An Overview on Aquatic Unmanned Aerial Vehicles
Qiu Suming1,2 and Cui Weicheng2*
1College of Civil Engineering and Mechanics, Lanzhou University, China
2Deep Sea Technology Research Center, School of Engineering, Westlake University, China
Submission: November 06, 2019;Published: November 19, 2019
*Corresponding author: Cui Weicheng, Deep Sea Technology Research Center, School of Engineering, Westlake University, China
How to cite this article: Qiu Suming, Cui Weicheng. An Overview on Aquatic Unmanned Aerial Vehicles. Ann Rev Resear. 2019; 5(3): 555663. DOI: 10.19080/ARR.2018.04.555663
Abstract
Aquatic unmanned aerial vehicles (aquatic UAVs) are latest progresses broadening the application prospects of UAVs and autonomous underwater vehicles (AUVs). However, physical differences between air and water make a fully functional aquatic UAV hard to be designed. This paper analyzes difficulties in shape design, wing design, cross-domain design, take-off and landing design, etc., and discusses some insightful solutions from the perspectives of AUVs and UAVs. Furthermore, this paper pays more attentions to bio-inspired aquatic UAVs and discusses some promising conceptual designs in the end of the paper.
Keywords: Unmanned Aerial Vehicle (UAV); Autonomous Underwater Vehicle (AUV); Biomimetics; Cross-Domain Design; Aquatic UAV
JEL Classification: P23, Q52, Q54.
Abbreviations: Annals of Reviews & Research; Juniper Publishers Open Access Journal; Reviews Research Paper; Research & Reviews Journal of Life Sciences; Research & Reviews a Journal of Health Professions; research & Reviews a Journal of Bioinformatics; Research & Reviews in Food & Nutrition Science; Research & Reviews Medical and Clinical Oncology; Annual Reviews Materials Research, Scientific Research Articles; Juniper Publishers Review
Introduction
Since Wright Brothers’ first flight, aerial vehicles have been improved rapidly. Because manned vehicles are usually difficult to design due to complex layout, high cost, and low efficiency, unmanned vehicles like unmanned aerial vehicles (UAVs) and unmanned underwater vehicles (UUVs) developed rapidly and separately in the past several decades. There are two main types of UUVs, i.e., AUVs and remotely operated vehicles (ROVs). UAVs and AUVs comprise a large part of unmanned vehicles. In order to expand working domains of unmanned vehicles, aquatic UAVs have been developed. Easy transitions between air and water make them flexible and latent. There are many problems in the design of aquatic UAVs. For instance, in the air, the power demand of an aquatic UAV is usually large because it has to overcome its gravity, so diesel engines are suitable for UAVs; while in the water, the gravity can be offset by the buoyancy so the electric engine can satisfy the needed power; then using which type of engines becomes a problem. A partially functional aquatic UAV is usually hard to design, and more problems like the communication problems, fuselage shape problems, and design of the switching algorithms will occur if it is a fully functional aquatic UAV. There are some other names like amphibious UAV [1] and unmanned aerial-aquatic vehicle [2], but we use aquatic UAV in this paper to define those unmanned vehicles which can fly in the air and in the water.
Many innovations to solve design problems of aquatic UAVs are bio-inspired, because many animals are able to dive and fly, like gannets, flying fish and cormorants [3-7]. But how to maintain a long time travelling in both domains is still an unsolved problem. Before reviewing the developments of aquatic UAVs, this paper first briefly introduces the developments of UAVs and AUVs separately. Then this paper discusses problems in the design of aquatic UAVs and give some bio-inspired solutions. In the end, this paper discusses some promising conceptual prototypes. The rest of this paper is arranged as follows. Section 2 is the introduction to potential applications of aquatic UAVs. Section 3 introduces developments and main types of AUVs, and Section 4 describes developments and main types of UAVs. Section 5 introduces two categorizing methods for aquatic UAVs. Section 6 discusses some designing problems including control problems, take-off and landing problems, and communication problems. Section 7 shows some promising conceptual designs and Section 8 makes a summary on the key problems of designing an aquatic UAV.
Potential applications of aquatic UAVs
There are numerous applications for aquatic UAVs. In agriculture, they can be used to spray chemicals, monitor the growth of plants, do weed surveillance, and drive away birds [8,9]. A variety of sensors can be installed on them to measure elements undersea such as reflection of light and presence of microscopic life [10-12]. Under-ice working is very dangerous, but with aquatic UAVs, it will be easier to observe the creatures and inspect under-ice situations [13,14]. Moreover, these are single-domain applications, so they cannot show the crossdomain advantages of aquatic UAVs. Here are some unique applications of aquatic UAVs.
a) Water sampling is usually difficult, particularly for large number of samples in a large area (Figure 1). UAVs can do a sampling task relatively easily [15-17]. Water sampling tasks done by UAVs are limited to shallow water, while using aquatic UAVs, deep water sampling tasks can be done. With aquatic UAVs, one location’s water samples from different depths and the seafloor can be collected. When one location’s samples are collected, aquatic UAVs can fly to other locations quickly, making sampling tasks more efficient.
b) Aquatic UAVs will be used as transport mechanisms too. They can transport things in many ways, as they can fly and dive. The cooperative transport systems will be important for ocean oil pipes’ transportations [18] (Figure 2).
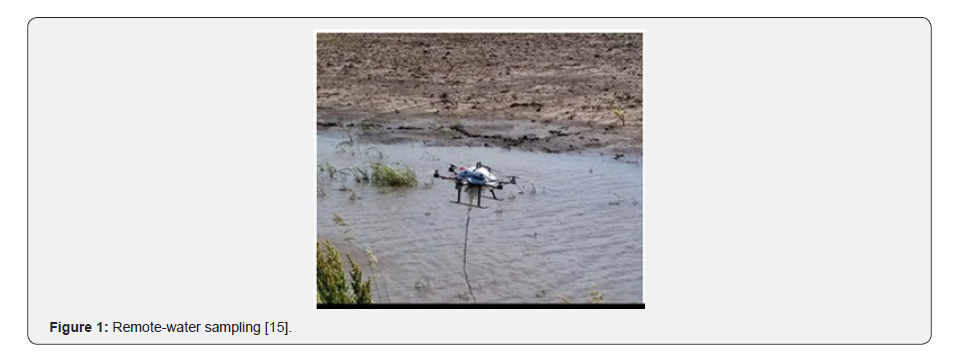
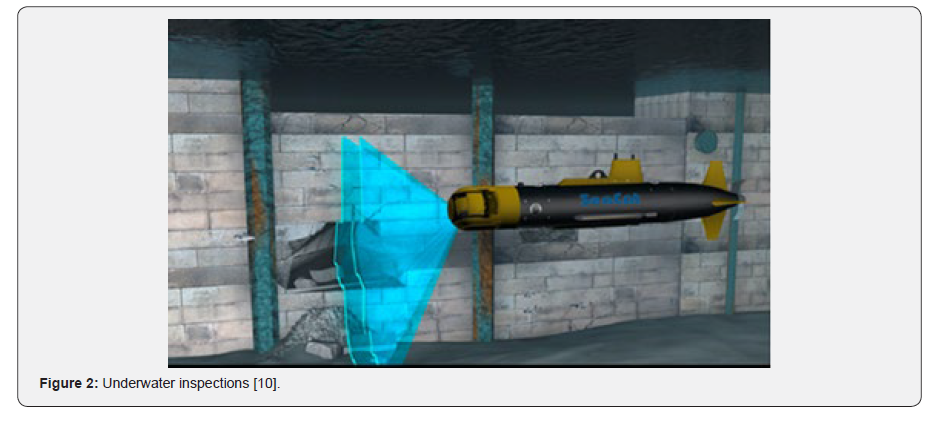
Technical introduction to AUVs
AUVs are instrument carriers, with self-contained propulsion, sensors, and intelligence, enabling them to complete missions without human interventions [19]. ROVs are controlled by operators or pilots via an umbilical or remote control, and most ROVs are tethered. In contrast, AUVs are untethered [19]. AUVs constitute a large part of UUVs and UUVs account for a large part of submersibles. There are two main types of submersibles, i.e., UUVs and human-occupied vehicles (HOVs). For UUVs, there are four main types, i.e., AUVs, gliders, hybrid ROV and AUV (HROVs), and remotely operated vehicles (ROVs). There are four types of HOVs, i.e., manned submersibles, atmospheric diving suits, rescue bells, and deep submergence rescue vehicles. Figure 3 is a brief division of submersibles [20].
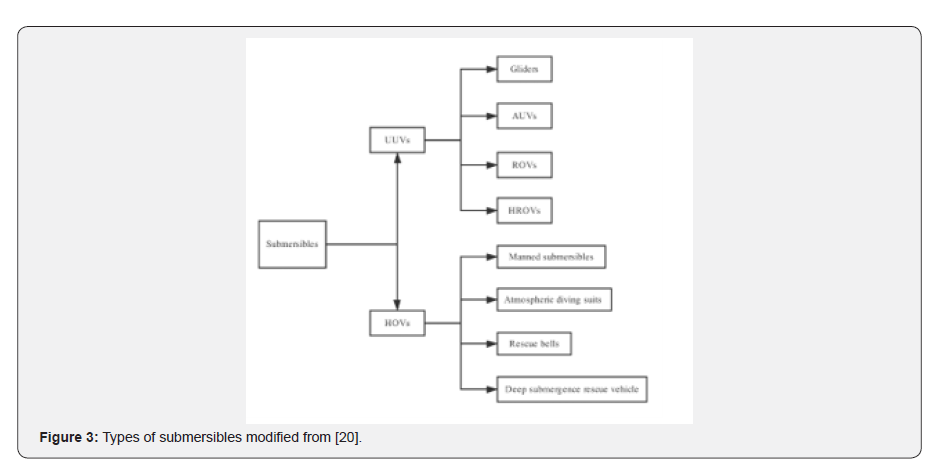
Development history of AUVs
The first AUV was developed at Applied Physics Laboratory at University of Washington in 1957 [21] which is called “special purpose underwater research vehicle”, or SPURV. It is used to study diffusion, acoustic transmission, and submarine wakes. There are many developments since SPURV was developed, but only three AUVs, ALIVE, SAUVIM, and GIRONA 500 have demonstrated autonomous intervention capabilities till 2015 [21]. ALIVE is equipped with two hydraulic grasps for docking and a 7 degrees of freedom (DOF) manipulation arm, and able to carry out a manipulation action consisting of opening and closing a valve in a sub-sea panel autonomously. The arm and fuselage have no interactions, so the manipulation is an underwater single arm manipulation [21]. SAUVIM is carried out at the Autonomous System Laboratory of University of Hawaii and built around an open-framed structure enclosed by a flooded composite fairing, and the movement is controlled by thrusters around the mass center [22]. Unlike ALIVE, the free-floating-manipulation concept of SAUVIM is paid more attentions to. SAUVIM is equipped with a 7 DOF robotic arm to achieve operating capabilities. Actuated by electromechanical components, the robotic arm has higher accuracy and lower requirements of power in manipulation (Figure 4).
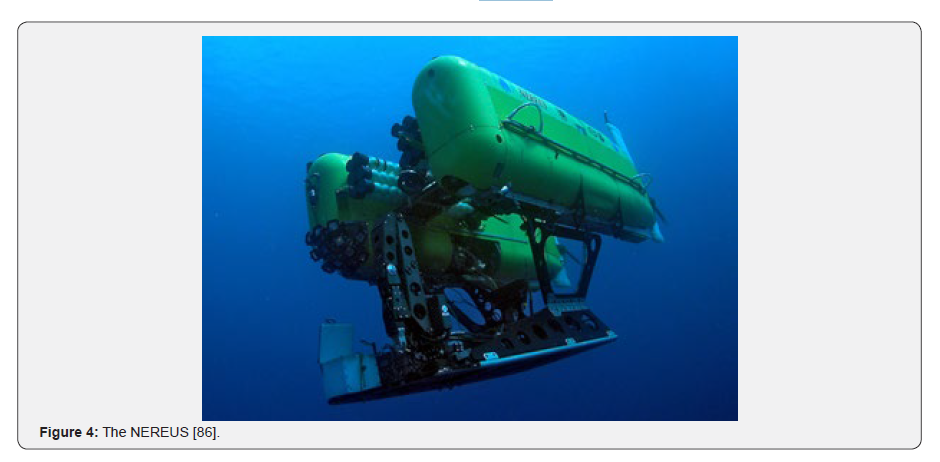
GIRONA 500 (Figure 5) is a compact-size AUV designed for a maximum operating depth of 500 m by University of Girona. The dimensions of the AUV are 1 m in height, 1 m in width, 1 m in length, and a weight of 140 kg, making it lighter than ALIVE and SAUVIM. The most remarkable characteristic of GIRONA 500 is the capacity to reconfigure for different tasks; typical sensors like DVL, pressure gauge, etc., are installed, and besides these sensors, almost half of the volume is reserved for missionspecific sensors and equipment, making it possible to modify sensing and actuation capabilities for different tasks [21]. In the last few decades, besides these three representative AUVs, some biomimetic AUVs have also been developed, such as boxfish-like AUVs [23], lobster-like AUVs [11,12], turtle-like AUVs [24], and so on. They mimic the unique structures possessed by underwater creatures. For instance, a squid rocket-like AUV can eject carbon dioxide to make a fast move in the water, which can also be used in the water-exit process [25].
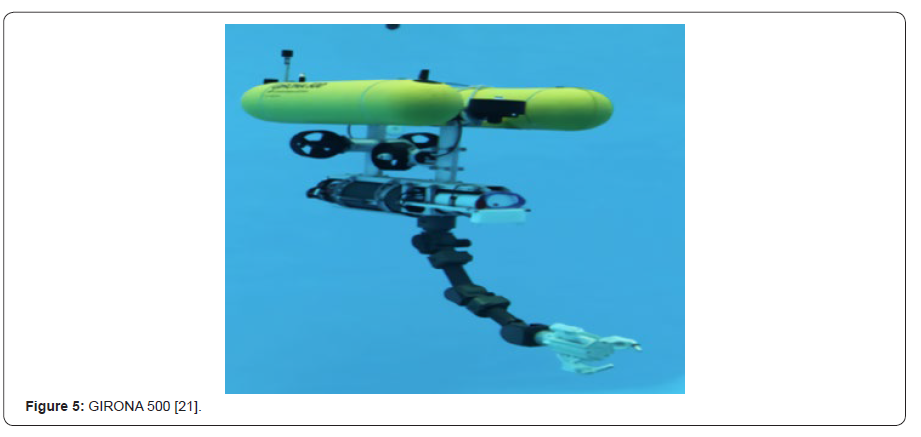
Design of AUV
Shape Design: AUV’s shape design is an essential part. Because of the fluid-structure interaction with water surrounding the fuselage, the shape of the AUV affects the dynamics of motions. Most of AUVs are torpedo-shaped, and some analytical computations of torpedo-shaped AUVs are done in [26]. An optimal shape design for a torpedo shaped AUV is listed in [27] and the optimized design is a streamlined shape without abrupt changes in curvature, so flow separation is very little which also represents a substantial increase of the autonomy of the vehicle. Designers have always been working on designing a shape that can decrease the drag and increase the hydrodynamic stability of the vehicle. Designers look into ocean animals for inspirations because many ocean animals have good streamlined and lowdrag shapes [24,28,29]. Catfish has a special low-drag shape and numerical analyses show that this shape can reduce the drag and increase the stability significantly [30], a catfish-shaped hull is proposed in this paper

Power Problems: When operating underwater, diesel engines cannot get enough oxygen to provide power, so most of AUVs use electric engines or hybrid-powered engines. When using batteries, how many batteries are needed for a task and how long can one battery sustain should be carefully calculated [26,31,32]. Another important reason to abandon diesel engines is the noise produced by them which can disclose AUVs’ locations. Wireless charging is another advantage of electric engines. A wireless charging system and the corresponding control algorithms are proposed in [33]. Different AUVs can be charged through electromagnetic waves produced by other AUVs. With this method, the charging process can be done underwater, although the efficiency of this method is low. Some of the AUVs go to mothership to charge the batteries and turn back to working areas to charge AUVs in low battery period wirelessly, then these AUVs can work for a very long time. If a solar cell is attached to each vehicle, these AUVs can operate longer. There is an interesting way to save energy when you need to move around. The remora suckerfish can move to many places by attaching themselves to the bodies of other animals with the disc on their heads (Figure 6a), which can be used on AUVs as well because this will save a large amount of energy when moving a large distance. An AUV with biorobotic adhesive disc is developed to move around quickly with the help of other fish [34] (Figure 6b), but the drawback is that you cannot choose where to go.
Technical Introduction to UAVs
A UAV is aircraft without a pilot on board and a type of unmanned vehicles. UAVs are components of an unmanned aircraft system (UAS), including UAVs, ground-based controllers, and a system of communications between them [35]. The flight of UAVs may operate with various degrees of autonomy: either under remote control by a human operator or autonomously by onboard computers.
Main Types of UAVs
UAVs can be divided into two types, i.e., lighter than air UAVs, which are lifted by buoyancy, and heavier than air UAVs, lifted by relative motion against the air [35].Lighter than Air UAVs: UAVs lighter than air include airships, balloons, etc., the main parts of them are airbags filled with a gas lighter than air such as helium, hydrogen, or hot air. Owing to the weight of air they displace is bigger than their own mass so they can fly.
Heavier than Air UAVs: Heavier-than-air UAVs should find ways to push air or gas downwards to produce reactions to push the aircraft upwards. There are two ways producing dynamic up-thrust, i.e., aerodynamic lift and powered lift in the form of engine thrust. Aerodynamic lift is one of the most common ways to produce up-thrust. To fly, air must flow over the wings to generate lift. Fixed wings UAVs and rotorcrafts usually use this way to generate lift. Powered lift is produced by directing the engine thrust vertically downward. Harrier Jump Jet uses this way to generate lift. Heavier than air UAVs usually have three main kinds: fixed wings UAVs (planes, gliders), rotor wings UAVs (helicopters) and flapping wings UAVs. A division of UAVs is illustrated in Figure 7.
Design of UAV
Power problem is important for UAVs and it has been discussed in Section 3.2. Because it is almost the same for UAVs, so we do not lay much emphases on it in this section. The wing design and hull design are quite different from those in Section 3.2. In the air, we usually need a large wing to provide buoyancy while underwater this design will bring considerable resistances. Because of the different physical properties between air and water, the layouts of an AUV and a UAV can vary a lot too.
Shape Design: Shape design is vital for UAVs. A welldesigned one will shorten the take-off distance significantly. Torpedo shapes can satisfy most needs, and there are many numerical analyses on torpedo design [36]. But this does not lessen the importance of shape design because a badly designed shape might increase the power requirements for the engines, even making UAVs fly at a low speed. To design a good shape, aerodynamic drag is important. It is a function combining frontal area, velocity, air density, and drag coefficient. During the take-off process, the angle, density and the aerodynamic drag coefficient can be considered as constants, so the aerodynamic drag becomes a function of velocity [37], making it difficult to design shapes of UAVs. Researches have been carried out to design a shape which can reduce the drag a lot. A good way to design low-drag body is to use natural design. Some birds’ lowdrag bodies fit the aerodynamics needs. Which can be used as reference for UAVs shape design. By scanning their bodies and making models, researchers can get a primary fuselage design. Then using computational fluid dynamics (CFD) method to verify whether the qualities of the scanned model meet the need and make some adjustments. A duck’s body was scanned by special machines in [38], and they calculate the aerodynamic properties of the model as well, which can inspire us a lot.
Wing Design: UAVs can be divided into four main types by wings, i.e., quadcopters UAVs, fixed wings UAVs, morphing wings UAVs, and flapping wings UAVs. Both aquatic UAVs and UAVs share the same categorizing method, so we do not discuss them here, we will discuss them in detail in Section 5.
Development of UAVs
UAV has experienced a long period of development. From fixed wings UAVs to rotor wings UAVs and to quadcopters UAVs. Nowadays designs of UAVs vary a lot. Figure 8 combines some of the information from [39-41], and it shows the development history of the UAVs.

Technical Introduction to Aquatic UAVs
Until now, a fully featured aquatic UAV has not been produced yet, but many partially featured aquatic UAVs have been designed by many universities and companies. Some technologies like autonomous control in two kinds of fluid media (air and water), structure and layout design which take hydrodynamics and aerodynamics into considerations, etc., are developed. According to launching modes and the operation environments, aquatic UAVs can be categorized into three types, i.e., seaplane aquatic UAVs, submarine-launched aquatic UAVs, and submersible aquatic UAVs [42]. When categorized by wings’ structures, there are four types, i.e., fixed wings aquatic UAVs, morphing wings aquatic UAVs, quadcopter aquatic UAVs, and flapping wings aquatic UAVs. We will discuss about three launching ways in Section 5.1 and four types of wings in Section 5.2. The waterentry and water-exit processes will be discussed in Section 5.3.
Three launching ways
Table 1 summarizes working domains and working forms of three kinds of aquatic UAVs and some of the information is taken from [42].

Seaplane Aquatic UAVs: Seaplane aquatic UAVs can work on the water surface and in the air. They can launch and land on the surface of the water. One famous type of seaplane aquatic UAVs is the GULL from the UK. The developments of seaplane aquatic UAVs are shown in Figure 9. Seaplane aquatic UAVs have three main features:
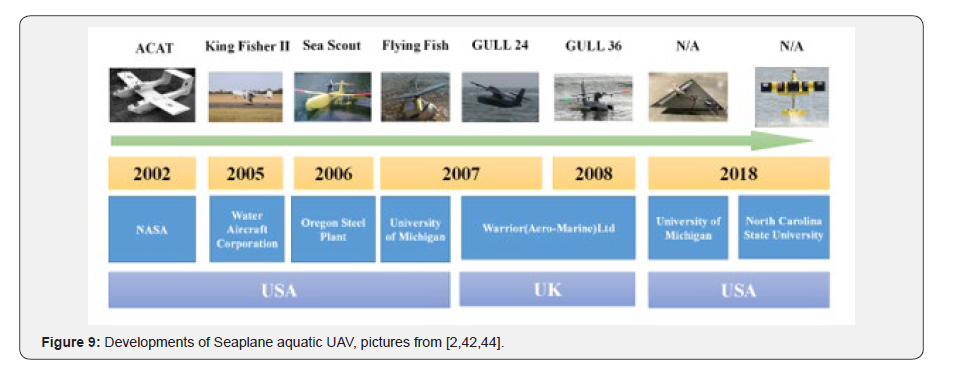
i. Seaplane aquatic UAVs have float mechanisms to ensure that the bodies can be separated from the water and reduce the frictions when launched. But these mechanisms may add frictions when seaplane aquatic UAVs fly in the air.
ii. The most used launching way of seaplane aquatic UAV is sliding take-off.
iii. The working areas of seaplane aquatic UAVs are the water surface and the air [43].

Submarine-Launched Aquatic UAVs: Submarine-launched aquatic UAVs are launched by submarines to commit missions of spying in the air, target-locating, and communications. There are two types of launching ways, i.e., wet launch and dry launch. The dry launch is a way that submarine emits a missile equipped with UAVs or using a special launcher system to let the UAV out. The wet launch uses buoyancy mechanisms or propellers to complete. When submarine’s launching tube is open, UAVs can get out with their propellers. The developments of submarinelaunched aquatic UAVs are shown in Figure 10 and they have three main features:
a) Two different launching ways.
b) Most of their wings are variable, making them easier to be stored, transmitted and retrieved.
c) Most of aquatic UAVs work in the air. Except for the launching and retrieving processes, they do not get touch with water [42].
Submersible Aquatic UAVs: Submersible aquatic UAVs can work underwater and fly in the air. Compared with seaplane aquatic UAVs, submersible aquatic UAVs can extend their working areas into water. Compared with submarine-launched aquatic UAVs, they have a stronger independent working ability. Their launching and landing process can be done without carriers. The developments of submersible UAVs are shown in Figure 11. They have two features:

a) Submersible aquatic UAVs can work underwater and in the air.
b) Variable wings’ structures include folded swept wings and bio-inspired flapping wings. These changeable wings are designed to reduce frictions and improve moving efficiency in the water [42,43].
Four Kinds of Wings
Fixed Wings Aquatic UAVs: For fixed wings aquatic UAVs, the lift of the body can be used to assist in exiting the water, which can reduce the amount of needed power [44]. In 2002, NASA made a prototype fixed wings UAV called autonomous cargo amphibious transport (ACAT), it can take off and land on ground and the water surface [1, 2]. In 2007, a fixed wings aquatic UAV called flying fish was developed (Figure 12a). It can take off and land on the water autonomously but cannot dive into the water [45-47]. From 2007 to 2012, researchers had been working on improving it. The path planning, fault-tolerant air data system, and aerodynamic configuration are studied in detail. It has two navigation modes, namely the sea-wave mode and the flying mode. To save energy, engines would be cut in the sea-wave mode. When batteries run out, they can float on water surface and use solar cells on the wings to charge the batteries. Flying fish is an attempt to achieve the goal of “persistent ocean surveillance” [48,49]. In 2018, a fixed wings UAV developed by Michigan University (Figure 12b), almost achieved the goal of aerial and underwater working as well as the cross-domain goal. It can manage buoyancy passively by using floodable compartments in the fuselage, allowing neutral buoyancy in the water and minimizing the vehicle weight at the same time. Their work has characterized a single fixed wings vehicle capable of aerial and underwater operations [44]. In 2018, another fixed wings UAV (Figure 12c), which was developed by Joseph, achieved the cross-domain goal too. A successful water-to-air transition using an electric motor and single propeller is done, through the use of positive buoyancy. Because of the lifting surface, it could achieve greater endurance than quadcopter UAVs [2].
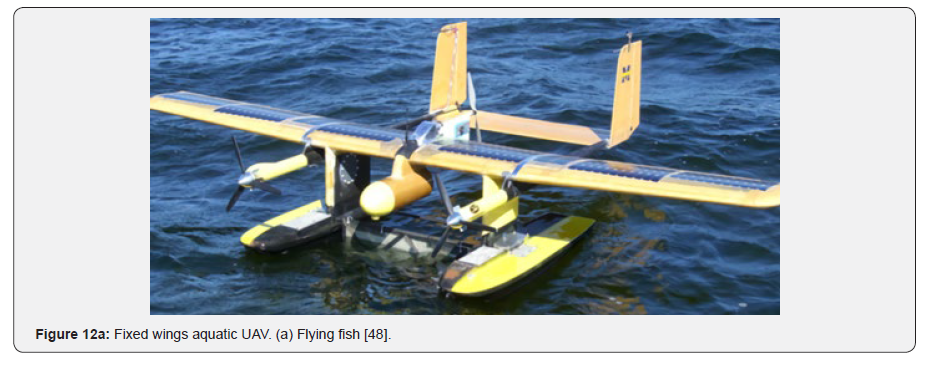
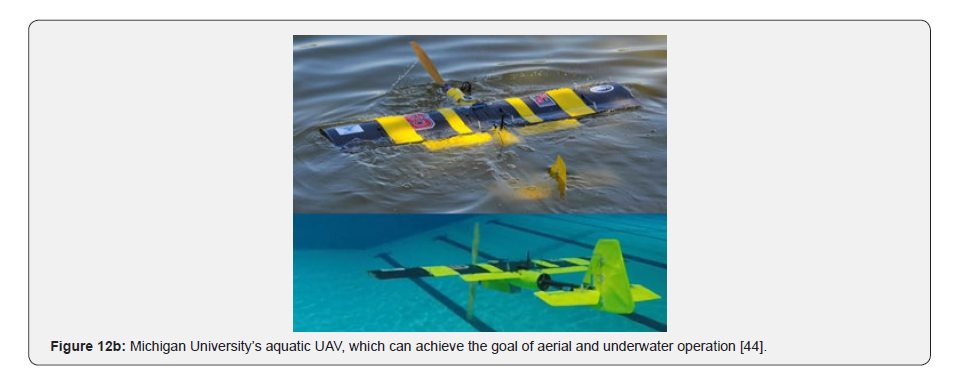
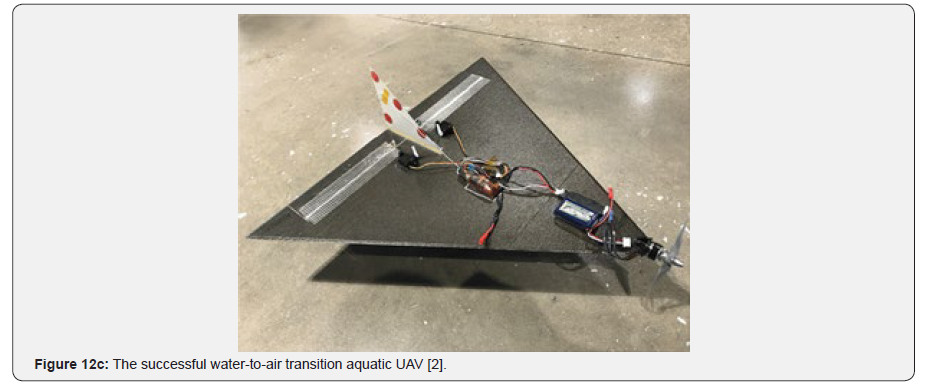
Morphing wings aquatic UAVs: In the late 1950s and early 1960s, the XB-70 (Figure 13a,b,c,d) supersonic bomber successfully incorporated out-of-plane wing morphing. The XB-70 outer wing panels folded downward almost 30 degrees to increase L∕D (lift/drag) at both low subsonic and supersonic speeds. The XB-70 exceeded Mach 3 and operated in an extremely high-temperature environment. Although the weight for the structural fittings and actuators required several tons of material, the design of XB-70 remains the most successful multi- Mach number, out-of-plane morphing wings surface [50]. And later Lockheed company made another morphing wings UAV, which can change its configurations (Figure 13b) and wing areas by taking the inner wing panels into the fuselage. This design enables it to achieve high-speed moving [50]. They can also give inspirations in aquatic UAVs.
In 2014, Beihang University built a submersible unmanned flying boat, which can fold back its wings to increase underwater maneuverability. In 2016, R. Siddall et. al. developed a morphing wings aircraft and its wings can rotate around the pivot from 0-90 degrees. In 2019, MIT produced a bimodal UAV and the wings of which can be folded back 65 degrees to achieve low drag for underwater cruise and a high aspect ratio for long endurance airborne (Figure 13c). This bimodal UAV also involves an integrated propulsion system comprising with a compressed gas thruster to get out of the water [51]. The UAV produced by Beihang University in 2014 is called mini-gannet (Figure 13d) which mimicked the morphology of a living gannet and the folding wings were realized by variable swept back wings. The pivot point of the wing root is a vital element of the UAV and must have the adequate safety of bearing the impact load. Its strength, stiffness, functional reliability and antiknock qualities are extremely complicated, because the variable swept-back wings constrain all of the aerodynamic loads through the pivot and produce a massive concentration of stresses [52]. Last but not least, some morphing wings can also be used as a jumping mechanism. In [53], the UAV can jump and glide using the morphing wings.

Quadcopter Aquatic UAVs:
There are many quadcopter UAVs which can operate in the water and in the air. The quadcopter UAVs generate lift through rotations of multiple rotors. The flight is stable, and the maneuverability is good, but quadcopter aquatic UAVs have low energy efficiency and short battery life. Compared with other UAVs, quadcopter UAVs are relatively easy to enter and exit the water, but the propellers should not touch the water surface. Once touched, the speed is reduced, which will cause the UAV to violently shake and fall into the water. There are two designs to ensure that aquatic UAVs get out of the water safely and swiftly, i.e., double-layers wings and single-layer wing.
Single-Layer Aquatic UAVs
One question connected with quadcopter aquatic UAVs is that whether the air propellers can be used underwater for propulsions as well. In [54], the authors proposed a preliminary feasibility study of using a single set of aerial fixed-pitch aerial propellers underwater. They have tested a wide range of pitch angles for aerial propellers that otherwise have the same diameter and aerodynamic profile. The experimental results showed that the aerial propellers can operate effectively underwater at low rotation speed (around 180 rpm) without cavitation, providing enough thrust for movement and maneuvering while still providing high thrust to power ratio in the air. Suitable sized propellers have been selected to meet the requirements in the ongoing development of an aerial quadrotor with underwater capabilities.
Auckland University developed a single layer UAV called Looncopter (Figure 14). An adjustable buoyancy device was used to adjust the buoyancy of the aircraft so that the UAV can float on the water surface steadily and the blades will not be affected by the water. The aircraft uses a single row of propellers beyond or below the surface of the water, and the depth is adjusted by a depth adjustment system consisting of a water depth sensor and a water discharge buoyancy device. The speed of the propellers is enough to meet the underwater motion of the aircraft. Detailed data and control algorithms were shown in [55,56]. Some other papers have also discussed the feasibility to build a single layer aquatic UAV [57,58].
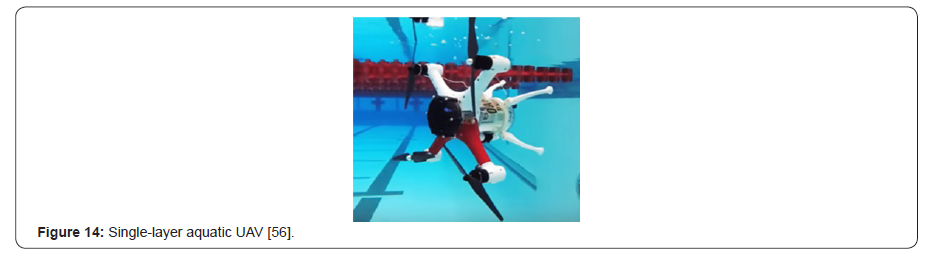
Double Layers Aquatic UAVs
Rutgers University developed a double-layers aquatic UAV called Navigator (Figure 15). When floating on water surface, the low row of blades slowly rotates, so that the UAV is in a favorable take-off attitude on the water surface. The eight propellers all use small-sized air propellers, which can better achieve air flight, underwater navigation, and water-free seamless crossing. However, due to water and air communication obstacles, Navigator needs to be connected to the water surface signal receiving device through cables. Low flight speed and poor load capacity are two challenges. More detailed double-layer UAVs are shown in [56,58].
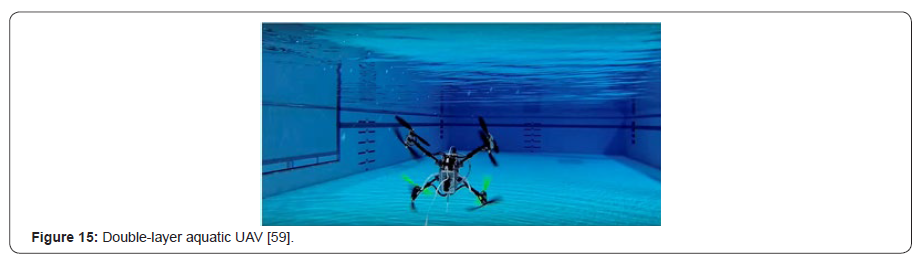
Flapping Wings Aquatic UAVs
Most of the flapping wings aquatic UAVs mimicked natural creatures. In 2019, Harvard’s Wyss Institute launched a quadrotor bionic robot (Figures 16a & b). At first, it was powered by lithium-ion battery, which has weighed more than 4 times of the vehicle’s mass. The present batteries are insufficient for the vehicle. Solar cells, however, offer a promising alternative and have been used in a number of autonomous vehicles. The new design lifts the efficiency up to 30% and enables the vehicle to work for 3 minutes with a load of 370 mg. The robot utilizes bimorph piezoelectric actuators to actively control the wings stroke motion, while the wing pitch motion is passively mediated by a polyimide flexure [47]. To verify whether the UAV can achieve aerial-aquatic qualities, some researches were carried out. By comparing flapping flight in the water and air through 3D CFD simulations and experiments, they found that favorable passive pitching can be achieved using the identical wing hinge design no matter in the air or in the water [46].
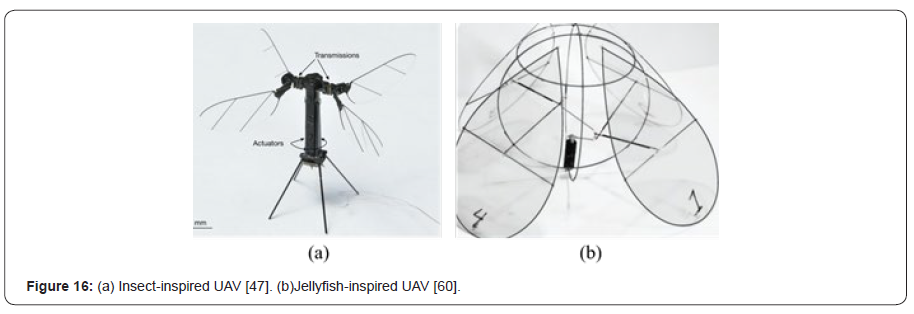
A jellyfish-inspired flapping wings aquatic UAV was also designed (Figure 16b). The main body is made of carbon fiber, and the wings are made of plastics [59]. The design is based on an alternative concept that exhibits intrinsic stability using flapping wings alone. Unlike back-and-forth motions adapted in other robots, this scheme of in-and-out motions provides the strong damping of the body motions needed for stability. Four plastic wings can keep it upright without sensors or feedback controls, while other insect-shaped aquatic UAVs need smart sensors and complex algorithms to keep balance. Because of their layouts, they can realize motions underwater as well. It is also instructive to compare and contrast the design with the jellyfish’s counterparts to make more advancements [60]. There is also another insightful jellyfish design. A tissue-engineered jellyfish was developed, and the combination of algorithms with physiological performance suggests that this strategy can be applied to simple life forms that pump to survive [61]. Although this tissue-engineered jellyfish’s swimming movement is limited to one stereotypic mode of execution because of the lack of muscle’s fine-controlling, and it can only work under water; we can still use this prototype as an inspiration to develop tissueengineered aquatic flapping wings UAVs.

In 2010, a fapping wings aquatic UAV mimicked guillemot was designed aiming for aerial and underwater propulsion. Through the platform test, researchers found that the sweptback foil reduces the needed power comparing to an extended foil. They also found that key mechanisms utilized by the common guillemot and other avian animals capable of aerial and aquatic locomotion are the retraction of the wing reducing the overall wing planform area and moment arm about which the wing is flapped [62,63]. This can be applied for further flapping wings UAVs’ developments. Another good example of flapping wings is batoid-shaped wings [28]. This kind of wings have high maneuverability and energy efficiency underwater. There are also many good designs [64,65,29] and we do not make more detailed introductions.
Quadcopter’s Water-air Transition (Entry and Exit Processes)
For a single-layer quadcopter aquatic UAV, the main challenge in the water-air transition process is how to keep blades away from water. The blade spins at high speed and once touched with water, the vehicle will lose balance and drop into the water. A single-layer UAV can use an adjustable buoyancy mechanism to maintain balance [66,67]. The design of the aquatic UAVs has to take modes transferring algorithms of the rotors, the lift and drag coefficients in both media, and ballast system into considerations. The first quadcopter aquatic UAV is called Loon Copter and it achieved a novel seamless cross-domains transitions using the ballast system which improves the vehicle’s stability underwater and reduces the power consumption [55]. There is also a detailed experiment of the transition in [57]. For a double-layers quadcopter aquatic UAV, the way to maintain balance is simple. During the water-air transition process, as shown in Figure 17, the top layer will spin faster to provide the thrust to get out of the water and the lower layer of the blades will rotate slowly to provide buoyancy rather than use an adjustable buoyancy mechanism [56]. And during the air-water transition, the fuselage can dive into the water directly if it is heavier than water [59].
Another most frequently used way of diving is plunge-diving inspired by gannets. Gannets can plummet from 30 m into the water to catch fish, so a gannet-like UAV is developed. Some numerical models are established in [68,69] and a CFD based method is used to verify the stability of the model (Figures 18ad). There are three phases in the diving process.
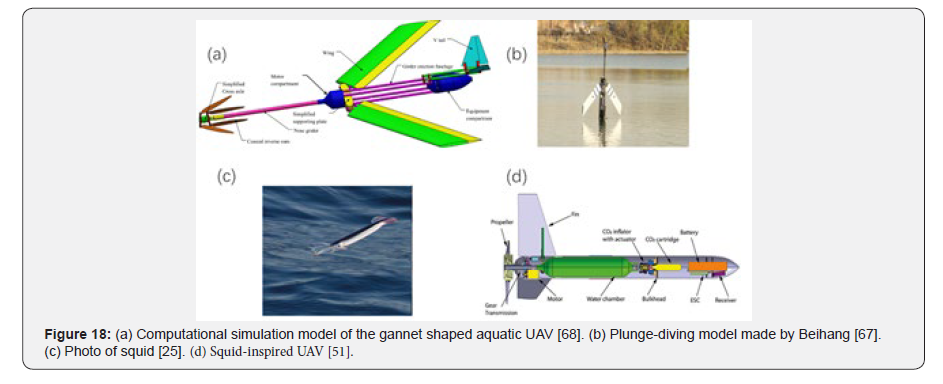
i. The first phase is the air phase. Gannets sweep their wings to adjust the posture for plunge-diving and they will experience a dropping process with a large oblique angle. When touched with water, they will streamline to avoid injury due to the collision.
ii. The second phase is the transition phase. Within hundreds of milliseconds, gannets split the water surface with a streamlined arrow-like posture at a specific oblique angle with the water surface, and travel from the air into the water rapidly [67].
iii. In the last phase, gannets will keep the posture until reaching the specific depth.
A plunge-diving UAV (Figure 18b) is made in [68,69]. A large impact force will be produced during the plunge-diving process and the value of impact force is obtained using a CFD method in [68]. The diving speed and beak angles at which the vehicle experience injury limited to boundary conditions, model and parameter assumptions are answered in [69]. Another plunge-diving bird called cormorant. An entry model mimicking cormorant is built in [70].
Rocket Transition (exit process):
By studying the unique thrusting mechanisms of squids, researchers get some new ideas in the water-air exiting design. The Neon Flying Squid propels itself out of the ocean by shooting a high pressurized waterjet (Figure 18c), before opening its fins to glide, covering them as long-distance as possible [25]. As they land back in the water, the fins are all folded back into place to minimize the impact [71]. Squid-inspired UAV uses a common CO2 cartridge to accelerate water stored inside a water chamber for efficient and sustained thrust (Figure 18d). In an underwater environment, water is the most accessible resource that could provide a high flow mass without introducing any additional weight. This system can transfer from water to air easily by shooting a high pressurized waterjet. While questions exist as well, during the operation time, CO2 tank is hard to fulfill. How to make sure the lasting time of the CO2 cartridge is a question too. Numerical analyses of the design and how much CO2 is needed to achieve the exit process are done in [72]. There are many good designs in [51,73] which can be referred to as examples.
Regular Transition (entry and exit):
Some other UAVs without CO2 cartridge nor plunge-diving structures have to use normal exit way. In [44] three main phases of water-air transitions are discussed.
i. The first phase happens when the fuselage breaks the surface of the water until the wing leading edge breaches the surface. During this phase, the vehicle is being accelerated upwards and building momentum, but due to reason that wing and fuselage compartments remain submerged, no significant draining occurs.
ii. The second phase starts when the leading edge of the wing breaks the surface and the passive draining of the wing happens, which ends until the trailing edge of the wing clears the surface. In this phase, the UAV will be more unstable in pitch, because the stabilizing effects of the wing against heave decrease. When the wings are out of the water and the vehicle can be more susceptible to wind disturbance.
iii. The last phase happens when the edge of the wing leaves the surface and ends when the vehicle is clear of the water.
The most significant limitation of regular transition UAVs are their inabilities to transfer between two modes of locomotion and the insufficient power to take off. This kind of UAVs can achieve their full functions if sufficient propulsive power is integrated for the take-off progress and well-designed transition algorithms are enabled [74]. There are also some types using positive buoyancy to execute successful transitions like the process shown in Figure 19 and the researchers find that a successful water exit relies a lot on both body angles at water exit together with initial speed.
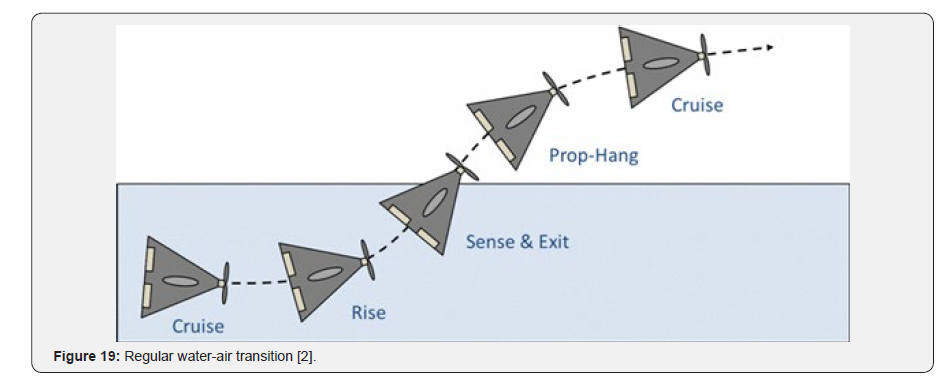
Other Problems with Design of an Aquatic UAV
The design of an aquatic UAV can be very complicated and there are still many other designing problems concerning with it, like the aquatic-aerial communication problems (discussed in 3.2.3), control problems of multi aquatic UAVs, take-off and landing problems, and control problems [75].
Control Problems of Multi Aquatic UAVs
A fully featured aquatic UAV can do many things, but compared to a group of aquatic UAVs, single UAV’s ability is largely limited. For instance, the wireless charging technology [33] mentioned in Section 3.2.2 cannot be done with one aquatic UAV. A group of aquatic UAVs can charge each other during the operation. A group of aquatic UAVs can also operate group tasks like transporting oil pipes undersea [18]. Cooperation algorithms should be considered comprehensively. In [76], a control strategy based on the multi-robot-fish’s competition is proposed. Using a learning-based coordination mechanism, two robot fish learn to cooperate with each other and achieve the goal. Robot learning can also be applied to every level of the architecture. To improve the implementation efficiency, the reactive behaviors will be defined by hand-coding on a priori knowledge and learning method will be used only in the deliberative behavior [76].
Take-off and Landing Problems
Although the take-off and diving processes from the underwater environment has been discussed detailly in Section 5.3, the take-off and landing processes are quite different on the water surface. When facing different wave conditions, especially in high sea states, an autonomous take-off control system for unmanned seaplanes is extremely important. The control system is based on fuzzy identification and generalized predictive control. Linear controlled auto-regressive integrated moving average model (CARIMA model) achieved from fuzzy identification is used as a representation of dynamic characteristics in various motion stages [77]. A vertical take-off strategy has been designed (Figure 20) in [75]. The simulations and experiments are well discussed in it as well.
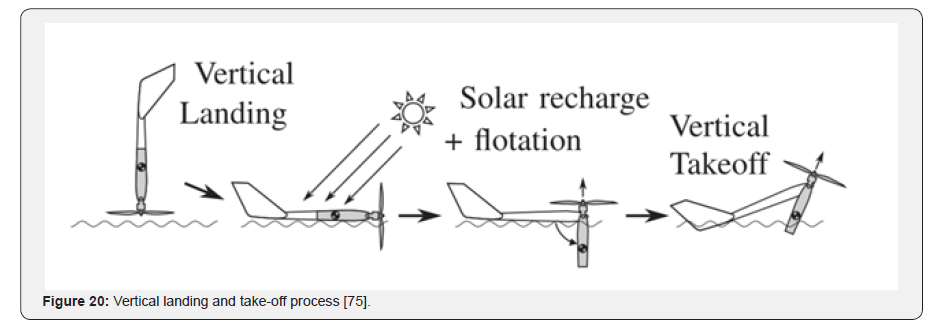
Aquatic-Aerial Communications
Because the water and the air are largely different in physical properties, the communication between these two media will be quite difficult to achieve. There are two main ways of wireless communication, i.e., acoustics communication system and radio frequency (RF). Of course, wire communication is also a way to communicate, but the range of working area and low operability make it not widely used. Acoustics communication is the most used way for underwater wireless links achieving a long link distance up to tens of kilometers, but it is limited to low frequencies (10HZ-1MHZ), so the bandwidth and transmission data rate are limited. RF is faster than acoustic ones, but the frequency is high, so the range is short. The tradeoff between range and speed is another important task. Some models are proposed in [78,79] to increase speed and range at the same time. A light-based underwater wireless communication system has also been developed [80]. But the light can be absorbed by the water if you dive too deep, so how to efficiently reduce the loss of light and how to combine light-based communications with acoustics communications should be taken into considerations seriously.
Control Problems
There is a basic problem in the design of UAVs, AUVs and aquatic UAVs from control perspective, e.g., how to maintain stability in the windy situation; how to switch from the underwater mode to the flying mode; how do quadcopter UAV move underwater. In [55-59,81], detailed algorithms for quadcopters’ control are proposed. In [82], a visual algorithm for UAVs is discussed detailly. The researchers propose a visionbased framework by addressing pose estimations for shortrange UAVs and detections using these systems. Accurate pose estimations and robust detections of docking stations are all equipped with aquatic UAVs. Experimental results show that the proposed framework is capable of detecting docking stations and estimating their relative pose more successfully and efficiently. For some biomimetic aquatic UAVs, algorithms mimicking movements of the animals are critical too. With these algorithms, aquatic UAVs can be more capable and efficient [24,82-84].
discussion
The Tradeoff between Merits and Drawbacks
During the interview with workers and administrative officials, we identified the following types of health hazards in the Leather industrial area.
Accidental hazards
As shown in the discussions above, to build an aquatic UAV, there are many obstacles to overcome and balances to be achieved. Quadcopters can achieve stable movements in the air and in the water. The transition processes are easy to design too. Furthermore, the technologies have been developed a lot in the aspect of drones, so aquatic UAVs can be easily made in the form of the quadcopters. But there are also many problems with quadcopter aquatic UAVs. The X-shaped drone is not streamlined shape, so the movement underwater and in the air are constrained compared to streamlined aquatic UAVs. Most of quadcopters aquatic UAVs have to use batteries to provide power, and this is a problem in the design of aquatic UAVs because the flying time is limited a lot. Plane-based and submarine-based aquatic UAVs are two main types of aquatic UAVs. How to combine them together well is a question too. In 7.2 we will show some insightful designs, many of them are conceptual designs, but can show us some inspirations in future design [85-87].
Some Insightful Designs
Swordfish like aquatic UAVs: Swordfish shaped aquatic UAV can move fast in the water. As shown in Figures 21a & b the sword in the front of the UAV can separate the seawater apart. A pair of hydrofoils in the front of the body can be used as front wings when flying. There is a pair of the adaptive till table ducted propellers in the middle of the body. These propellers can move and switch, and they are used to provide propulsions to fly in the air. They can also be used for vertical take-off and landing, like helicopters. The tail of the UAV adapts the X-shaped design, which has been used in submarine widely because of its good special stereo azimuth conversion capability. When operating under the water, the propellers can be folded or work together with the paddle in the back of the UAV to provide propulsions. The engine is hybrid powered. When the UAV flies in the air, the propellers can charge for the batteries. When in the water, the batteries power the paddles (propeller in the back).
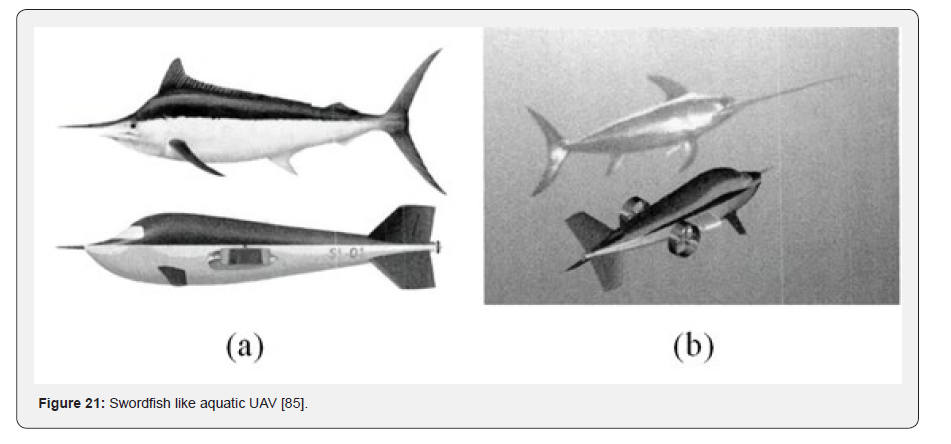
The streamlined shape can reduce resistance in the air and underwater and hydrofoils can be used as front wings in the air too. The change of positive and negative angles of attack can make motions of aquatic UAVs stable. The X-shaped design of tails equip the UAV with good special stereo azimuth conversion capability and good stability. The drawbacks are that folded propellers and the X-shaped tail can bring resistances because of the large exposing area in the air.
Skin rashes and dermatitis as a result of exposure to cleaners, solvents, disinfectants, pesticides, leather-processing chemicals, etc (Figure 2). Allergies-contact and systematic - caused by many of the chemicals used in tanneries [19].
Biological hazards
Submarine Based Aquatic UAVs: Submarine has excellent underwater navigation performance, but large submarine is hundreds of tons or even thousands of tons, so the idea of submarine take-off UAV is difficult to achieve, but for small-sized diving vehicles, the idea of submarine take-off is feasible. The helium gas compression tank can be placed into the submarine. When floating on the water, the helium gas can be released and placed in a special material balloon so that the submarine can take off. Propellers in the back of the fuselage propel the vehicle forward. An imaginary model with SolidWorks is created. (Figures 22a & b) is underwater situation and (Figure 22b) is aerial situation. Of course, in addition to helium, method of fuel combustion to float also works. However, this program is only applicable to small submarines. The position of the balloon and the amount of helium should be further calculated and studied.
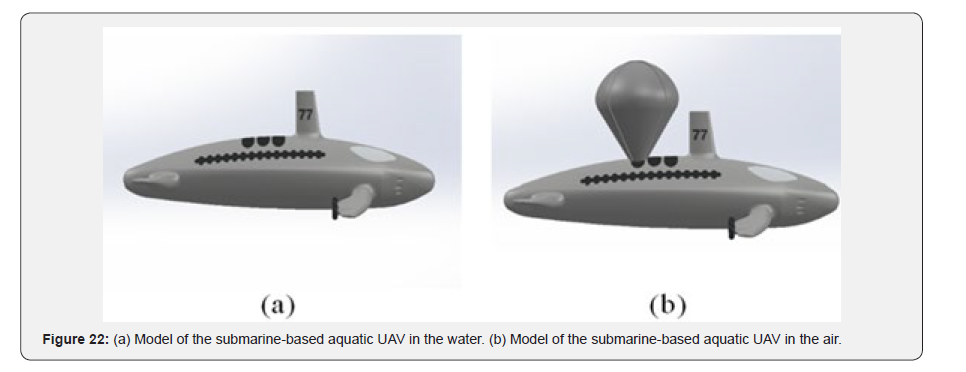
Summary and Conclusion
AUVs and UAVs are well developed in the last few decades. To facilitate developments of aquatic UAVs, many basic designs can follow the existing designs in UAVs and AUVs. So, in the beginning of the paper, we introduced some designs which can be used in aquatic UAVs from perspectives of AUVs and UAVs. Then we classify aquatic UAVs with two different methods. One uses the launching ways and the other uses wings types. The water-air transition, control system for multi-UAVs, take-off and landing processes, and aquatic-aerial communications problems are discussed as well. Biomimetics have been paid much attention to as well. They developed a lot in recent years which inspire designers to develop biomimetic aquatic UAVs using the revolutionary advantages. Many biomimetic prototypes have been tested to have cross-domains abilities though they cannot dive too deep nor dive too long underwater. But bio-inspired aquatic UAVs will contribute a lot to the ultimate realization of a fully functional aquatic UAV. Like batoid-shaped aquatic UAVs. In the water, the fins will move in a certain way to generate propulsions while in the air the fins can be used as wings to provide lift.
This is a promising design for aquatic UAVs and bio robotic aquatic UAVs. There are also many other good designs that can achieve the amphibious goal initially. Most of practicable designs are plane-based aquatic UAVs, which pay more attentions to flying rather than diving. However, there are few good designs in submarine-based aquatic UAVs. So further work can pay much attention to diving processes. For instance, a submarine equipped with a balloon can fly and dive, but the feasibility is unknown. Another problem with the existing designs is that designers do not pay much attention to flapping wings. In a big aquatic UAV, flapping wings are inefficient, but for small-sized aquatic UAVs, flapping wings are efficient and can adapt to the air and water conditions. The solar cells can provide power for small-sized aquatic UAVs. So small-sized insect-inspired aquatic UAVs with flapping wings should be paid more attention in the next few years. The developments of fully functional aquatic UAVs are promising and achievable. Many partially functional aquatic UAVs have proved the feasibility of a real one. We believe a fully featured aquatic UAV will be designed in the future.
This overview was finished when the first author was on the summer visit to Westlake University under the guidance of Prof. CUI Weicheng and his group. I am very grateful to Westlake University for providing me this precious opportunity. In particular, I appreciate very much to prof. Cui for his guidance on how to do research and various helps I received from his team members including Prof. LI Wei, Mr. Chen Hao, Prof. Zhang Liping, Prof. Song Changhui, Ms. SHA Jinyu, and Dr. LI Weikun.
References
- Pisanich G, Morris S (2002) Fielding an amphibious UAV: Development, results, and lessons learned; proceedings of the Proceedings The 21st Digital Avionics Systems Conference, IEEE.
- Moore J, Fein A, Setzler W (2018) Design and Analysis of a Fixed-Wing Unmanned Aerial-Aquatic Vehicle; proceedings of the 2018 IEEE International Conference on Robotics and Automation (ICRA), IEEE.
- Bar-Cohen Y (2006) Biomimetics--using nature to inspire human innovation. Bioinspir Biomim 1(1): P1-P12.
- Bhushan B (2009) Biomimetics: lessons from nature--an overview. Philos Trans A Math Phys Eng Sci 367(1893): 1445-1486.
- Vincent JF (2009) Biomimetics--a review. Proc Inst Mech Eng H 223(8): 919-939.
- Lepora NF, Verschure P, Prescott TJ (2013) The state of the art in biomimetics. Bioinspir Biomim 8(1): 013001.
- Ren L, Liang Y (2014) Preliminary studies on the basic factors of bionics. Science China Technological Sciences 57(3): 520-530.
- Costa F G, Ueyama J, Braun T, et al. (2012) The use of unmanned aerial vehicles and wireless sensor network in agricultural applications; proceedings of the 2012 IEEE International Geoscience and Remote Sensing Symposium p. 22-27.
- Göktoǧan AH, Sukkarieh S, Bryson M, Mitch Bryson, Jeremy Randle, et al. (2009) A Rotary-wing Unmanned Air Vehicle for Aquatic Weed Surveillance and Management. Journal of Intelligent and Robotic Systems 57(1-4): 467-484.
- Jacobi M (2015) Autonomous inspection of underwater structures. Robotics and Autonomous Systems 67: 80-86.
- Jun BH, Shim H, Kim B (2012) Preliminary design of the multi-legged underwater walking robot CR200; proceedings of the 2012 Oceans- Yeosu p. 21-24.
- Jun B, Shim H, Kim B, Jin-Yeong Park, Hyuk Baek, et al. (2013) Development of seabed walking robot CR200; proceedings of the 2013 MTS/IEEE Oceans- Bergen p. 10-14.
- Ferguson J (2009) Under-ice seabed mapping with AUVs; proceedings of the Oceans 2009-Europe, IEEE.
- Kaminski C, Crees T, Ferguson J (2010) 12 days under ice–an historic AUV deployment in the Canadian High Arctic; proceedings of the 2010 IEEE/OES Autonomous Underwater Vehicles, IEEE.
- Ore Jp, Elbaum S, Burgin A (2015) Autonomous Aerial Water Sampling. Journal of Field Robotics 32(8): 1095-1113.
- Rodrigues P, Marques F, Pinto E (2015) An open-source watertight unmanned aerial vehicle for water quality monitoring; proceedings of the Oceans 2015-MTS/IEEE Washington, IEEE.
- Schwarzbach M, Laiacker M, Mulero-Pázmány M (2014) Remote water sampling using flying robots; proceedings of the 2014 International Conference on Unmanned Aircraft Systems (ICUAS), IEEE.
- Simetti E, Casalino G (2016) Manipulation and transportation with cooperative underwater vehicle manipulator systems 42(4): 782-799.
- Alam K, Ray T, Anavatti SG (2014) A brief taxonomy of autonomous underwater vehicle design literature. Ocean Engineering 88: 627-630.
- CUI W (2019) An Overview of Submersible Research and Development in China. Journal of Marine Science and Application 17(4): 459-470.
- Ridao P, Carreras M, Ribas D (2015) Intervention AUVs: The next challenge. Annual Reviews in Control 40: 227-241.
- Marani G, Choi SK, Yuh J (2009) Underwater autonomous manipulation for intervention missions AUVs. Ocean Engineering 36(1): 15-23.
- Kadiyam J, Mohan S (2019) Conceptual design of a hybrid propulsion underwater robotic vehicle with different propulsion systems for ocean observations. Ocean Engineering 182: 112-125.
- Yao G, Liang J, Wang T (2013) Development of a turtle-like underwater vehicle using central pattern generator; proceedings of the 2013 IEEE International Conference on Robotics and Biomimetics (ROBIO), IEEE.
- Bacciaglia A, Guo D, Marzocca P (2018) Bimodal unmanned vehicle: propulsion system integration and water/air interface testing; proceedings of the 31st Congress of the international Council of the Aeronautical Sciences.
- Zhang T, Zhou H, Wang J (2019) Optimum design of a small intelligent ocean exploration underwater vehicle. Ocean Engineering 184: 40-58.
- Alvarez A, Bertram V, Gualdesi l (2009) Hull hydrodynamic optimization of autonomous underwater vehicles operating at snorkeling depth. Ocean Engineering 36(1): 105-112.
- Moored KW, Fish FE, Kemp TH (2011) Batoid fishes: inspiration for the next generation of underwater robots 45(4): 99-109.
- Gao A, Techet AH (2011) Design consideration for a robotic flying fish; proceedings of the Oceans 11 MTS/IEEE KONA, IEEE.
- Honaryar A, Ghiasi M (2018) Design of a Bio-inspired Hull Shape for an AUV from Hydrodynamic Stability Point of View through Experiment and Numerical Analysis. Journal of Bionic Engineering 15(6): 950-959.
- Villegas A, Mishkevich V, Gulak Y (2017) Analysis of key elements to evaluate the performance of a multirotor unmanned aerial–aquatic vehicle. Aerospace Science and Technology 70: 412-418.
- Kalikatzarakis M, Geertsma RD, Boonen EJ (2018) Ship energy management for hybrid propulsion and power supply with shore charging. Control Engineering Practice 76: 133-154.
- Chen B, Yang M, Chen Y (2019) A novel energy harvesting scheme in interference networks with UAVs. Physical Communication 33: 259-265.
- Wang Y, Yang X, Chen Y, Dylan Wainwright, Christopher PK, et al. (2017) A bio robotic adhesive disc for underwater hitchhiking inspired by the remora suckerfish. Science Robotics 2(10): 17-22.
- He,
- Eubank RD (2012) Autonomous Flight, Fault, and Energy Management of the Flying Fish Solar-Powered Seaplane [D]. PhD thesis, Aerospace Engineering in The University of Michigan.
- Eubank R, Atkins E, Macy D (2009) Autonomous guidance and control of the flying fish ocean surveillance platform; proceedings of the AIAA Infotech@ Aerospace Conference and AIAA Unmanned Unlimited Conference.
- Weisshaar TA (2013) Morphing Aircraft Systems: Historical Perspectives and Future Challenges. Journal of Aircraft 50(2): 337-353. Qingzhi (1997) Introduction to Aeronautics and Aerospace [M]. Beijing University of Aeronautics and Aerospace Press (in Chinese).
- Myring DF (2016) A Theoretical Study of Body Drag in Subcritical Axisymmetric Flow. Aeronautical Quarterly 27(3): 186-194.
- Ma D, Li Z, Yang M (2018) Sea-unammned aerial vehicle takeoff characteristics analysis method based on approximate equilibrium hypothesis. Proceedings of the Institution of Mechanical Engineers, Part G: Journal of Aerospace Engineering 233(3): 916-927.
- Yang X, Liang J, Li Y (2011) Modeling and analysis of variable buoyancy device imitating waterfowl plumage structure; proceedings of the Twenty-first International Offshore and Polar Engineering Conference. International Society of Offshore and Polar Engineers.
- (2007) Department of Defense, Unmanned Systems Roadmap FY2007-2032, department of Defense, Washington DC, USA.
- Erbil MA, Prior SD, Karamanoglu M (2009) Reconfigurable unmanned aerial vehicles; proceedings of the International Conference on Manufacturing and Engineering Systems Proceedings. International Conference on Manufacturing and Engineering Systems.
- Griffis C, Wilson T, Schneider J (2009) Unmanned Aircraft System Propulsion Systems Technology Survey.
- Yang X, Wang T, Liang J (2015) Survey on the novel hybrid aquatic–aerial amphibious aircraft: Aquatic unmanned aerial vehicle (Aqua UAV). Progress in Aerospace Sciences 74: 131-151.
- Siddall R, Kovac M (2014) Launching the Aqua MAV: bioinspired design for aerial-aquatic robotic platforms. Bioinspir Biomim 9(3): 031001.
- Weisler W, Stewart W, Anderson MB (2018) Testing and Characterization of a Fixed Wing Cross-Domain Unmanned Vehicle Operating in Aerial and Underwater Environments. IEEE Journal of Oceanic Engineering 43(4): 969-982.
- Gilad M, Shani L, Schneller A (2008) Waterspout-advanced deployable compact rotorcraft in support of special operation forces; proceedings of the 48th Israel Annual Conference on Aerospace Sciences.
- Chen Y, Helbling EF, Gravish N (2015) Hybrid aerial and aquatic locomotion in an at-scale robotic insect; proceedings of the 2015 IEEE/RSJ International Conference on Intelligent Robots and Systems (IROS), IEEE.
- Jafferis NT, Helbling EF, Karpelson M (2019) Untethered flight of an insect-sized flapping-wing microscale aerial vehicle. Nature 570(7762): 491-495.
- Guo D, Bacciaglia A, Simpson M (2019) Design and Development a Bimodal Unmanned System [M]. AIAA Scitech 2019 Forum.
- Liang J, Yao G, Wang T, Xing Bang Yang, Wen Di Zhao, et al. (2014) Wing load investigation of the plunge-diving locomotion of a gannet Morus inspired submersible aircraft. Science China Technological Sciences 57(2): 390-402.
- Desbiens A L, Pope M, Berg F (2013) Efficient Jump gliding: Theory and design considerations; proceedings of the 2013 IEEE International Conference on Robotics and Automation, IEEE.
- Alzu'bi H, Akinsanya O, Kaja N (2015) Evaluation of an aerial quadcopter power-plant for underwater operation; proceedings of the 2015 10th International Symposium on Mechatronics and its Applications (ISMA), IEEE.
- Alzu'bi H, Mansour I, Rawashdeh O (2018) Loon Copter: Implementation of a hybrid unmanned aquatic-aerial quadcopter with active buoyancy control. Journal of Field Robotics 35(5): 764-778.
- Mercado Ravell DA, Maia MM, Diez FJ (2018) Modeling and control of unmanned aerial/underwater vehicles using hybrid control. Control Engineering Practice 76: 112-122.
- Lu D, Xiong C, Lyu B (2018) Multi-Mode Hybrid Aerial Underwater Vehicle with Extended Endurance; proceedings of the 2018 OCEANS-MTS/IEEE Kobe Techno-Oceans (OTO), IEEE.
- Maia Mm, Soni P, Diez F (2015) Demonstration of an aerial and submersible vehicle capable of flight and underwater navigation with seamless air-water transition. Paper downloaded from Google without the publication information.
- Ma Z, Feng J, Yang J (2018) Research on vertical air–water trans-media control of Hybrid Unmanned Aerial Underwater Vehicles based on adaptive sliding mode dynamical surface control. International Journal of Advanced Robotic Systems 15(2): 1-10.
- Ristroph L, Childress S (2014) Stable hovering of a jellyfish-like flying machine. J R Soc Interface 11(92): 20130992.
- Nawroth JC, Lee H, Feinberg AW (2012) A tissue-engineered jellyfish with biomimetic propulsion. Nat Biotechnol 30(8): 792-797.
- Lock RJ, Vaidyanathan R, Burgess SC (2010) Development of a biologically inspired multi-modal wing model for aerial-aquatic robotic vehicles through empirical and numerical modelling of the common guillemot, Uria aalge. Bioinspir Biomim 5(4): 046001.
- Lock RJ, Peiris BPM, Bates S (2011) Quantification of the benefits of a compliant foil for underwater flapping wing propulsion; proceedings of the 2011 IEEE/ASME International Conference on Advanced Intelligent Mechatronics (AIM), IEEE.
- Izraelevitz JS, Triantafyllou MS (2015) A novel degree of freedom in flapping wings shows promise for a dual aerial/aquatic vehicle propulsor; proceedings of the 2015 IEEE International Conference on Robotics and Automation (ICRA), IEEE.
- Mackenzie D (2012) A flapping of wings [M]. American Association for the Advancement of Science.
- Ropert‐Coudert Y, Grémillet D, Ryan P (2004) Between air and water: the plunge dive of the Cape Gannet Morus capensis 146(2): 281-290.
- Wang TM, Yang XB, Liang JH (2013) CFD based investigation on the impact acceleration when a gannet impacts with water during plunge diving. Bioinspir Biomim 8(3): 036006.
- Yang X, Liang J, Wang T (2013) Computational simulation of a submersible unmanned aerial vehicle impacting with water; proceedings of the 2013 IEEE International Conference on Robotics and Biomimetics (ROBIO), IEEE.
- Zimmerman S, Ceballes S, Taylor G (2019) Nonlinear modeling and experimental verification of Gannet-inspired beam systems during diving. Bioinspir Biomim 14(2): 026002.
- Zink G (2008) Computational studies on the effect of water impact on an unmanned air vehicle. Retrospective Theses and Dissertations. 15296.
- O'Dor R, Stewart J, Gilly W, John Paynec, Teresa Cerveira Borges, et al. (2013) Squid rocket science: How squid launch into air. Deep Sea Research Part II: Topical Studies in Oceanography 95: 113-118.
- Siddall R, Kovac M (2016) Fast aquatic escape with a jet thruster 22(1): 217-226.
- Guo D, Bacciaglia A, Ceruti A (2018) Design and development of a transition propulsion system for bimodal unmanned vehicles; proceedings of the 2018 International Conference on Unmanned Aircraft Systems (ICUAS) IEEE.
- Tan Y H, Siddall R, Kovac M (2017) Efficient Aerial-Aquatic Locomotion with a Single Propulsion System. IEEE Robotics and Automation Letters 2(3): 1304-1311.
- Peloquin RA, Thibault D, Desbiens R (2016) Design of a passive vertical takeoff and landing aquatic UAV 2(2): 381-388.
- Yu J, Wang C, Xie G (2016) Coordination of Multiple Robotic Fish with Applications to Underwater Robot Competition. IEEE Transactions on Industrial Electronics 63(2): 1280-1288.
- Du H, Fan G, Yi J (2014) Autonomous takeoff control system design for unmanned seaplanes. Ocean Engineering 85: 21-31.
- Sharifzadeh M, Ahmadirad M (2018) Performance analysis of underwater wireless optical communication systems over a wide range of optical turbulence. Optics Communications 427: 609-616.
- Saeed N, Celik A, Al-Naffouri TY (2019) Underwater optical wireless communications, networking, and localization: A survey. Ad Hoc Networks 94: 101935.
- Oubei H M, Shen C, Kammoun A (2018) Light based underwater wireless communications. Japanese Journal of Applied Physics 57(8S2): 08PA06.
- Drews PL, Neto AA, Campos MF (2014) Hybrid unmanned aerial underwater vehicle: Modeling and simulation; proceedings of the 2014 IEEE/RSJ International Conference on Intelligent Robots and Systems, IEEE.
- Liu S, Ozay M, Okatani T (2018) Detection and Pose Estimation for Short-Range Vision-Based Underwater Docking 7: 2720-2749.
- Sarhadi P, Noei AR, Khosravi A (2016) Model reference adaptive PID control with anti-windup compensator for an autonomous underwater vehicle. Robotics and Autonomous Systems 83: 87-93.
- Xiang X, Lapierre L, Jouvencel B (2015) Smooth transition of AUV motion control: From fully actuated to under-actuated configuration. Robotics and Autonomous Systems 67: 14-22.
- https://web.whoi.edu/hades/nereus/
- https://en.wikipedia.org/wiki/Unmanned_aerial_vehicle.
- https://en.wikipedia.org/wiki/Aircraft#Heavier-than-air_–_aerodynes.
- Pisanich G, Morris S (2002) Fielding an amphibious UAV: Development, results, and lessons learned; proceedings of the Proceedings The 21st Digital Avionics Systems Conference, IEEE.
- Moore J, Fein A, Setzler W (2018) Design and Analysis of a Fixed-Wing Unmanned Aerial-Aquatic Vehicle; proceedings of the 2018 IEEE International Conference on Robotics and Automation (ICRA), IEEE.
- Bar-Cohen Y (2006) Biomimetics--using nature to inspire human innovation. Bioinspir Biomim 1(1): P1-P12.
- Bhushan B (2009) Biomimetics: lessons from nature--an overview. Philos Trans A Math Phys Eng Sci 367(1893): 1445-1486.
- Vincent JF (2009) Biomimetics--a review. Proc Inst Mech Eng H 223(8): 919-939.
- Lepora NF, Verschure P, Prescott TJ (2013) The state of the art in biomimetics. Bioinspir Biomim 8(1): 013001.
- Ren L, Liang Y (2014) Preliminary studies on the basic factors of bionics. Science China Technological Sciences 57(3): 520-530.
- Costa F G, Ueyama J, Braun T, et al. (2012) The use of unmanned aerial vehicles and wireless sensor network in agricultural applications; proceedings of the 2012 IEEE International Geoscience and Remote Sensing Symposium p. 22-27.
- Göktoǧan AH, Sukkarieh S, Bryson M, Mitch Bryson, Jeremy Randle, et al. (2009) A Rotary-wing Unmanned Air Vehicle for Aquatic Weed Surveillance and Management. Journal of Intelligent and Robotic Systems 57(1-4): 467-484.
- Jacobi M (2015) Autonomous inspection of underwater structures. Robotics and Autonomous Systems 67: 80-86.
- Jun BH, Shim H, Kim B (2012) Preliminary design of the multi-legged underwater walking robot CR200; proceedings of the 2012 Oceans- Yeosu p. 21-24.
- Jun B, Shim H, Kim B, Jin-Yeong Park, Hyuk Baek, et al. (2013) Development of seabed walking robot CR200; proceedings of the 2013 MTS/IEEE Oceans- Bergen p. 10-14.
- Ferguson J (2009) Under-ice seabed mapping with AUVs; proceedings of the Oceans 2009-Europe, IEEE.
- Kaminski C, Crees T, Ferguson J (2010) 12 days under ice–an historic AUV deployment in the Canadian High Arctic; proceedings of the 2010 IEEE/OES Autonomous Underwater Vehicles, IEEE.
- Ore Jp, Elbaum S, Burgin A (2015) Autonomous Aerial Water Sampling. Journal of Field Robotics 32(8): 1095-1113.
- Rodrigues P, Marques F, Pinto E (2015) An open-source watertight unmanned aerial vehicle for water quality monitoring; proceedings of the Oceans 2015-MTS/IEEE Washington, IEEE.
- Schwarzbach M, Laiacker M, Mulero-Pázmány M (2014) Remote water sampling using flying robots; proceedings of the 2014 International Conference on Unmanned Aircraft Systems (ICUAS), IEEE.
- Simetti E, Casalino G (2016) Manipulation and transportation with cooperative underwater vehicle manipulator systems 42(4): 782-799.
- Alam K, Ray T, Anavatti SG (2014) A brief taxonomy of autonomous underwater vehicle design literature. Ocean Engineering 88: 627-630.
- CUI W (2019) An Overview of Submersible Research and Development in China. Journal of Marine Science and Application 17(4): 459-470.
- Ridao P, Carreras M, Ribas D (2015) Intervention AUVs: The next challenge. Annual Reviews in Control 40: 227-241.
- Marani G, Choi SK, Yuh J (2009) Underwater autonomous manipulation for intervention missions AUVs. Ocean Engineering 36(1): 15-23.
- Kadiyam J, Mohan S (2019) Conceptual design of a hybrid propulsion underwater robotic vehicle with different propulsion systems for ocean observations. Ocean Engineering 182: 112-125.
- Yao G, Liang J, Wang T (2013) Development of a turtle-like underwater vehicle using central pattern generator; proceedings of the 2013 IEEE International Conference on Robotics and Biomimetics (ROBIO), IEEE.
- Bacciaglia A, Guo D, Marzocca P (2018) Bimodal unmanned vehicle: propulsion system integration and water/air interface testing; proceedings of the 31st Congress of the international Council of the Aeronautical Sciences.
- Zhang T, Zhou H, Wang J (2019) Optimum design of a small intelligent ocean exploration underwater vehicle. Ocean Engineering 184: 40-58.
- Alvarez A, Bertram V, Gualdesi l (2009) Hull hydrodynamic optimization of autonomous underwater vehicles operating at snorkeling depth. Ocean Engineering 36(1): 105-112.
- Moored KW, Fish FE, Kemp TH (2011) Batoid fishes: inspiration for the next generation of underwater robots 45(4): 99-109.
- Gao A, Techet AH (2011) Design consideration for a robotic flying fish; proceedings of the Oceans 11 MTS/IEEE KONA, IEEE.
- Honaryar A, Ghiasi M (2018) Design of a Bio-inspired Hull Shape for an AUV from Hydrodynamic Stability Point of View through Experiment and Numerical Analysis. Journal of Bionic Engineering 15(6): 950-959.
- Villegas A, Mishkevich V, Gulak Y (2017) Analysis of key elements to evaluate the performance of a multirotor unmanned aerial–aquatic vehicle. Aerospace Science and Technology 70: 412-418.
- Kalikatzarakis M, Geertsma RD, Boonen EJ (2018) Ship energy management for hybrid propulsion and power supply with shore charging. Control Engineering Practice 76: 133-154.
- Chen B, Yang M, Chen Y (2019) A novel energy harvesting scheme in interference networks with UAVs. Physical Communication 33: 259-265.
- Wang Y, Yang X, Chen Y, Dylan Wainwright, Christopher PK, et al. (2017) A bio robotic adhesive disc for underwater hitchhiking inspired by the remora suckerfish. Science Robotics 2(10): 17-22.
- He,
- Eubank RD (2012) Autonomous Flight, Fault, and Energy Management of the Flying Fish Solar-Powered Seaplane [D]. PhD thesis, Aerospace Engineering in The University of Michigan.
- Eubank R, Atkins E, Macy D (2009) Autonomous guidance and control of the flying fish ocean surveillance platform; proceedings of the AIAA Infotech@ Aerospace Conference and AIAA Unmanned Unlimited Conference.
- Weisshaar TA (2013) Morphing Aircraft Systems: Historical Perspectives and Future Challenges. Journal of Aircraft 50(2): 337-353. Qingzhi (1997) Introduction to Aeronautics and Aerospace [M]. Beijing University of Aeronautics and Aerospace Press (in Chinese).
- Myring DF (2016) A Theoretical Study of Body Drag in Subcritical Axisymmetric Flow. Aeronautical Quarterly 27(3): 186-194.
- Ma D, Li Z, Yang M (2018) Sea-unammned aerial vehicle takeoff characteristics analysis method based on approximate equilibrium hypothesis. Proceedings of the Institution of Mechanical Engineers, Part G: Journal of Aerospace Engineering 233(3): 916-927.
- Yang X, Liang J, Li Y (2011) Modeling and analysis of variable buoyancy device imitating waterfowl plumage structure; proceedings of the Twenty-first International Offshore and Polar Engineering Conference. International Society of Offshore and Polar Engineers.
- (2007) Department of Defense, Unmanned Systems Roadmap FY2007-2032, department of Defense, Washington DC, USA.
- Erbil MA, Prior SD, Karamanoglu M (2009) Reconfigurable unmanned aerial vehicles; proceedings of the International Conference on Manufacturing and Engineering Systems Proceedings. International Conference on Manufacturing and Engineering Systems.
- Griffis C, Wilson T, Schneider J (2009) Unmanned Aircraft System Propulsion Systems Technology Survey.
- Yang X, Wang T, Liang J (2015) Survey on the novel hybrid aquatic–aerial amphibious aircraft: Aquatic unmanned aerial vehicle (Aqua UAV). Progress in Aerospace Sciences 74: 131-151.
- Siddall R, Kovac M (2014) Launching the Aqua MAV: bioinspired design for aerial-aquatic robotic platforms. Bioinspir Biomim 9(3): 031001.
- Weisler W, Stewart W, Anderson MB (2018) Testing and Characterization of a Fixed Wing Cross-Domain Unmanned Vehicle Operating in Aerial and Underwater Environments. IEEE Journal of Oceanic Engineering 43(4): 969-982.
- Gilad M, Shani L, Schneller A (2008) Waterspout-advanced deployable compact rotorcraft in support of special operation forces; proceedings of the 48th Israel Annual Conference on Aerospace Sciences.
- Chen Y, Helbling EF, Gravish N (2015) Hybrid aerial and aquatic locomotion in an at-scale robotic insect; proceedings of the 2015 IEEE/RSJ International Conference on Intelligent Robots and Systems (IROS), IEEE.
- Jafferis NT, Helbling EF, Karpelson M (2019) Untethered flight of an insect-sized flapping-wing microscale aerial vehicle. Nature 570(7762): 491-495.
- Guo D, Bacciaglia A, Simpson M (2019) Design and Development a Bimodal Unmanned System [M]. AIAA Scitech 2019 Forum.
- Liang J, Yao G, Wang T, Xing Bang Yang, Wen Di Zhao, et al. (2014) Wing load investigation of the plunge-diving locomotion of a gannet Morus inspired submersible aircraft. Science China Technological Sciences 57(2): 390-402.
- Desbiens A L, Pope M, Berg F (2013) Efficient Jump gliding: Theory and design considerations; proceedings of the 2013 IEEE International Conference on Robotics and Automation, IEEE.
- Alzu'bi H, Akinsanya O, Kaja N (2015) Evaluation of an aerial quadcopter power-plant for underwater operation; proceedings of the 2015 10th International Symposium on Mechatronics and its Applications (ISMA), IEEE.
- Alzu'bi H, Mansour I, Rawashdeh O (2018) Loon Copter: Implementation of a hybrid unmanned aquatic-aerial quadcopter with active buoyancy control. Journal of Field Robotics 35(5): 764-778.
- Mercado Ravell DA, Maia MM, Diez FJ (2018) Modeling and control of unmanned aerial/underwater vehicles using hybrid control. Control Engineering Practice 76: 112-122.
- Lu D, Xiong C, Lyu B (2018) Multi-Mode Hybrid Aerial Underwater Vehicle with Extended Endurance; proceedings of the 2018 OCEANS-MTS/IEEE Kobe Techno-Oceans (OTO), IEEE.
- Maia Mm, Soni P, Diez F (2015) Demonstration of an aerial and submersible vehicle capable of flight and underwater navigation with seamless air-water transition. Paper downloaded from Google without the publication information.
- Ma Z, Feng J, Yang J (2018) Research on vertical air–water trans-media control of Hybrid Unmanned Aerial Underwater Vehicles based on adaptive sliding mode dynamical surface control. International Journal of Advanced Robotic Systems 15(2): 1-10.
- Ristroph L, Childress S (2014) Stable hovering of a jellyfish-like flying machine. J R Soc Interface 11(92): 20130992.
- Nawroth JC, Lee H, Feinberg AW (2012) A tissue-engineered jellyfish with biomimetic propulsion. Nat Biotechnol 30(8): 792-797.
- Lock RJ, Vaidyanathan R, Burgess SC (2010) Development of a biologically inspired multi-modal wing model for aerial-aquatic robotic vehicles through empirical and numerical modelling of the common guillemot, Uria aalge. Bioinspir Biomim 5(4): 046001.
- Lock RJ, Peiris BPM, Bates S (2011) Quantification of the benefits of a compliant foil for underwater flapping wing propulsion; proceedings of the 2011 IEEE/ASME International Conference on Advanced Intelligent Mechatronics (AIM), IEEE.
- Izraelevitz JS, Triantafyllou MS (2015) A novel degree of freedom in flapping wings shows promise for a dual aerial/aquatic vehicle propulsor; proceedings of the 2015 IEEE International Conference on Robotics and Automation (ICRA), IEEE.
- Mackenzie D (2012) A flapping of wings [M]. American Association for the Advancement of Science.
- Ropert‐Coudert Y, Grémillet D, Ryan P (2004) Between air and water: the plunge dive of the Cape Gannet Morus capensis 146(2): 281-290.
- Wang TM, Yang XB, Liang JH (2013) CFD based investigation on the impact acceleration when a gannet impacts with water during plunge diving. Bioinspir Biomim 8(3): 036006.
- Yang X, Liang J, Wang T (2013) Computational simulation of a submersible unmanned aerial vehicle impacting with water; proceedings of the 2013 IEEE International Conference on Robotics and Biomimetics (ROBIO), IEEE.
- Zimmerman S, Ceballes S, Taylor G (2019) Nonlinear modeling and experimental verification of Gannet-inspired beam systems during diving. Bioinspir Biomim 14(2): 026002.
- Zink G (2008) Computational studies on the effect of water impact on an unmanned air vehicle. Retrospective Theses and Dissertations. 15296.
- O'Dor R, Stewart J, Gilly W, John Paynec, Teresa Cerveira Borges, et al. (2013) Squid rocket science: How squid launch into air. Deep Sea Research Part II: Topical Studies in Oceanography 95: 113-118.
- Siddall R, Kovac M (2016) Fast aquatic escape with a jet thruster 22(1): 217-226.
- Guo D, Bacciaglia A, Ceruti A (2018) Design and development of a transition propulsion system for bimodal unmanned vehicles; proceedings of the 2018 International Conference on Unmanned Aircraft Systems (ICUAS) IEEE.
- Tan Y H, Siddall R, Kovac M (2017) Efficient Aerial-Aquatic Locomotion with a Single Propulsion System. IEEE Robotics and Automation Letters 2(3): 1304-1311.
- Peloquin RA, Thibault D, Desbiens R (2016) Design of a passive vertical takeoff and landing aquatic UAV 2(2): 381-388.
- Yu J, Wang C, Xie G (2016) Coordination of Multiple Robotic Fish with Applications to Underwater Robot Competition. IEEE Transactions on Industrial Electronics 63(2): 1280-1288.
- Du H, Fan G, Yi J (2014) Autonomous takeoff control system design for unmanned seaplanes. Ocean Engineering 85: 21-31.
- Sharifzadeh M, Ahmadirad M (2018) Performance analysis of underwater wireless optical communication systems over a wide range of optical turbulence. Optics Communications 427: 609-616.
- Saeed N, Celik A, Al-Naffouri TY (2019) Underwater optical wireless communications, networking, and localization: A survey. Ad Hoc Networks 94: 101935.
- Oubei H M, Shen C, Kammoun A (2018) Light based underwater wireless communications. Japanese Journal of Applied Physics 57(8S2): 08PA06.
- Drews PL, Neto AA, Campos MF (2014) Hybrid unmanned aerial underwater vehicle: Modeling and simulation; proceedings of the 2014 IEEE/RSJ International Conference on Intelligent Robots and Systems, IEEE.
- Liu S, Ozay M, Okatani T (2018) Detection and Pose Estimation for Short-Range Vision-Based Underwater Docking 7: 2720-2749.
- Sarhadi P, Noei AR, Khosravi A (2016) Model reference adaptive PID control with anti-windup compensator for an autonomous underwater vehicle. Robotics and Autonomous Systems 83: 87-93.
- Xiang X, Lapierre L, Jouvencel B (2015) Smooth transition of AUV motion control: From fully actuated to under-actuated configuration. Robotics and Autonomous Systems 67: 14-22.
- https://web.whoi.edu/hades/nereus/
- https://en.wikipedia.org/wiki/Unmanned_aerial_vehicle.
- https://en.wikipedia.org/wiki/Aircraft#Heavier-than-air_–_aerodynes.