of antigen presenting cells (APCs) [178]. The interaction between agonists and these co-stimulatory receptors would activate intracellular cell signaling pathways involved in T cell growth and activation of T cells to kill cancer cells [138]. However, the interaction between the original body ligand and Tumor necrosis factor receptors (TNFR) would lead to the activation of signaling pathways involving the c-Jun N-terminal Kinases (JNK) and phosphatidylinositol 3-kinase or protein kinase B (PI3K-PKB also known as AKT) [178], leading to cancer cell survival, proliferation, and angiogenesis.
Although agonistic antibodies have been developed prior to any other type of immunotherapeutic agents, none of them have been approved by the FDA for clinical use. However, most of them have reached clinical trials. Among these, the most recent ones in clinical trials are agonistic antibodies targeting 4-IBB (utomilumab and urelumab) [179,180] and those that target OX40 (PF-04518600, BMS-986178 and INCAGN-01949, among others) [181]. However, studies have shown that agonistic antibodies have toxicities in relation to the dose levels similar to those encountered among cytokines, induce regulatory T cells leading to the activation of immune cells against healthy cells and autoimmunity [178,182]. Research is still ongoing to predict the specific doses required to eliminate the toxicity effects and to develop new delivery strategies of these agents. It was proposed that delivery platforms are essential in toxicity reduction. For instance, delivering anti-4-1BB agonistic antibodies linked to liposomal nanoparticles was able to increase the number of agonistic antibodies in the tumor microenvironment and decrease the toxicity levels as compared to those delivered alone in mice models with metastatic lung cancer [183].
c) Cancer Vaccines: There are various types of cancer vaccines. These include tumor cell lysate, dendritic cells, nucleic acids (such as mRNA) or neo-antigens [184]. Dendritic cell vaccines are the most commonly studied class of cancer vaccine using immune cells [185]. Dendritic cell vaccines are made from dendritic cells isolated from cancer patients and laboratory modified to express tumor antigens; the latter would directly activate T cells to kill tumor cells [185]. One dendritic cell vaccine, sipuleucel-T, was approved to treat prostate cancer in 2010 on the basis of its ability to prolong overall survival [126]. However, other types of dendritic cell-based vaccines failed in clinical trials due to the lack of efficacy in treating cancers. This efficacy can be further improved if more studies are done to identify dendritic cells that express high levels of targeted antigens and facilitate the delivery of those cells to the target lymph nodes to enhance their activation [185].
Nucleic acid-based vaccines including DNA or RNA, could be used as alternatives to the traditional vaccines. These vaccines depend on the delivery of external nucleic acids into the target cell [186]. DNA or mRNA will be internalized by APCs and translated to enhance antigen presentation. APCs will present the targeted tumor-specific antigen to T cells leading to their activation [186]. Despite the studies involving DNA vaccines, the latter were ineffective due to the lack of appropriate delivery modes and to immunogenicity [187]. Alternatively, mRNA-based cancer vaccines, due to their natural and easy production, were able to directly activate tumor-specific antigen expression on APCs in a non-infectious manner and without integrating into the host’s genome [186]. However, delivery platforms are stil, l required for mRNA-based vaccines because mRNA are easily degradable by nucleases and cannot be easily internalized by the cells [186]. Neoantigen vaccines are a type of cancer immunotherapy known for their ability to boost the immunity against cancer cells [141, 188]. Neoantigens are antigens produced from DNA mutations in the tumor cell and therefore, they are only expressed on tumor cells eliminating any autoimmune response [188]. Furthermore, these types of vaccines can contain an unlimited number of neoantigens and therefore, they can be used to treat multiple cancers at a time [188].
ii) Immunotherapy and angiogenesis
EGF is a growth factor secreted by tumor cells and their adjacent stromal cells to enhance angiogenesis and cancer metastasis. Recently, monoclonal antibodies have been developed to target either the VEGF ligand itself or its receptors (VEGFR). Bevacizumab (Avastin), the first approved biological agent to treat metastatic colorectal cancer, is a monoclonal antibody that binds the ligand VEGF-A. However, studies have shown that better results were achieved upon combining bevacizumab with chemotherapeutic agents in phase III trial and in a less period of time [105]. Bevacizumab is FDA approved as a first- and secondline cancer treatment in combination with 5FU-containing chemotherapy. It was also effective when administered after disease progression. Moreover, other immunotherapeutic agents targetting the VEGF pathway were used. These include zivaflibercept (Zaltrap), a recombinant fusion protein that binds VEGFR-1 and VEGFR-2 and targets VEGF-A, VEGF-B, and PIGF and Ramucirumab (Cyramza), a monoclonal antibody that targets VEGR-2. Similarly, better results were noticed when administering these agents (ziv-aflibercept or Ramucirumab) in combination with chemotherapeutic agents versus mere chemotherapy [105]. Therefore, ziv-aflibercept and ramucirumab were FDA approved to be administered in the second line setting only in combination with chemotherapy and never alone [105].
- Review Article
- Abstract
- Colon Cancer
- Colon Cancer Epidemiology
- Colon Cancer Diagnosis
- Colon Cancer Signs and Symptoms
- Stages of Colon Cancer
- Colon Cancer Risk Factors
- Prognosis
- Signaling Pathways Involved in Colon Cancer Angiogenesis and Progression
- Colon Cancer Treatment
- Role of Immune System in Colon Cancer
- Conclusion and Future directions
- References
Role of Immune System in Colon Cancer
Solid tumors consist of a complex system that contains immune cells, fibroblasts, endothelial cells, and various other types of cells [189]. The immune cells present in this complex system play various roles. These include roles that might enhance tumor development and roles that eliminate tumor growth and kill tumor cells. Historically, the immune system is divided based on its function into innate immunity and adaptive/acquired immunity. The latter would lead to what is called the immunological memory.
One of the major factors implicated in cancer progression and malignant transformation is the chronic inflammation. For instance, in colorectal cancer (CRC), inflammatory bowel disease (IBD) is significantly present and associated with increased risk of malignant transformation. Pathways involved in tumor development and disease progression are enhanced by inflammatory signals activated by the tumor cell or by the host’s immune system while attacking cancer cells. Those signals include the activation of apoptotic cells, damage-associated molecular patterns, free DNA molecules, heat shock proteins and Tolllike receptors (TLRs)/ligands or cytokines therefore, resulting in immune activation. Furthermore, the activation of immune responses would result in the production of chemokines.
The role of chemokines is to attract immune cells to their target and therefore, here they may lead to tumor growth and dissemination by activating or inhibiting certain immune cells. Innate immune cells include physical epithelial barriers, phagocytic leukocytes (such as granulocytes and macrophages), dendritic cells, natural killer (NK)/innate lymphoid cells and circulating plasma proteins. Some factors were challenging to scientists while studying the presence of certain innate immune cell in the tumor microenvironment. For instance, despite that fact that NK cells were known to have a great influence in cancers like breast cancer and CRC, recently, it was reported that these cells are absent from these tumors. However, interestingly, the products of these cells including chemokines and adhesion factors were present. Moreover, scientists were challenged by the presence of various myeloid cell origins. Furthermore, fibroblasts and other mesenchymal cell types are a significant constituent of the tumor cell. They affect the travel of immune cells and the ability of cancer cells to metastasize [189].
One of the most critical challenges encountered during immunotherapy is the presence of different phenotypes of immune cells involved in cancer progression and metastasis; the latter can be overcome by several mechanisms including isolating the person’s own immune cells to multiply and reinject into the patient in order to target the same particular type of cancer and using treatments that target the complex interaction between the innate and adaptive immunity [189]. Over the past decade, various studies have reported the significant dual effect of immune system in eliminating/enhancing cancer cells and inhibiting/activating disease progression in mouse and human models. This dual role by which the immune system can suppress and/or promote cancer growth is termed cancer immunoediting and consists of three phases: elimination, equilibrium, and escape [190]. This dual role was well reported in T cells and in tumor-associated inflammation. For instance, tumor-related chronic inflammation can enhance tumor development due to immunosuppression whereas acute inflammation can lead to immune activation against tumor cells by enhancing DC maturation and T cell activation [190].
- Review Article
- Abstract
- Colon Cancer
- Colon Cancer Epidemiology
- Colon Cancer Diagnosis
- Colon Cancer Signs and Symptoms
- Stages of Colon Cancer
- Colon Cancer Risk Factors
- Prognosis
- Signaling Pathways Involved in Colon Cancer Angiogenesis and Progression
- Colon Cancer Treatment
- Role of Immune System in Colon Cancer
- Conclusion and Future directions
- References
Conclusion and Future directions
In conclusion, this review points out the collective information in relation to signaling pathways involved in colon cancer progression and angiogenesis. Moreover, it also targets the various treatments used in colon cancer therapy. The available data can be further utilized by scientists to build upon in their future research projects.
- Review Article
- Abstract
- Colon Cancer
- Colon Cancer Epidemiology
- Colon Cancer Diagnosis
- Colon Cancer Signs and Symptoms
- Stages of Colon Cancer
- Colon Cancer Risk Factors
- Prognosis
- Signaling Pathways Involved in Colon Cancer Angiogenesis and Progression
- Colon Cancer Treatment
- Role of Immune System in Colon Cancer
- Conclusion and Future directions
- References
References
- Spallanzani A, Gelsomino F, Caputo F, Santini C, Andrikou K, et al. (2018) Immunotherapy in the treatment of colorectal cancer: a new kid on the block. J Cancer Metastasis Treat 4(6): 28.
- Cunningham D, Atkin W, Lenz HJ, Lynch HT, Minsky B, et al. (2010) Colorectal cancer. Lancet 375(9719): 1030-1047.
- Siegel RL, Miller KD, Goding Sauer A, Fedewa SA, Butterly LF, et al. (2020) Colorectal cancer statistics. CA: A Cancer Journal for Clinicians 70(3): 145-164.
- Siegel, Miller KD, Jemal A (2018) Cancer statistics. CA Cancer J Clin 68(1): 7-30.
- Issa IA, Noureddine M (2017) Colorectal cancer screening. An updated review of the available options, World J Gastroenterol 23(28): 5086-5096.
- Zhang HL, Wang P, Lu MZ, Zhang ZD, Zheng L (2019) c‑Myc maintains the self‑renewal and chemoresistance properties of colon cancer stem cells. Oncology letters 17(5): 4487-4493.
- Tiwari A, Saraf S, Verma A, Panda PK, Jain SK (2018) Novel targeting approaches and signaling pathways of colorectal cancer: An insight. World Journal of Gastroenterology 24(39):4428.
- Mardi K, Sharma M, Bhardwaj M, Rao M (2017) p53 expression in colorectal carcinomas and its correlation with clinicopathological parameters. Clinical Cancer Investigation Journal 6(1): 26-29.
- Cappell MS, (2008) Pathophysiology, clinical presentation, and management of colon cancer Gastroenterology Clinics of North America 37(1): 1-24.
- Chen QF, Zhou XD, Sun YJ, Fang DH, Zhao Q, et al. (2017) Sex-influenced association of non-alcoholic fatty liver disease with colorectal adenomatous and hyperplastic polyps. World journal of gastroenterology 23(28): 5206-5215.
- Arnold M, Sierra MS, Laversanne M, Soerjomataram I, Jemal A, et al. (2017) Global patterns and trends in colorectal cancer incidence and mortality. Gut 66(4): 683-691.
- Yoshida I, Suzuk A, Vallée M, Matano Y, Masunaga T, et al. (2006) Serum insulin levels and the prevalence of adenomatous and hyperplastic polyps in the proximal colon. Clinical Gastroenterology and Hepatology 4(10):1225-1231.
- Morimoto LM, Newcomb PA, Ulrich CM, Bostick RM, Lais CJ, et al. (2002) Risk factors for hyperplastic and adenomatous polyps: evidence for malignant potential? Cancer Epidemiology and Prevention Biomarkers 11(10): 1012-1018.
- Ji BT, Weissfeld JL, Chow WH, Huang WY, Schoen RE, et al. (2006) Tobacco smoking and colorectal hyperplastic and adenomatous polyps. Cancer Epidemiology and Prevention Biomarkers 15(5): 897-901.
- Huang E, Whelan R, Gleason N, Maeda J, Terry M, et al. (2001) Increased incidence of colorectal adenomas in follow-up evaluation of patients with newly diagnosed hyperplastic polyps. Surgical endoscopy 15(7): 646-648.
- Bittoni A, Sotte V, Meletani T, Cantini L, Giampieri R, et al. (2018) Immunotherapy in colorectal cancer treatment: actual landscape and future perspectives. J. Cancer Metastasis Treat 4: 55.
- Rawla P, Sunkara T, Barsouk A (2019) Epidemiology of colorectal cancer: incidence, mortality, survival, and risk factors. Przeglad gastroenterologiczny 14(2): 89-103.
- Society AC (2020) Colorectal cancer facts & figures 2020-2022. Published online p. 48.
- Birendra K, Hwang JJ, Farhangfar CJ, Chai SJ (2017) Advances in immunotherapy in the treatment of colorectal cancer. American Journal of Hematology/Oncology 13: 7.
- Glover M, Mansoor E, Panhwar M, Parasa S, Cooper GS (2019) Epidemiology of colorectal cancer in average risk adults 20–39 years of age: a population-based national study. Digestive diseases and sciences 64(12): 3602-3609.
- Kwak EL, Chung DC (2007) Hereditary colorectal cancer syndromes: an overview. Clinical colorectal cancer 6(5): 340-344.
- Labianca R, Beretta GD, Kildani B, Milesi L, Merlin F, et al. (2010) Colon cancer. Critical Reviews in Oncology/Hematology 74(2): 106-133.
- C. C. A. Campaign, 2019.
- Manzat Saplacan RM, Balacescu L, Gherman C, Chira RI, Craiu A, et al. (2017) The role of PDGFs and PDGFRs in colorectal cancer. Mediators of inflammation 2017.
- Siregar GA, Sibarani H (2019) Comparison of Carcinoembryonic Antigen Levels Among Degree of Differentiation and Colorectal Cancer's Location in Medan. Open access Macedonian journal of medical sciences 7(20): 3447-3450.
- Nakatani H, Kumon T, Kumon M, Hamada S, Okanoue T, et al. (2012) High serum levels of both carcinoembryonic antigen and carbohydrate antigen 19-9 in a patient with sigmoid colon cancer without metastasis. The Journal of Medical Investigation 59(3-4): 280-283.
- Jelski W, Mroczko B (2020) Biochemical Markers of Colorectal Cancer–Present and Future Cancer Management and Research 12: 4789-4797.
- Garranzo-Asensio M, San Segundo-Acosta P, Povés C, Fernández-Aceñero MJ, Martínez-Useros J, et al. (2020) Identification of tumor-associated antigens with diagnostic ability of colorectal cancer by in-depth immunomic and seroproteomic analysis. Journal of Proteomics 214: 103635.
- Hashiguchi Y, Hase K, Kotake K, Ueno H, Shinto E, et al. (2012) Evaluation of the seventh edition of the tumour, node, metastasis (TNM) classification for colon cancer in two nationwide registries of the United States and Japan. Colorectal Dis 14(9): 1065-1074.
- Freeman HJ (2013) Early stage colon cancer. World journal of gastroenterology 19(46): 8468-8473.
- Liljegren A, Lindblom A, Rotstein S, Nilsson B, Rubio C, et al. Prevalence and incidence of hyperplastic polyps and adenomas in familial colorectal cancer: correlation between the two types of colon polyps. Gut 52(8): 1140-1147.
- Sostres C, Gargallo CJ, Lanas A (2014) Aspirin, cyclooxygenase inhibition and colorectal cancer. World journal of gastrointestinal pharmacology and therapeutics 5(1): 40-49.
- Wiseman M (2008) The second world cancer research fund/american institute for cancer research expert report. Food, nutrition, physical activity, and the prevention of cancer: A global perspective: Nutrition society and bapen medical symposium on ‘nutrition support in cancer therapy. Proceedings of the Nutrition Society 67(3): 253-256.
- Baena R, Salinas P (2003) Diet and colorectal cancer. Maturitas 80(3): 258-264.
- Stewart BW, Kleihues P (2003) World cancer report.
- Giovannucci E (2001) An updated review of the epidemiological evidence that cigarette smoking increases risk of colorectal cancer. Cancer Epidemiology and Prevention Biomarkers 10(7): 725-731.
- Asano T, McLeod R (2004) Nonsteroidal anti-inflammatory drugs and aspirin for the prevention of colorectal adenomas and cancer: a systematic review. Diseases of the colon & rectum 47(5): 665-673.
- Von Roon AC, Reese G, Teare J, Constantinides V, Darzi AW, et al. (2007) The risk of cancer in patients with Crohn’s disease. Diseases of the colon & rectum 50(6): 839-855.
- Eaden J, Abrams K, Mayberry J (2001) The risk of colorectal cancer in ulcerative colitis: a meta-analysis. Gut 48(4):526-535.
- Ahmed RL, Schmitz KH, Anderson KE, Rosamond WD, Folsom AR, et al. (2006) The metabolic syndrome and risk of incident colorectal cancer. Cancer: Interdisciplinary International Journal of the American Cancer Society 107(1): 28-36.
- Chlebowsk RT, Wactawski-Wende J, Ritenbaugh C, Hubbell FA, Ascensao J, et al. (2004) Estrogen plus progestin and colorectal cancer in postmenopausal women. New England Journal of Medicine 350(10): 991-1004.
- Loomba R, Sanyal A (2013) The global NAFLD epidemic. Nature reviews Gastroenterology & hepatology 10(11): 686-690.
- Liu Y, Dai M, Bi Y, Xu M, Xu Y, et al. (2013) Active smoking, passive smoking, and risk of nonalcoholic fatty liver disease (NAFLD): a population-based study in China. Journal of epidemiology 23(2): 115-121.
- Gaggini M, Morelli M, Buzzigoli E, DeFronzo RA, Bugianesi E, et al. (2013) Non-alcoholic fatty liver disease (NAFLD) and its connection with insulin resistance, dyslipidemia, atherosclerosis and coronary heart disease. Nutrients 5(5): 1544-1560.
- Bugianesi E, Gastaldelli A, Vanni E, Gambino R, Cassader M, et al. (2005) Insulin resistance in non-diabetic patients with non-alcoholic fatty liver disease: sites and mechanisms. Diabetologia 48(4): 634-642.
- Probst-Hensch N, Yuan J, Stanczyk F, Gao Y, Ross R, et al. (2001) IGF-1, IGF-2 and IGFBP-3 in prediagnostic serum: association with colorectal cancer in a cohort of Chinese men in Shanghai. British journal of cancer 85(11): 1695-1699.
- Muhidin SO, Magan AA, Osman KA, Syed S, et al. (2012) The relationship between nonalcoholic fatty liver disease and colorectal cancer: the future challenges and outcomes of the metabolic syndrome. Journal of obesity 2012: 637538.
- Hwang ST, Cho YK, Park JH, Kim HK, Park DI, et al. (2010) Relationship of non‐alcoholic fatty liver disease to colorectal adenomatous polyps. Journal of gastroenterology and hepatology 25(3): 562-567.
- Lee YI, Lim YS, Park HS (2012) Colorectal neoplasms in relation to non‐alcoholic fatty liver disease in Korean women: a retrospective cohort study. Journal of gastroenterology and hepatology 27(1): 91-95.
- Touzin NT, Bush KN, Williams CD, Harrison SA, et al. (2011) Prevalence of colonic adenomas in patients with nonalcoholic fatty liver disease. Therapeutic advances in gastroenterology 4(3): 169-176.
- Swanson RS, Compton CC, Stewart AK, Bland KI (2003) The prognosis of T3N0 colon cancer is dependent on the number of lymph nodes examined. Annals of surgical oncology 10(1): 65-71.
- Roth AD, Tejpar S, Delorenzi M, Yan P, Fiocca R, et al. (2010) Prognostic role of KRAS and BRAF in stage II and III resected colon cancer: results of the translational study on the PETACC-3, EORTC 40993, SAKK 60-00 trial. Journal of clinical oncology 28(3): 466-474.
- Taieb J, K. Le Malicot, Shi Q, Penault-Llorca F, Bouché O, et al. (2017) Prognostic value of BRAF and KRAS mutations in MSI and MSS stage III colon cancer. JNCI: Journal of the National Cancer Institute 109(5).
- Sarasqueta AF, Forte GI, Corver WE, de Miranda nf, Ruano D, et al. (2013) Integral analysis of p53 and its value as prognostic factor in sporadic colon cancer. BMC cancer 13(1): 1-11.
- Sasahira T, Sasaki T, Kuniyasu H (2005) Interleukin-15 and transforming growth factor alpha are associated with depletion of tumor-associated macrophages in colon cancer. Journal of experimental & clinical cancer research: CR 24(1): 69-74.
- Resnick MB, Routhier J, Konkin T, Sabo E, Pricolo VE (2004) Epidermal growth factor receptor, c-MET, β-catenin, and p53 expression as prognostic indicators in stage II colon cancer: a tissue microarray study Clinical Cancer Research10(9): 3069-3075.
- Poincloux L, Durando X, Seitz JF, Thivat E, Bardou VJ, et al. (2009) Loss of Bcl-2 expression in colon cancer: a prognostic factor for recurrence in stage II colon cancer. Surgical oncology 18(4): 357-365.
- Nehls O, Okech T, Hsieh C, Enzinger T, Sarbia, et al. (2007) Studies on p53, BAX and Bcl-2 protein expression and microsatellite instability in stage III (UICC) colon cancer treated by adjuvant chemotherapy major prognostic impact of proapoptotic BAX. British journal of cancer 96(9): 1409-1418.
- Bilimoria KY, Palis B, Stewart AK, Bentrem DJ, Freel AC, et al. (2008) Impact of tumor location on nodal evaluation for colon cancer. Diseases of the colon & rectum 51(2): 154-161.
- Katoh H, Yamashita K, Wang G, Sato, Nakamura T, et al. (2011) Prognostic significance of preoperative bowel obstruction in stage III colorectal cancer. Annals of surgical oncology 18(9): 2432-2441.
- Otani K, Kawai K, Hata K, Tanaka T, Nishikawa T, et al. (2019) Colon cancer with perforation Surgery Today 49(1): 15-20.
- Zhao Y, Adjei AA (2015) Targeting Angiogenesis in Cancer Therapy: Moving Beyond Vascular Endothelial Growth Factor. The oncologist 20(6): 660-673.
- Radhakrishnan E, Bava SV, Narayanan SS, Nath LR, Thulasidasan AKT, et al. (2014) Anto [6]-Gingerol induces caspase-dependent apoptosis and prevents PMA-induced proliferation in colon cancer cells by inhibiting MAPK/AP-1 signaling. PloS one 9(8): e104401.
- Mohseni M, Park BH (2010) PIK3CA and KRAS mutations predict for response to everolimus therapy: now that’s RAD001.The Journal of Clinical Investigation 120(8): 2655-2658.
- Saletti P, De Dosso S, Frattini M (2015) EGFR signaling in colorectal cancer: a clinical perspective. Gastrointest Cancer 5: 21-38.
- Yang J, Nie J, Ma X, Wei Y, Peng Y, et al. (2019) Targeting PI3K in cancer: mechanisms and advances in clinical trials. Molecular cancer 18(1): 1-28.
- Fruman DA, Chiu H, Hopkins BD, Bagrodia D, Cantley LC, et al. (2017) The PI3K pathway in human disease. Cell 170(4): 605-635.
- Shi X, Wang J, Lei Y, Cong C, Tan D, et al. (2019) Research progress on the PI3K/AKT signaling pathway in gynecological cancer. Molecular medicine reports 19(6): 4529-4535.
- Harbst K, Lauss M, Cirenajwis H, Isaksson K, Rosengren F, et al. (2016) Multiregion whole-exome sequencing uncovers the genetic evolution and mutational heterogeneity of early-stage metastatic melanoma Cancer research 76(16): 4765-4774.
- McGranahan N, Swanton C (2017) Clonal heterogeneity and tumor evolution: past, present, and the future. Cell 168(4): 613-628.
- Uchi R, Takahashi Y, Niida A, Shimamura T, Hirata H, et al. (2016) Integrated multiregional analysis proposing a new model of colorectal cancer evolution. PLoS genetics 12(2): e1005778.
- Yates LR, Gerstung M, Knappskog S, Desmedt C, Gundem G, et al. (2015) Subclonal diversification of primary breast cancer revealed by multiregion sequencing. Nature medicine 21(7): 751-759.
- Cho D, Mier JW, Atkins MB (2009) PI3K/Akt/mTOR pathway: a growth and proliferation pathway in Renal cell carcinoma. Springer Ed pp. 267-285.
- Manning BD, Toker A (2017) AKT/PKB signaling: navigating the network. Cell 169(3): 381-405.
- Jacinto E, Facchinetti V, Liu D, Soto N, Wei, et al. (2006) SIN1/MIP1 maintains rictor-mTOR complex integrity and regulates Akt phosphorylation and substrate specificity. Cell 127(1): 125-137.
- Sarbassov DD, Guertin DA, Ali SM, Sabatini DM (2005) Phosphorylation and regulation of Akt/PKB by the rictor-mTOR complex. Science 307(5712): 1098-1101.
- Vadlakonda L, Dash A, Pasupuleti A. Kotha K, Reddanna P (2013) The paradox of Akt-mTOR interactions. Frontiers in oncology 3: 165.
- Vadlakonda L, Pasupuleti M, Reddanna P (2013) Role of PI3K-AKT-mTOR and Wnt Signaling Pathways in Transition of G1-S Phase of Cell Cycle in Cancer Cells. Frontiers in Oncology 3: 85.
- Ebner M, Sinkovics B, Szczygieł M Ribeiro DW, Yudushkin I (2017) Localization of mTORC2 activity inside cells. Journal of Cell Biology 216(2): 343-353.
- Liu P, Gan W, Chin YR, Ogura K, Guo J (2015) PtdIns (3,4,5) P3-dependent activation of the mTORC2 kinase complex. Cancer discovery 5 (11): 1194-1209.
- Barbosa R, Acevedo LA, Marmorstein R (2000) The MEK/ERK Network as a Therapeutic Target in Human Cancer. Molecular Cancer Research 19(3): 361-374.
- Raghavendra P, Pullaiah T (2018) Chapter 1 - in Advances in Cell and Molecular Diagnostics, Raghavendra P, Pullaiah T, Edn, Academic Press p. 1-32.
- Montagut C, Settleman J (2009) Targeting the RAF-MEK-ERK pathway in cancer therapy. Cancer Lett 283(2): 125-134.
- Gollob JA, Wilhelm S, Carter C, Kelley SL (2006) Role of Raf Kinase in Cancer: Therapeutic Potential of Targeting the Raf/MEK/ERK Signal Transduction Pathway. Seminars in Oncology 33(4): 392-406.
- Chen J, Fujii K, Zhang L, Roberts T, Fu H (2001) Raf-1 promotes cell survival by antagonizing apoptosis signal-regulating kinase 1 through a MEK–ERK independent mechanism. Proceedings of the National Academy of Sciences 98(14): 7783-7788.
- Ma SQ, Cao BR, Zhang H, Luo LP, Ren Y, et al. (2017) The lack of Raf-1 kinase feedback regulation enhances antiapoptosis in cancer cells. Oncogene 36(14): 2014-2022.
- O'Neill E, Rushworth L, Baccarini M, Kolch W (2004) Role of the kinase MST2 in suppression of apoptosis by the proto-oncogene product Raf-1. Science306(5705): 2267-2270.
- Neill EO, Kolch W (2005) Taming the Hippo: Raf-1 controls apoptosis by suppressing MST2/Hippo.Cell Cycle 4(3): 365-367.
- Downward J (2003) Targeting RAS signalling pathways in cancer therapy. Nature reviews cancer 3(1): 11-22.
- Fleming JB, Shen GL, Holloway SE, Davis M, Brekken RA (2005) Molecular consequences of silencing mutant K-ras in pancreatic cancer cells: justification for K-ras–directed therapy. Molecular cancer research 3(7): 413-423.
- Brunner TB, Cengel KA, Hahn SM, Wu J, Fraker DJ, et al. (2005) Pancreatic cancer cell radiation survival and prenyltransferase inhibition: the role of K-Ras. Cancer research 65(18): 8433-8441.
- Pollock CB, Shirasawa S, Sasazuki T, Kolch W, Dhillon AS (2005) Oncogenic K-RAS is required to maintain changes in cytoskeletal organization, adhesion, and motility in colon cancer cells. Cancer research 65(4): 1244-1250.
- Belly RT, Rosenblatt JD, Steinmann M, Toner J, Sun J, et al. (2001) Detection of mutated K12-ras in histologically negative lymph nodes as an indicator of poor prognosis in stage II colorectal cancer. Clinical colorectal cancer 1(2): 110-116.
- Sigismund S, Avanzato D, Lanzetti L (2018) Emerging functions of the EGFR in cancer. Molecular oncology12(1): 3-20.
- Cao Z, Zhang R, Li J, Huang H Zhang D, et al. (2013) X-linked inhibitor of apoptosis protein (XIAP) regulation of cyclin D1 protein expression and cancer cell anchorage-independent growth via its E3 ligase-mediated protein phosphatase 2A/c-Jun axis. The Journal of biological chemistry 288(28): 20238-20247.
- Appiah-Kubi K, Wang Y, Qian H Wu M, Yao X, et al. (2016) Platelet-derived growth factor receptor/platelet-derived growth factor (PDGFR/PDGF) system is a prognostic and treatment response biomarker with multifarious therapeutic targets in cancers. Tumor Biology 37(8): 10053-10066.
- McDermott U, Ames RY, Iafrate AJ, Maheswaran S, Stubbs H P, et al. (2009) Ligand-dependent PDGF receptor-alpha activation sensitizes rare lung cancer and sarcoma cells to PDGF receptor kinase inhibitors. Cancer research 69(9): 3937.
- Steller EJ, Raats DA, Koster J, Rutten B, Govaert KM, et al. (2013) PDGFRB promotes liver metastasis formation of mesenchymal-like colorectal tumor cells. Neoplasia 15(2): 204-217.
- Wehler T, Frerichs K, Graf C, Drescher D, Schimanski K, et al. (2008) PDGFRα/β expression correlates with the metastatic behavior of human colorectal cancer: a possible rationale for a molecular targeting strategy. Oncolog reports 19(3): 697-704.
- Erben P, Horisberger K, Muessle B, Müller MC, Treschl A, et al. (2008) mRNA expression of platelet-derived growth factor receptor-β and C-KIT: correlation with pathologic response to cetuximab-based chemoradiotherapy in patients with rectal cancer. International Journal of Radiation Oncology Biology Physics 72(5): 1544-1550.
- Kitadai Y, Sasaki T, Kuwai T, Nakamura T, Bucana CD, et al. (2006) Targeting the expression of platelet-derived growth factor receptor by reactive stroma inhibits growth and metastasis of human colon carcinoma. The American journal of pathology 169(6): 2054-2065.
- Kitadai Y, Sasaki T, Kuwai T, Nakamura T, Bucana CD, et al. (2006) Expression of activated platelet‐derived growth factor receptor in stromal cells of human colon carcinomas is associated with metastatic potential. International journal of cancer119(11): 2567-2574.
- Kraus C, Rau TT, Lux P, Erlenbach‐Wunsch K, Lohr S, et al. (2015) Comprehensive screening for mutations associated with colorectal cancer in unselected cases reveals penetrant and nonpenetrant mutations. International journal of cancer 136(6): E559-E568.
- Pai SG, Carneiro BA, Mota JM, Costa R, Leite CA, et al. (2017) Wnt/beta-catenin pathway: modulating anticancer immune response. Journal of hematology & oncology 10(1): 1-12.
- Wu C (2018) Systemic therapy for colon cancer. Surg Oncol Clin N Am 27(2): 235-242.
- Kacan T, Nayir E, Altun, Kilickap S, Babacan NA, et al. (2016) Antitumor activity of sorafenib on colorectal cancer. Journal of Oncological Sciences 2(2-3): 53-57.
- Kohne C, Cunningham D, Costanzo FD, Glimelius B, Blijham G, et al. (2002) Gastrointestina tumors-Clinical determinants of survival in patients with 5-fluorouracil-based treatment for metastatic colorectal cancer: Results of a multivariate analysis of 3825 patients. Annals of Oncology 13(2): 308-317.
- Sargent DJ, Kohne CH, Sanoff HK, Bot BM, Seymour MT, et al. (2009) Pooled safety and efficacy analysis examining the effect of performance status on outcomes in nine first-line treatment trials using individual data from patients with metastatic colorectal cancer. Journal of Clinical Oncology 27(12): 1948-1955.
- Gunningham SP, Currie MJ, Han C, Turner K, Scott PA, et al. (2001) Vascular endothelial growth factor-B and vascular endothelial growth factor-C expression in renal cell carcinomas: regulation by the von Hippel-Lindau gene and hypoxia. Cancer research 61(7): 3206-3211.
- Liu L, Cao Y, Chen C, Zhang X, McNabola A, et al. (2006) Sorafenib blocks the RAF/MEK/ERK pathway, inhibits tumor angiogenesis, and induces tumor cell apoptosis in hepatocellular carcinoma model PLC/PRF/5. Cancer research 66(24): 11851-11858.
- Fondevila F, Méndez-Blanco C, Fernández-Palanca P, González-Gallego J, Mauriz JL (2019) Anti-tumoral activity of single and combined regorafenib treatments in preclinical models of liver and gastrointestinal cancers. Experimental & Molecular Medicine 51(9): 1-15.
- Arai H, Battaglin F, Wang J, Lo JH, Soni S, et al. (2019) Molecular insight of regorafenib treatment for colorectal cancer. Cancer Treat Rev 81: 101912.
- Dhillon S (2018) Regorafenib: A Review in Metastatic Colorectal Cancer. Drugs 78(11): 1133-1144.
- Song X, Shen L, Tong J, Kuang C, Zeng S, et al. (2020) Mcl-1 inhibition overcomes intrinsic and acquired regorafenib resistance in colorectal cancer. Theranostics10(18): 8098-8110.
- Fukuoka S, Hara H, Takahashi N, Kojima T, Kawazoe A, et al. (2020) Regorafenib Plus Nivolumab in Patients with Advanced Gastric or Colorectal Cancer: An Open-Label, Dose-Escalation, and Dose-Expansion Phase Ib Trial (REGONIVO, EPOC1603). J Clin Oncol 38(18): 2053-2061.
- Plastaras JP, Kim SH, Liu YY, Dicker DT, Dorsey JF, et al. (2007) Cell cycle–dependent and schedule-dependent antitumor effects of sorafenib combined with radiation. Cancer research 67(19): 9443-9454.
- Suen AW, Galoforo S, Marples B, McGonagle M, Downing L, et al. (2010) Sorafenib and radiation: a promising combination in colorectal cancer. International Journal of Radiation Oncology Biology Physics 78 (1): 213-220.
- Heim M, Scharifi M, Zisowsky J, Jaehde U, Voliotis D, et al. (2005) The Raf kinase inhibitor BAY 43-9006 reduces cellular uptake of platinum compounds and cytotoxicity in human colorectal carcinoma cell lines. Anti-cancer drugs 16(2): 129-136.
- Riley RS, June CH, Langer R Mitchell MJ (2019) Delivery technologies for cancer immunotherapy, Nature reviews. Drug discovery 18 (3): 175-196.
- Rosenberg SA (2014) IL-2: the first effective immunotherapy for human cancer. The Journal of Immunology 192(12): 5451-5458.
- Quesada JR, Hersh EM, Manning J, Reuben J, Keating M, et al. (1986) Treatment of hairy cell leukemia with recombinant α-interferon. Blood 68(2): 493-497.
- Ahmed S, Rai KR (2003) Interferon in the treatment of hairy-cell leukemia. Best Practice & Research Clinical Haematology 16(1): 69-81.
- Alwan LW, Grossmann K, Sageser D, Van Atta J, Agarwal N, et al. (2014) Comparison of acute toxicity and mortality after two different dosing regimens of high-dose interleukin-2 for patients with metastatic melanoma. Targeted oncology 9(1): 63-71.
- Lee S, Margolin K (2011) Cytokines in cancer immunotherapy. Cancers 3(4): 3856-3893.
- Rosenberg SA, Yang JC, Restifo NP (2004) Cancer immunotherapy: moving beyond current vaccines. Nature medicine 10(9): 909-915.
- Kantoff PW, Higano CS, Shore ND, Berger ER, Small EJ, et al. (2010) Sipuleucel-T immunotherapy for castration-resistant prostate cancer. New England Journal of Medicine 363(5): 411-422.
- Hodi FS, O'Day SJ, McDermott DF, Weber RW, Sosman JA, et al. (2010) Improved survival with ipilimumab in patients with metastatic melanoma. New England Journal of Medicine 363(8): 711-723.
- Ribas A, Wolchok JD (2018) Cancer immunotherapy using checkpoint blockade. Science 35(6382): 1350-1355.
- Grupp SA, Kalos M, Barrett D, Aplenc R, Porter DL, et al. (2013) Chimeric antigen receptor–modified T cells for acute lymphoid leukemia. New England Journal of Medicine 368(16): 1509-1518.
- Fesnak AD, June CH, Levine BL (2016) Engineered T cells: the promise and challenges of cancer immunotherapy. Nature Reviews Cancer 16(9): 566-581.
- Couzin-Frankel J (2013) Breakthrough of the year 2013. Cancer Immunother Sci 342 (6165): 1432-1433.
- Vareki SM, Garrigos C, Duran I (2017) Biomarkers of response to PD-1/PD-L1 inhibition. Critical reviews in oncology/hematology 116: 116-124.
- Hay KA, Hanafi LA, Gust DLIJ, Liles WC, Wurfel MM, et al. (2017) Kinetics and biomarkers of severe cytokine release syndrome after CD19 chimeric antigen receptor–modified T-cell therapy. Blood 130(21): 2295-2306.
- Riley RS, Day ES (2017) Gold nanoparticle‐mediated photothermal therapy: applications and opportunities for multimodal cancer treatment. Wiley Interdisciplinary Reviews: Nanomedicine and Nanobiotechnology 9(4): 10.
- Menon S, Shin S, Dy G (2016) Advances in cancer immunotherapy in solid tumors. Cancers 8(12): 106.
- Williams AD, Payne KK, Posey AD, Hill C, Conejo-Garcia J, et al. (2017) Immunotherapy for breast cancer: current and future strategies. Current surgery reports 5: 31.
- Brown CE, Alizadeh D, Starr R, Weng L, Wagner JR, et al. (2016) Regression of glioblastoma after chimeric antigen receptor T-cell therapy. New England Journal of Medicine 375(26): 2561-2569.
- Milling L, Zhang Y, Irvine DJ (2017) Delivering safer immunotherapies for cancer. Advanced drug delivery reviews 114: 79-101.
- June CH, Warshauer JT, Bluestone JA (2017) Is autoimmunity the Achilles' heel of cancer immunotherapy? Nature medicine 23(5): 540-547.
- Wang C, Ye Y, Hu Q, Bellotti A, Gu Z (2017) Tailoring Biomaterials for Cancer Immunotherapy: Emerging Trends and Future Outlook. Adv Mater 29(29).
- Steven A, Fisher SA, Robinson BW (2016) Immunotherapy for lung cancer. Respirology 21(5): 821-833.
- Kennedy LB, Salama AKS (2020) A review of cancer immunotherapy toxicity. CA: A Cancer Journal for Clinicians 70(2): 86-104.
- Pardoll DM (2012) The blockade of immune checkpoints in cancer immunotherapy. Nature Reviews Cancer 12(4): 252-264.
- Alsaab HO, Sau S, Alzhrani R, Tatiparti K, Bhise K, et al. (2017) PD-1 and PD-L1 checkpoint signaling inhibition for cancer immunotherapy: mechanism, combinations, and clinical outcome. Frontiers in pharmacolog 8: 61.
- Munn DH, Bronte V (2016) Immune suppressive mechanisms in the tumor microenvironment. Current opinion in immunology 39: 1-6.
- Blank C, Kuball J, Voelkl S, Wiendl H, Becker B (2006) Blockade of PD‐L1 (B7‐H1) augments human tumor‐specific T cell responses in vitro. International journal of cancer 119(2): 317-327.
- Webb ES, Liu P, Baleeiro R, Lemoine NR, Yuan M, et al. (2018) Immune checkpoint inhibitors in cancer therapy. Journal of biomedical research 32(5): 317.
- Vargas FA, Furness AJ, Litchfield K, Joshi K, Rosenthal R, et al. (2018) Fc effector function contributes to the activity of human anti-CTLA-4 antibodies. Cancer cell 33(4): 649-663. e644.
- Ellis PM, Vella ET, Ung YC (2017) Immune checkpoint inhibitors for patients with advanced non–small-cell lung cancer: a systematic review. Clinical lung cancer 18(5): 444-459. e441.
- Restifo NP, Smyth MJ, Snyder A (2016) Acquired resistance to immunotherapy and future challenges. Nature Reviews Cancer 16(2): 121.
- Dillman RO (2017) Is there a role for therapeutic cancer vaccines in the age of checkpoint inhibitors? Human vaccines & immunotherapeutics 13(3): 528-532.
- Joyce JA, Fearon DT (2015) T cell exclusion, immune privilege, and the tumor microenvironment. Science 348(6230): 74-80.
- Müller L, Aigner P, Stoiber D (2017) Type I interferons and natural killer cell regulation in cancer. Frontiers in immunology 8: 304.
- Sun T, Yang Y, Luo X, Cheng Y, Zhang M, et al. (2014) Inhibition of tumor angiogenesis by interferon-γ by suppression of tumor-associated macrophage differentiation. Oncology Research Featuring Preclinical and Clinical Cancer Therapeutics 21(5): 227-235.
- Enomoto H, Tao L, Eguchi R, Sato A, Honda M, et al. (2017) The in vivo antitumor effects of type I-interferon against hepatocellular carcinoma: the suppression of tumor cell growth and angiogenesis. Scientific reports 7(1): 1-11.
- Cox MA, Harrington LE,Zajac AJ (2011) Cytokines and the inception of CD8 T cell responses. Trends in immunology 32(4): 180-186.
- Yan WL, Shen KY, Tien CY, Chen YA, Liu S (2017) Recent progress in GM-CSF-based cancer immunotherapy. Immunotherapy 9(4): 347-360.
- Uhl M, Aulwurm S, Wischhusen J, Weiler M, Ma JY, et al. (2004) SD-208, a novel transforming growth factor β receptor I kinase inhibitor, inhibits growth and invasiveness and enhances immunogenicity of murine and human glioma cells in vitro and in vivo. 0 Cancer research 64(21): 7954-7961.
- Fu J, Kanne DB, Leong M, Glickman LH, McWhirter SM, Lemmens E, et al. (2015) STING agonist formulated cancer vaccines can cure established tumors resistant to PD-1 blockade. Science translational medicine 7(283): 283ra252.
- Chi H, C Li, Zhao FS, Zhang L, Ng TB, et al. (2017) Anti-tumor activity of toll-like receptor 7 agonists. Frontiers in pharmacology 8: 304.
- Berger C, Berger M, Hackman RC, Gough M, Elliott C, et al. (2009) Safety and immunologic effects of IL-15 administration in nonhuman primates. The Journal of the American Society of Hematology 114(12): 2417-2426.
- Yang JC, Rosenberg SA (2016) Adoptive T-cell therapy for cancer. Advances in immunology 130: 279-294.
- Rosenberg SA, Yang JC, Sherry RM, Kammula US, Hughes MS, et al. (2011) Durable complete responses in heavily pretreated patients with metastatic melanoma using T-cell transfer immunotherapy. Clinical Cancer Research 17(13): 4550-4557.
- June CH, O’Connor RS, Kawalekar OU, Ghassemi S, Milone MS (2018) CAR T cell immunotherapy for human cancer. Science 359(6382): 1361-1365.
- Ahmed N, Brawley VS, HegdeM, Robertson C, Ghazi A, et al. (2015) Human epidermal growth factor receptor 2 (HER2)-specific chimeric antigen receptor–modified T cells for the immunotherapy of HER2-positive sarcoma. Journal of clinical oncology 33(15): 1688.
- Zacharakis N, Chinnasamy H, Black M, Xu H, Lu YC, et al. (2018): Immune recognition of somatic mutations leading to complete durable regression in metastatic breast cancer. Nature medicine 24(6): 724-730.
- Lim WA, June CH (2018) The principles of engineering immune cells to treat cancer. Cell 168(4): 724-740.
- Levine BL, Miskin J, Wonnacott K, Keir C (2017) Global manufacturing of CAR T cell therapy. Molecular Therapy-Methods & Clinical Development 4: 92-101.
- Davila ML, Brentjens RJ (2016) CD19-Targeted CAR T cells as novel cancer immunotherapy for relapsed or refractory B-cell acute lymphoblastic leukemia. Clinical advances in hematology & oncology: H&O14(10): 802.
- Neelapu SS, Locke FL, Bartlett NL, Lekakis LA, Miklos D, et al. (2017) CAR T-cell therapy in refractory large B-cell lymphoma. New England Journal of Medicine 377(26): 2531-2544.
- Maude SL, Laetsch TW, Buechner J, Rives S, Boyer M (2018) Tisagenlecleucel in children and young adults with B-cell lymphoblastic leukemia. New England Journal of Medicine 378(5): 439-448.
- Fitzgerald JC, Weiss SL, Maude SL, Barrett DM, Lacey SF, et al. (2017) Cytokine release syndrome after chimeric antigen receptor T cell therapy for acute lymphoblastic leukemia. Critical care medicine 45(2): e124-e131.
- Rourke DMO, Nasrallah MP, Desai A, Melenhorst JJ, Mansfield K, et al. (2017) A single dose of peripherally infused EGFRvIII-directed CAR T cells mediates antigen loss and induces adaptive resistance in patients with recurrent glioblastoma. Science translational medicine 9(399): eaaa0984.
- Migliorini D, Dietrich PY, Stupp R, Linette GP, Posey AD, et al. (2018) CAR T-cell therapies in glioblastoma: a first look. Clinical Cancer Research 24(3): 535-540.
- Cohen M, Reiter Y (2013) T-cell receptor-like antibodies: targeting the intracellular proteome therapeutic potential and clinical applications. Antibodies 2(3): 517-534.
- Linnemann C, Heemskerk B, Kvistborg P, Kluin RJ, Bolotin DA, et al. (2013) High-throughput identification of antigen-specific TCRs by TCR gene capture. Nature medicine 19(11): 1534-1541.
- Peggs K, Quezada S, Allison J (2009) Cancer immunotherapy: co‐stimulatory agonists and co‐inhibitory antagonists. Clinical & Experimental Immunology 157(1): 9-19.
- Croft M (2003) Co-stimulatory members of the TNFR family: keys to effective T-cell immunity? Nature Reviews Immunology 3(8): 609-620.
- Tolcher AW, Sznol M, Hu-Lieskovan S (2017) Papadopoulos KP A. Phase Ib study of utomilumab (PF-05082566), a 4-1BB/CD137 agonist, in combination with pembrolizumab (MK-3475) in patients with advanced solid tumors. Clinical Cancer Research 23(18): 5349-5357.
- Segal NH, Logan TF, Hodi FS, McDermott D, Melero I (2017) Results from an integrated safety analysis of urelumab, an agonist anti-CD137 monoclonal antibody. Clinical Cancer Research 23(8): 1929-1936.
- Buchan SL, Rogel A, Al-Shamkhani (2018) A The immunobiology of CD27 and OX40 and their potential as targets for cancer immunotherapy. The Journal of the American Society of Hematology 131(1): 39-48.
- Zhang P, Gao F, Wang Q, Wang X, Zhu F, et al. (2007) Agonistic anti‐4‐1BB antibody promotes the expansion of natural regulatory T cells while maintaining Foxp3 expression. Scandinavian journal of immunology 66(4): 435-440.
- Zhang Y, Li N, Suh H, Irvine DJ (2018) Nanoparticle anchoring targets immune agonists to tumors enabling anti-cancer immunity without systemic toxicity. Nature communications 9(1): 1-15.
- Guo C, Manjili MH, Subjeck JR, Sarkar D et al. (2013) Therapeutic cancer vaccines: past, present, and future. Advances in cancer research 119: 421-475.
- Garg AD, Coulie PG, Van den Eynde BJ, Agostinis P (2017) Integrating next-generation dendritic cell vaccines into the current cancer immunotherapy landscape. Trends in immunology 38(8): 577-593.
- Pardi N, Hogan MJ, Porter FW, Weissman D (2018) mRNA vaccines-a new era in vaccinology. Nature reviews Drug discovery 17(4): 261.
- Yang B, Jeang J, Yang A, Wu TC, Hung CF (2014) DNA vaccine for cancer immunotherapy. Human vaccines & immunotherapeutics 10(11): 3153-3164.
- Li L, Goedegebuure S, Gillanders WE (2017) Preclinical and clinical development of neoantigen vaccines. Annals of Oncology 28(suppl_12): xii11-xii17.
- Kather JN, Halama N (2019) Harnessing the innate immune system and local immunological microenvironment to treat colorectal cancer. British Journal of Cancer 120(9): 871-882.
- Yang Y (2015) Cancer immunotherapy: Harnessing the immune system to battle cancer. The Journal of clinical investigation125(9): 3335-3337.