The Chemistry of Oxidative Stress and Glycoxidation As Risk Factors for Developing Degenerative Disease
Marco Brito Arias*
Instituto Politécnico Nacional, México
Submission: September 03, 2018;Published: June 25, 2019p>
*Corresponding author: Marco Brito Arias, Unidad Profesional Interdisciplinaria de Biotecnología- Instituto Politécnico (UPIBI-IPN), Avenida Acueducto s/n Colonia La Laguna Ticomán CP 07340 Ciudad de México
How to cite this article: M Brito-Arias. The Chemistry of Oxidative Stress and Glycoxidation As Risk Factors for Developing Degenerative Disease. Ann Rev Resear. 2019; 4(5): 555648.
Abstract
Oxidative stress conceived as an abnormal production of highly reactive oxygen species has been strongly implicated in degenerative and chronic diseases such as diabetes, cancer, coronary artery disease, vitiligo, arteriosclerosis, lupus, rheumatoid arthritis, and neurodegenerative pathologies. Additionally, glycoxidation is intimately associated with diabetes, coronary heart disease, retinopathy, Parkinson, Lewy body disease and aging. These oxidative processes are strongly interconnected and related to common alterations and therefore it is primordial to understand their chemistry and what is the impact of the oxidative stress and glycoxidation on the modulation and homeostasis of the body, with the aim of establishing strategies that could lead to the prevention or control of chronic and degenerative disease.
Keywords: Reactive oxygen species, Glycosylation, Diabetes, Amadori adducts, Advanced glycosylation, Autoimmune, Degenerative disease, Mitochondria, Cell membrane, Abnormal redox processes, Enzymes, Xanthine oxidase, Xanthine, Physiological purine substrates, L-arginine, Noncovalently bound, Hydroxylation, Catalytic site, Iron atom, Superoxide anion
Introduction
Oxidative stress has been defined as an imbalance between oxidants and antioxidants in favor of the oxidants, potentially leading to damage [1] The reactive species known as reactive ox ygen species (ROS), are the superoxide anion (O2.-), the hydroxyl radical (•OH), hydrogen peroxide (H2O2), hydroperoxyl radical (HOO•) and singlet oxygen (1O2) (Figure 1).
Almost more than 60 percent of tannery industries have been relocated in the newly formed leather industry city in the Hemayetpur area of a saver. The industries are producing health hazard among the workers and inhabitants and the industries are also producing an environmental hazard [12,13]. There are many studies on different issues of leather industries in the Hazaribagh area. Until there are no such types of studies on Hemayetpur tannery industrial area. Thus, here we tried to assess the risk of health hazard among workers in tannery industries and environmental hazards produced in the area. The study attempted to assess the health and environmental risk generated from the production activities of the tannery industry.

On the other hand, reactive nitrogen species (RNS) also participate in the form of nitric oxide (NO•) and peroxynitrite anion (ONOO−) [2]. ROS are mainly expressed in mitochondria, cell membrane and nucleus and combines extracellular components such as superoxide dismutase and glutathione, with abnormal redox processes. Different studies pointed out that the increase of ROS is associated with anomalous production of hydrogen peroxide (H2O2) and peroxynitrite (ONOO−) in the mitochondria [3,4]. The generation of superoxide anion (•O2-) as a central player is attributed to the combination of electrons coming from the NOX2/ p22phox complex and the subunits p47, p67, and p40 with free oxygen. This highly reactive specie is quickly transformed to hydrogen peroxide (H2O2) by the action of superoxide dismutase or to hydroxyl radical in combination with Fe2+. The superoxide anion can also react with nitric oxide (NO) to form the potent oxidant peroxynitrite (ONOO-) associated with oxidative damage of biomolecules [5] (Figure 2).
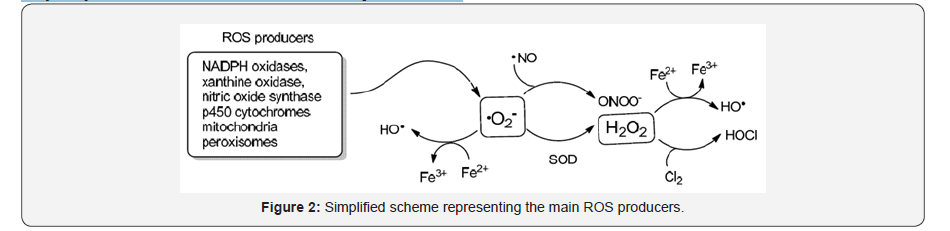
On the other hand, the degradation of oxidative species is mediated by reduced glutathione (2GSH) which in turn is trans formed to the oxidized glutathione (GSSG) and reactivated to the reduced form by the system NADPH/H+ → NAP+.

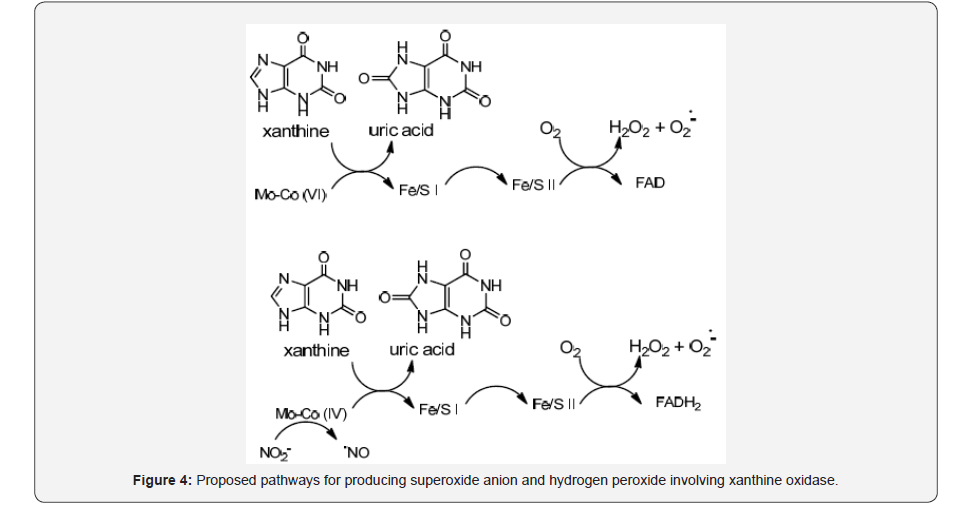
Enzymes involved in the production of ROS Some of the enzymes identified as ROS producers are NADPH oxidases, xanthine oxidase, nitric oxide synthetase, p450 cytochromes and organelles (mitochondria, peroxisomes). A well identified source of ROS in humans is the NADPH oxidase consisting in a multi subunit enzyme complex of flavocytochrome b558 formed by two membrane subunits p22phox and gpp1phox, activated by cytosolic p40phox, p47phox, p67phox and the GTPase Rac [6]. The normal production of ROS is necessary for autoimmune response, cellular signaling, and the regulation of gene expression, however the overproduction give place to serious alterations including cancer and autoimmune diseases [7]. The maximal activation of NADPH oxidase requires a complex formation between p40phox and p67phox subunits via association of their PB1 domains [8] (Figure 3).
Xanthine oxidase is a molybdenum hydroxylase family of proteins having one FAD site and two Fe/S clusters which catalyses the hydroxylation of hypoxanthine to xanthine as well as xanthine to uric acid [9]. During inflammatory conditions xanthine dehydrogenase is converted to xanthine oxidase, giving as result a NAD+ decrease while oxygen affinity increase, with the resulting generation of superoxide anion and hydrogen peroxide. The proposed mechanism for the generation of oxidative species such as superoxide anion (O2.-) and hydrogen peroxide (H2O2) involves xanthine oxidation to uric acid where electrons are transferred to FAD resulting in O2reduction to H2O2 and (O2-).
Alternatively, superoxide anion and hydrogen peroxide can be generated by NO2- reduction to •NO at the Mo cofactor with electrons donated by xanthine [10] (Figure 4).
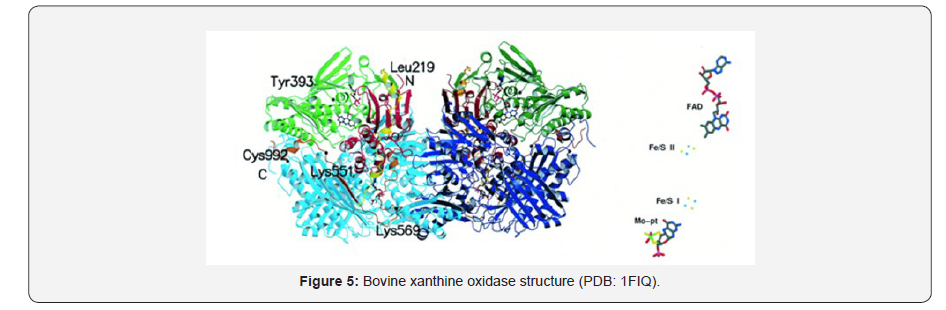
The crystal structure of bovine xanthine oxidase shows a molybdenum- containing enzyme conformed by two monomers with domains iron/sulphur-center domains (residues 3-165), FAD domain (residues 226-531), and Mo-pt domain (residues 590-1,331) [11]. This butterfly shaped dimer catalyses the hydroxylation of a wide variety of aromatic heterocycles, including the physiological purine substrates, hypoxanthine and xanthine, and aldehydes [12] (Figure 5).


Nitric oxide synthase (NOS) isoforms uses L-arginine as substrate and in combination with O2 and NADPH produce superoxide anion (O2.-). The whole process involves an electron transfer from reduced nicotinamide-adenine-dinucleotide phosphate (NADPH), to flavin-adenine-dinucleotide (FAD) and flavin-mononucleotide (FMN), which ultimately will reduce molecular oxygen to superoxide (O2.-), although with limited capacity [13] (Figure 6).
Glucose oxidase is a homodimer consisting in two identical subunits and two noncovalently bound flavin adenine dinucleotides (FAD), and its main function is to produce H2O2 as antibacterial and anti-fungal repulsive agent [14]. Glucose oxidase is responsible of the conversion of β-D-glucose to D-gluconolactone and hydrogen peroxide. A detailed reductive half-reaction shows the oxidation reaction of β-D-glucose involving a hydride migration of C1 to His516 and FAD (Figure 7).
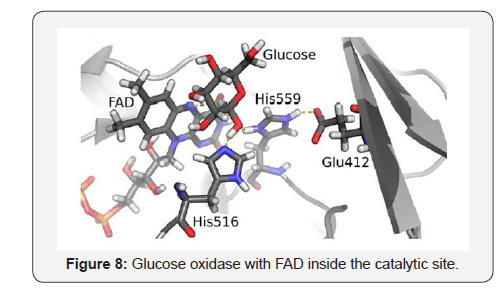
The catalytic site involving FAD, His516, His559, Glu412 and glucose as substrate, indicates that the protonation of His516 and hydride migration to the N5 of the flavine adenine dinucleotide result critical in the transition state [15] (Figure 8).

Cytochrome p450 is an enzyme complex that plays a central role in detoxification processes mainly of drugs during the phase I and carries out several oxidative transformations on alkenes, heteroatoms, carbonyl groups, aromatic and other alpha activated positions. The oxidation products include mainly hydroxylation, however epoxidation, N-oxides, S-oxides, oximes, O- and N-dealkylations are usually observed. The p450 enzyme family utilize in the catalytic site the heme group, the iron atom, and the NADPH as a redox system as it can be visualized in the hydroxylation reaction of benzene (Figure 9)
The catalytic cycle of cytochrome p450 postulates different intermediates some of them isolated and studied extensively by different spectroscopies [16,17] (Figure 10).

The vast majority of P450s requires NAD(P)H as a redox partner in vivo, although an alternative route involves hydrogen peroxide (H2O2) to generate iron-oxo species [18]. The crystal structure of P450 3A4 (CYP3A4) which metabolize more drugs than any other isoform was determined, showing the heme grupo as a balland- stick model in the center of the molecule, flanked by helix [19] (Figure 11).
Mitochondria organelle is another important source for the generation of reactive oxidative species (ROS) besides his primor dial activity regarding the ATP production within the cell. The increase in the production of ROS superoxide anion (O2.-) is observed under two circumstances, a diminished ATP levels and when there is a high NADH/NAD+ ratio in the matrix [20]. The generation of superoxide anion also depends on the proton motive force Dp determined by the redox systems NADH/NAD+, CoQH2/CoQ ratios and the O2 concentrations. However, according to literature small molecule electron carriers such as NADH, NADPH, CoQH2 (reduced coenzyme Q) and glutathione (GSH) do not react with O2 to generate O2.-, so this reduced form is mainly generated at low ATP production levels and high NADH/NAD+, or CoQH2/CoQ ratios during the reverse electron transport (RET) consisting in the transfer of two electrons to the CoQ pool where in the presences of Dp force the electrons moves back from CoQH2 into the complex I of the respiratory chain and reduce NAD+ to NADH at the FMN site[21-24] (Figure 12).


Peroxisomes are membrane-bound organelles involved primarily in the lipid metabolism and not less important in the productions of reactive oxygen species (ROS). With the discovery of an alternative respiratory pathway in peroxisomes, which is characterized by the generation of electrons from different metabolites, which convert the O2 into H2O2 and finally to H2O, several studies have been directed with the aim of establishing a potential connexion between the oxidative processes with human malignancies. It is well known that the main metabolic processes associated with the H2O2 production in peroxisomes are the β-oxidation of fatty acids. The enzymes presents in peroxisomes that generate ROS are: Acyl-CoA oxidase (Palmitoyl-CoA oxidase, Pristanoyl-CoA oxidase, Trihydroxycoprostanoyl-CoA oxidase), Urate oxidase, Xanthine oxidase, D-amino acid oxidase, pipecolic acid oxidase, D-aspartate oxidase, sarosine oxidase, L-alpha hydroxyl acid oxidase, poly amine oxidase, nitric oxide synthase and plant sulphite oxidase [25].

Glycoxidation
Carbohydrates are biological units with unquestionable importance for the well-functioning of the cell, however the intake of high amounts of some sugars specially sucrose, fructose and starch which ultimately will deliver glucose, had modified dramatically the conception about the good reputation of the carbohydrates, and how to deal with this primordial substance considering that glucose is the first alternative as a fuel by the cell. Numerous studies have been undertaken to understand the implications of the carbohydrates in alterations or dysfunctions associated with the oxidative stress and glycoxidation which has been identified with accuracy through intensive analysis. Monosaccharaides such as glucose, fructose, and disaccharides (sucrose and maltose) may react under non enzymatic and enzymatic conditions with ROS. Example of the former case is the reaction of monosaccharaides and disaccharides with hydroxyl radicals (HO•) to produce initially a secondary hydroxyl alcohol radical which is further oxidized with O2 to hydroxyalkylperoxy radical. Final decomposition will furnish the corresponding ketone and superoxide anion O2.- [26] (Figure 13).

On the other hand, the well-known Fenton reaction is an example of enzymatic reaction, consisting in the oxidation mediated by glucose oxidase of the anomeric hydroxyl group of β-D-glucose to form D-gluconolactone and hydrogen peroxide which in the presence of Fe+2 decompose to hydroxyl anion and hydroxyl radical [27] (Figure 14).
Another important transformation involving saccharides is the Amadori reaction for glucose (Figure 15) or Heyns for fructose [28] (Figure 16) also known in food chemistry as Millard reaction when the amines belong to amino acid residues present in proteins. This process has been largely considered a non-enzymatic reaction although recent evidence suggests the participation of enzymes especially for the attachment of some amino acids [29]. This important transformation with high implications in food chemistry as well as in degenerative processes mainly diabetes mellitus and autoimmune disease is associated with the formation of α-ceto aldehydes considered oxidation products implicated in the formation of advanced glycation end products (AGES). Such Amadori adducts have been identified in hemoglobin through the attachment with lysine residues [30].


Glucuronic acid is another monosaccharide with importance in phase II of metabolism implicated in the detoxification of drugs that has been described to generate Amadori adducts under non-enzymatic conditions, and it has been conjugated to lysine containing pentapeptides under mild conditions [31] (Figure 17).


As predicted, amino purines also react with glucose either in the open or cyclic forms leading through the imine of Schiff product which establish an equilibrium with the enaminol product, and then addition to the enamine to form the cyclic Amadori-purine, adducts [31-33] (Figure 18).
Also, purines can be modified by oxygen radicals that react with polyunsaturated fatty acid residues in phospholipids producing a cocktail of products being Malondialdehyde (MDA) a major product of lipid peroxidation [34] (Figure 19).
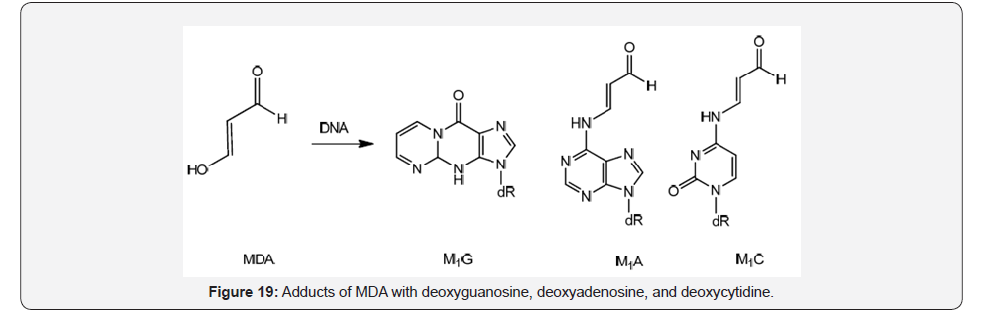
High glucose concentration brings as a pathologic consequence the abnormal attachment with proteins to generate Amadori adducts, advanced glycosylation end products (AGES) and reactive carbonyl species such as glyoxal (GO) and methyl glyoxal(MGO) 3-deoxyglucosone (3-DG). The reactive carbonyl species may react with amino acid lysine and arginine to produce imidazole derivatives and other AGES adducts [35] (Figure 20).

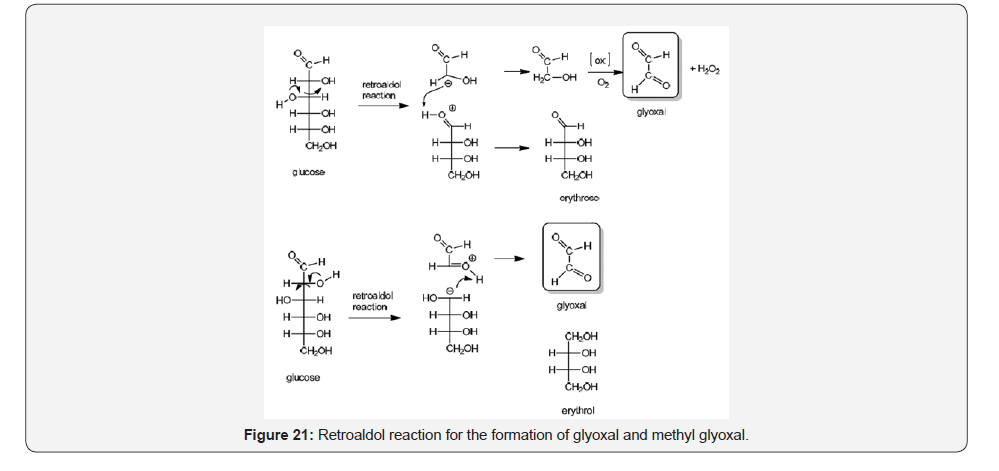
Non enzymatic glyoxal (GO) and methyl glyoxal (MGO) formation from glucose is a process with high implications since these α-oxoaldehydes are responsible of most of the advanced glycosylation product (AGES) especially after attachment with amino acids arginine and lysine. A proposed mechanism for converting glucose into glyoxal considers a retroaldol reaction followed by autooxidation, or direct glyoxal formation and erythrol [36,37] (Figure 21).


Alternatively, methyl glyoxal can be generated from glucose through the formation of 2,3-enol, which is converted by conjugated retroaldol reaction to methyl glyoxal or by tautomerism to 3-deoxyglucosone (Figure 22).
Fructose is undisputedly very important monosaccharide for his extended use as sweetener present in soft drinks and corn syrup, not to mention his presence in sucrose disaccharide. Fructose metabolism differs from glucose for his non dependence from insulin, and instead is phosphorylated to fructose-1-phosphate by fructokinase and ATP [38,39] (Figure 23).
On the other hand, glucose and fructose are interconnected trough the polyol pathway which has been highly associated with the generation of reactive oxygen species leading to tissue damage and apoptosis. An increase flux of glucose through this pathway is related with diabetes mellitus complications due the increase of NADH/NAD+ ratio and decrease of cytosolic NADPH which is required for regenerating glutathione (GSH) and important oxygen radical scavenger [40] (Figure 24).
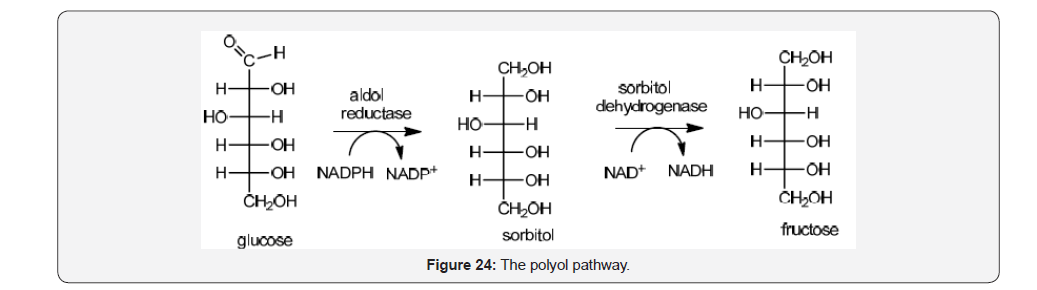
Advanced glycation end products (AGES)
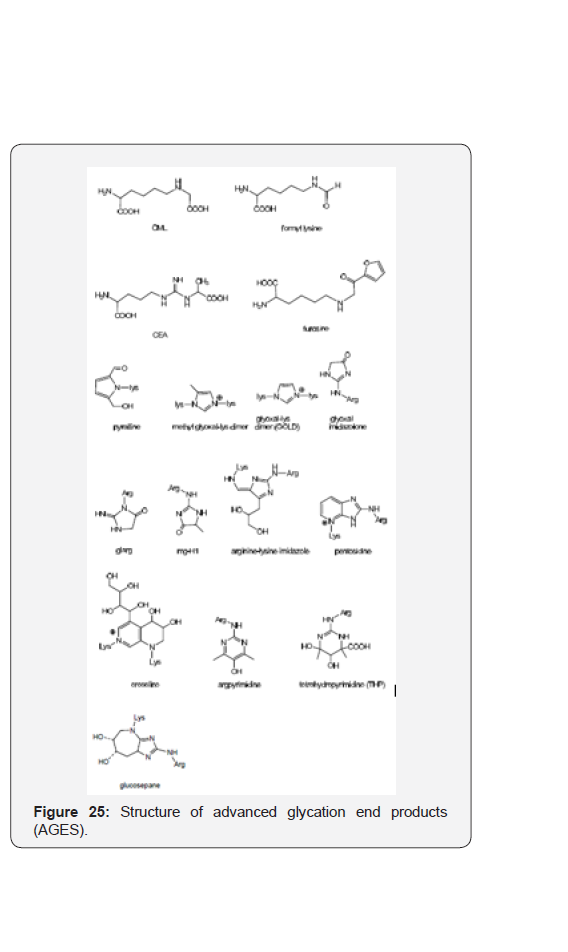
This term is referred to an array of compounds resulting from the condensation of amino acids mainly lysine and arginine with α-dicarbonyl products (glyoxal, methyl glyoxal), and carbohydrates. The conjugates that have been identified by high resolution mass spectrometry and rasonance spectroscopy includes open structures such as Nε- (carboxymethyl)lysine (CML), formyllysine, Nδ-(carboxyethyl)arginine (CEA), or 5 and 6 membered heterocyclic rings including pyrrole, imidazole, imidazolone, imidazo [4,5- b] pyridine, and tetrahydropyrimidin [41] (Figure 25).
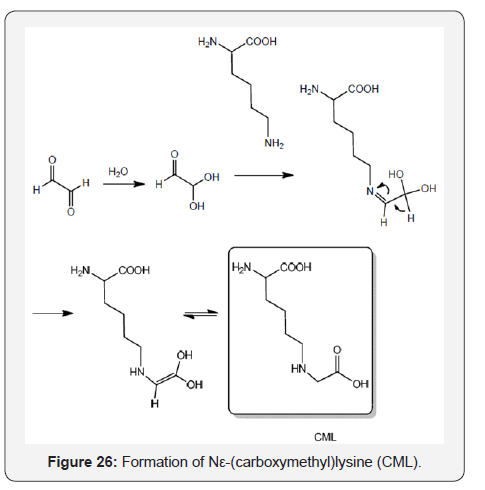
Nε-(carboxymethyl) lysine (CML) is a commo advanced glycation product found in foods described for over 30 years. It can be prepared from casein and glucose incubated at 95 °C for 8h [42]. According to the literature CML can be prepared in vivo from the Schiff base, the Amadori product, and from glyoxal with lysine [43] (Figure 26).
Pentosidine is a fluorescent imidazo [4,5-b] pyridine AGE adduct, [44] associated with diabetic complications, aging, kidney damage, and can be prepared from fructose, ascorbate, glucose, and ribose with lysine and arginine residues [45]. The structural assignment has been described by using mono and heteronuclear two dimensional 1H and 13CNMR spectroscopy [46] (Figure 27).
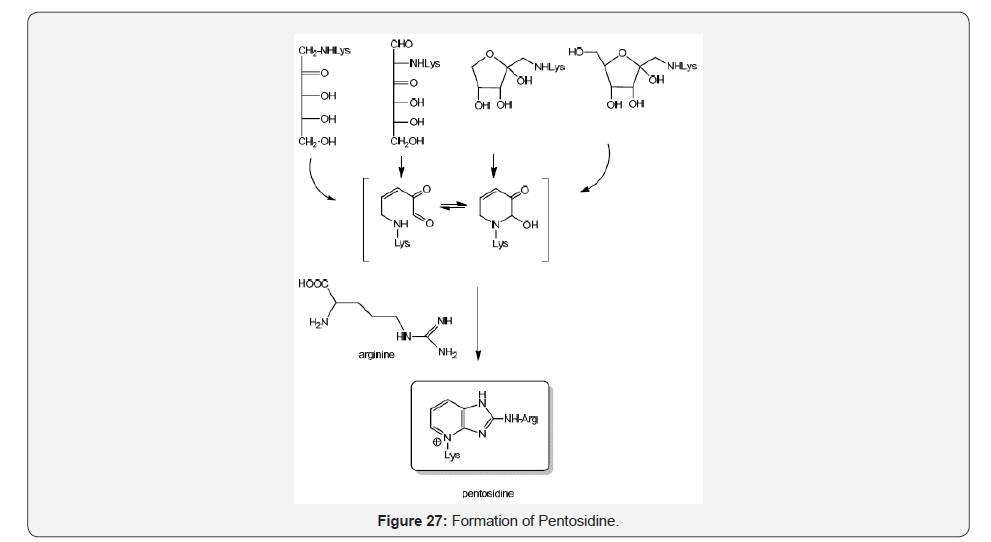
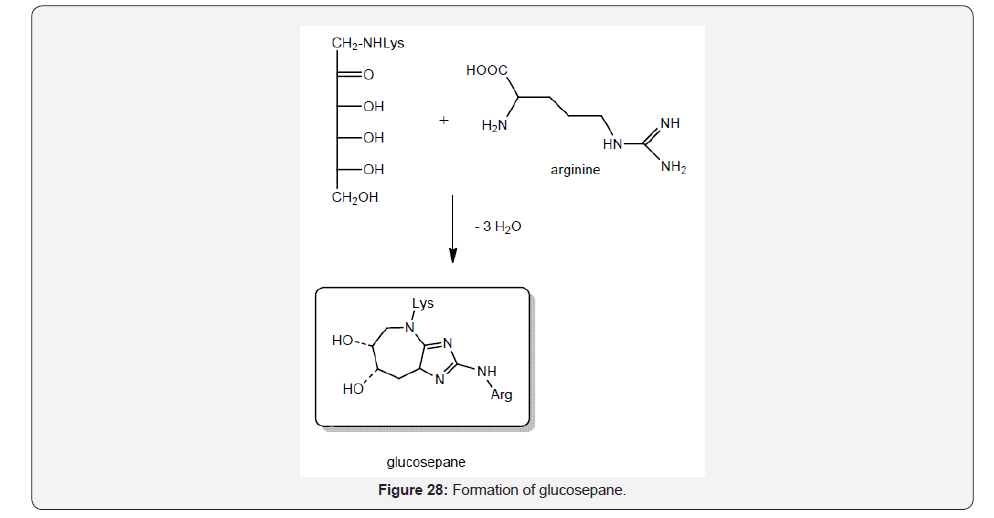
Glucosepane is an advanced gycation product less investigated with implications in retinopathy, neuropathy and nephropathy which is in vivo prepared by conjugation of arginine with Amadori adduct [46,47] (Figure 28).
Furosine is a ε-N-(furoylmethyl)-L-lysine formed by hydrolysis of the Amadori adducts fructosyl-lysisne, lactulosyl-lysisne and maltulosyl-lysisne under acid conditions, and it is produced by lysine conjugation with glucose, lactose and maltose [48] (Figure 29).
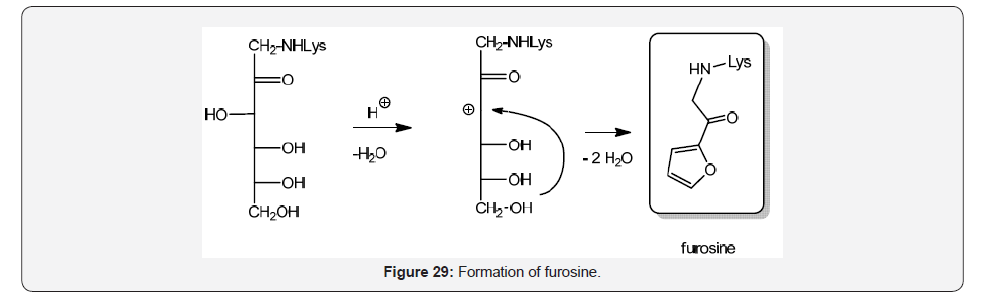
Methodology
The oxidative stress is design by the living systems for the regulation of important processes such as proliferation-differentiation, apoptosis, tissue homeostasis and self defense against pathogens, however when there is an imbalance between ROS production an anti-oxidative mechanism, a oxidative stress scenario occurs with severe consequences in the wellness of the living system deriving in pathologies that compromise the survival. Likewise glycoxidation is a process conceived to provide essential small molecules such as pyruvate from monosaccharide’s mainly glucose and fructose which are the main source of energy by the cell, however under hyperglycaemia the oxidative processes stimulate the production of highly reactive molecules such as glyoxal and methyl glyoxal which combined with proteins produce advanced glycation end-products responsible for expression of numerous degenerative disease.
Acknowledgments
The author would like to thank COFAA and SIP-IPN for financial support.
References
- Sies H (1997) Oxidative stress: oxidants and antioxidants. Exp Physiol 82(2): 291-295.
- Chen SK, Hsu CH, Tsai ML, Chen RH, Drummen GP (2013) Inhibition of Oxidative Stress by Low-Molecular-Weight Polysaccharides with Various Functional Groups in Skin Fibroblasts. Int J Mol Sci 14(10): 19399-19415.
- de M Bandeira S, da Fonseca LJ, da S Guedes G, Rabelo LA, Goulart MO, et al. (2013) Oxidative Stress as an Underlying Contributor in the Development of Chronic Complications in Diabetes Mellitus. Int J Mol Sci 14(2): 3265-3284.
- Brieger K, Schiavone S, Miller FJ Jr, Krause KH (2012) Reactive oxygen species: from health to disease. Swiss Med Wkly 142: 13659.
- Förstermann U, Sessa WC (2012) Nitric oxide synthases: regulation and function. Eur Heart J 33(7): 829-837.
- Wilson MI, Gill DJ, Perisic O, Quinn MT, Williams RL (2003) PB1 Domain-Mediated Heterodimerization in NADPH Oxidase and Signaling Complexes of Atypical Protein Kinase C with Par6 and p62. Mol Cell 12(1): 39-50.
- Panday A, Sahoo MK, Osorio D, Batra S (2015) NADPH oxidases: an overview from structure to innate immunity-associated pathologies. Cell Mol Immunol 12(1): 5-23.
- Groemping Y, Rittinger K (2005) Activation and assembly of the NADPH oxidase: a structural perspective. Biochem J 386: 401-416.
- Pauff JM, Zhang J, Bell CE, Hille R (2008) Substrate Orientation in Xanthine Oxidase crystal structure of enzyme in reaction with 2-hydroxy-6-methylpurine. J Biol Chem 283(8): 4818-4824.
- Cantu-Medellin N, Kelley EE (2013) Xanthine oxidoreductase-catalyzed reactive species generation: A process in critical need of reevaluation. Redox Biol 1: 353-358.
- Enroth C, Eger BT, Okamoto K, Nishinoi T, Nishino T, et al. (2000) Crystal structures of bovine milk xanthine dehydrogenase and xanthine oxidase: Structurebased mechanism of conversion. Proc Natl Acad Sci U S A 97(20): 10723-10728.
- Cao H, Pauff JM, Hille R (2014) X‑ray Crystal Structure of a Xanthine Oxidase Complex with the Flavonoid Inhibitor Quercetin. J Nat Prod 77(7): 1693-1699.
- Förstermann U, Sessa WC (2012) Nitric oxide synthases: regulation and function. Eur Heart J 33(7): 829-837.
- Wong CM, Wong KH, Chen XD (2008) Glucose oxidase: natural occurrence, function, properties and industrial applications. Appl Microbiol Biotechnol 78(6): 927-938.
- Petrović D, Frank D, Kamerlin SCL, Hoffmann K, Strodel B (2017) Shuffling Active Site Substate Populations Affects Catalytic Activity: The Case of Glucose Oxidase. ACS Catal 7(9): 6188-6197.
- Sono M, Roach MP, Coulter ED, Dawson JH (1996) Heme-Containing Oxygenases. Chem Rev 96(7): 2841-2887.
- Ulrich R, Hofrichter M (2007) Enzymatic hydroxylation of aromatic compounds. Cell Mol Life Sci 64(3): 271-293.
- Munro AW, McLean KJ, Grant JL, Makris TM (2018) Structure and function of the cytochrome P450 peroxygenase enzymes. Biochem Soc Trans 46(1): 183-196.
- Williams PA, Cosme J, Vinkovic DM, Ward A, Angove HC, et al. (2004) Crystal structures of human cytochrome P450 3A4 bound to metyrapone and progesterone. Science 305(5684): 683-686.
- Murphy MP (2009) How mitochondria produce reactive oxygen species. Biochem J 417(1): 1-13.
- Adam-Vizi, V, Chinopoulos C (2006) Bioenergetics and the formation of mitochondrial reactive oxygen species. Trends Pharmacol Sci 27(12): 639-645.
- Kudin AP, Bimpong-Buta NY, Vielhaber S, Elger CE, Kunz WS (2004) Characterization of superoxide-producing sites in isolated brain mitochondria. J Biol Chem 279(6): 4127-4135.
- Votyakova TV, Reynolds IJ (2001) ψm-Dependent and-independent production of reactive oxygen species by rat brain mitochondria. J Neurochem 79: 266-277.
- Chew GT, Watts GF (2004) Coenzyme Q10 and diabetic endotheliopathy: oxidative stress and the ‘recoupling hypothesis’. QJM 97(8): 537-548.
- Schrader M, Fahimi HD (2006) Peroxisomes and oxidative stress. Biochimica et Biophysica Acta 1763(12): 1755-1766.
- Choe E, Min DB (2005) Chemistry and Reactions of Reactive Oxigen Species in Foods. Journal of Food Sciences 70(9): 142-159.
- Elias RJ, Waterhouse AL (2010) Controlling the Fenton Reaction in Wine. J Agric Food Chem 58(3): 1699-1707.
- Brito-Arias M (2016) Synthesis and Characterization of Glycosides. Second Edition Springer International, 10-11.
- Semchyshyn HM, Lozinska LM, Miedzobrodzki J, Lushchak VI (2011) Fructose and glucose differentially affect aging and carbonyl/oxidative stress parameters in Saccharomyces cerevisiae cells. Carbohydr Res 346(7): 933-938.
- Saraswathi NT, Syakhovich VE, Bokut, SB, Moras, D, Ruff M (2008) Crystal Structure of Glycated Human Haemoglobin.
- Horvat S, Roscić M (2010) Glycosylation of lysine-containing pentapeptides by glucuronic acid: new insights into the Maillard reaction. Carbohydr Res 345(3): 377-384.
- Lederer MO, Dreisbusch CM, Bundschuh RM (1997) Amadori products from model reactions of D-glucose with phosphatidyl ethanolamine Independent synthesis and identification of 1 -deoxy- 1-t 2-hydroxyethylamino)-D-fructose derivatives. Carbohydrate Research 301: 111-121.
- Ahmad S, Khan MS, Akhter F, Khan MS, Khan A, et al. (2014) Glycoxidation of biological macromolecules: A critical approach to halt the menace of glycation. Glycobiology 24(11): 979-990.
- Marnett LJ (2002) Oxy radicals, lipid peroxidation and DNA damage. Toxicology181-182: 219-222.
- Chetyrkin S, Mathis M, Pedchenko V, Sanchez OA, Hayes W, et al. (2011) Glucose Autoxidation Induces Functional Damage to Proteins via Modification of Critical Arginine residues. Biochemistry 50(27): 6102-6112.
- Thornalley PJ (1985) Monosaccharide Autooxidation in Health and Disease. Environ Health Perspect 64: 297-307.
- Thornalley PJ, Langsborg A, Minhas HS (1999) Formation of glyoxal, methyl glyoxal and 3-deoxyglucosone in the glycation of proteins by glucose. Biochem J 344: 109-116.
- Yang K, Qiang D, Delaney S, Mehta S, Bruce WR, et al. (2011) Differences in glyoxal and methylglyoxal metabolism determine cellular susceptibility to protein carbonylation and cytotoxicity. Chem Biol Interact 191(1-3): 322-329.
- Gugliucci A (2017) Formation of Fructose-Mediated Advanced Glycation End Products and Their Roles in Metabolic and Inflammatory Diseases. Adv Nutr 8(1): 54-62.
- Dodda D, Ciddi V (2014) Plants used in the management of diabetic complications. Indian J Pharm Sci 76(2): 97-106.
- Soboleva A, Schmidt R, Vikhnina M, Grishina T, Frolov A (2017) Maillard Proteomics: Opening New Pages. Int J Mol Sci 18(12): 2677.
- Lima M, Assar SH, Ames JM (2010) Formation of N(epsilon)-(Carboxymethyl)lysine and loss of Lysisne in Casein Glucose-Fatty Acid Model System. J Agric Food Chem 58(3): 1954-1958.
- Ferreira AE, Ponces Freire AM, Voit EO (2003) A Quantitative Model of the Generation of N - (Carboxymethyl) lysine in the Maillard Reaction Between Collagen and Glucose. Biochem J 376: 109.
- Rosenberg AJ, Clark DA (2012) The Total Synthesis of Pentosidine. Org Lett 14(17): 4678-4681.
- Grandhee SK, Monnier VM (1991) Mechanism of formation of the Maillard Protein Crosslink Pentosidine. J Biol Chem 266(18): 11649-11653.
- Biemel KM, Reihl O, Conrad J, Lederer MO (2001) Formation Pathways for Lysine-Arginine Cross-links Derived from Hexoses and Pentoses by Maillard Processes. J Biol Chem 276(26): 23405-23412.
- Monnier VM, Sun W, Sell DR, Fan X, Nemet I, et al. (2014) Glucosepane: a poorly understood advanced glycation end product of growing importance for diabetes and its complications. Clin Chem Lab Med: 52(1): 21-32.
- Rufián HJA, Guera HE, García VB (2004) Pyrraline content in enteral formula processing and storage and model systems. Eur Food Res Technol 219(1): 42-47.
- Sies H (1997) Oxidative stress: oxidants and antioxidants. Exp Physiol 82(2): 291-295.
- Chen SK, Hsu CH, Tsai ML, Chen RH, Drummen GP (2013) Inhibition of Oxidative Stress by Low-Molecular-Weight Polysaccharides with Various Functional Groups in Skin Fibroblasts. Int J Mol Sci 14(10): 19399-19415.
- de M Bandeira S, da Fonseca LJ, da S Guedes G, Rabelo LA, Goulart MO, et al. (2013) Oxidative Stress as an Underlying Contributor in the Development of Chronic Complications in Diabetes Mellitus. Int J Mol Sci 14(2): 3265-3284.
- Brieger K, Schiavone S, Miller FJ Jr, Krause KH (2012) Reactive oxygen species: from health to disease. Swiss Med Wkly 142: 13659.
- Förstermann U, Sessa WC (2012) Nitric oxide synthases: regulation and function. Eur Heart J 33(7): 829-837.
- Wilson MI, Gill DJ, Perisic O, Quinn MT, Williams RL (2003) PB1 Domain-Mediated Heterodimerization in NADPH Oxidase and Signaling Complexes of Atypical Protein Kinase C with Par6 and p62. Mol Cell 12(1): 39-50.
- Panday A, Sahoo MK, Osorio D, Batra S (2015) NADPH oxidases: an overview from structure to innate immunity-associated pathologies. Cell Mol Immunol 12(1): 5-23.
- Groemping Y, Rittinger K (2005) Activation and assembly of the NADPH oxidase: a structural perspective. Biochem J 386: 401-416.
- Pauff JM, Zhang J, Bell CE, Hille R (2008) Substrate Orientation in Xanthine Oxidase crystal structure of enzyme in reaction with 2-hydroxy-6-methylpurine. J Biol Chem 283(8): 4818-4824.
- Cantu-Medellin N, Kelley EE (2013) Xanthine oxidoreductase-catalyzed reactive species generation: A process in critical need of reevaluation. Redox Biol 1: 353-358.
- Enroth C, Eger BT, Okamoto K, Nishinoi T, Nishino T, et al. (2000) Crystal structures of bovine milk xanthine dehydrogenase and xanthine oxidase: Structurebased mechanism of conversion. Proc Natl Acad Sci U S A 97(20): 10723-10728.
- Cao H, Pauff JM, Hille R (2014) X‑ray Crystal Structure of a Xanthine Oxidase Complex with the Flavonoid Inhibitor Quercetin. J Nat Prod 77(7): 1693-1699.
- Förstermann U, Sessa WC (2012) Nitric oxide synthases: regulation and function. Eur Heart J 33(7): 829-837.
- Wong CM, Wong KH, Chen XD (2008) Glucose oxidase: natural occurrence, function, properties and industrial applications. Appl Microbiol Biotechnol 78(6): 927-938.
- Petrović D, Frank D, Kamerlin SCL, Hoffmann K, Strodel B (2017) Shuffling Active Site Substate Populations Affects Catalytic Activity: The Case of Glucose Oxidase. ACS Catal 7(9): 6188-6197.
- Sono M, Roach MP, Coulter ED, Dawson JH (1996) Heme-Containing Oxygenases. Chem Rev 96(7): 2841-2887.
- Ulrich R, Hofrichter M (2007) Enzymatic hydroxylation of aromatic compounds. Cell Mol Life Sci 64(3): 271-293.
- Munro AW, McLean KJ, Grant JL, Makris TM (2018) Structure and function of the cytochrome P450 peroxygenase enzymes. Biochem Soc Trans 46(1): 183-196.
- Williams PA, Cosme J, Vinkovic DM, Ward A, Angove HC, et al. (2004) Crystal structures of human cytochrome P450 3A4 bound to metyrapone and progesterone. Science 305(5684): 683-686.
- Murphy MP (2009) How mitochondria produce reactive oxygen species. Biochem J 417(1): 1-13.
- Adam-Vizi, V, Chinopoulos C (2006) Bioenergetics and the formation of mitochondrial reactive oxygen species. Trends Pharmacol Sci 27(12): 639-645.
- Kudin AP, Bimpong-Buta NY, Vielhaber S, Elger CE, Kunz WS (2004) Characterization of superoxide-producing sites in isolated brain mitochondria. J Biol Chem 279(6): 4127-4135.
- Votyakova TV, Reynolds IJ (2001) ψm-Dependent and-independent production of reactive oxygen species by rat brain mitochondria. J Neurochem 79: 266-277.
- Chew GT, Watts GF (2004) Coenzyme Q10 and diabetic endotheliopathy: oxidative stress and the ‘recoupling hypothesis’. QJM 97(8): 537-548.
- Schrader M, Fahimi HD (2006) Peroxisomes and oxidative stress. Biochimica et Biophysica Acta 1763(12): 1755-1766.
- Choe E, Min DB (2005) Chemistry and Reactions of Reactive Oxigen Species in Foods. Journal of Food Sciences 70(9): 142-159.
- Elias RJ, Waterhouse AL (2010) Controlling the Fenton Reaction in Wine. J Agric Food Chem 58(3): 1699-1707.
- Brito-Arias M (2016) Synthesis and Characterization of Glycosides. Second Edition Springer International, 10-11.
- Semchyshyn HM, Lozinska LM, Miedzobrodzki J, Lushchak VI (2011) Fructose and glucose differentially affect aging and carbonyl/oxidative stress parameters in Saccharomyces cerevisiae cells. Carbohydr Res 346(7): 933-938.
- Saraswathi NT, Syakhovich VE, Bokut, SB, Moras, D, Ruff M (2008) Crystal Structure of Glycated Human Haemoglobin.
- Horvat S, Roscić M (2010) Glycosylation of lysine-containing pentapeptides by glucuronic acid: new insights into the Maillard reaction. Carbohydr Res 345(3): 377-384.
- Lederer MO, Dreisbusch CM, Bundschuh RM (1997) Amadori products from model reactions of D-glucose with phosphatidyl ethanolamine Independent synthesis and identification of 1 -deoxy- 1-t 2-hydroxyethylamino)-D-fructose derivatives. Carbohydrate Research 301: 111-121.
- Ahmad S, Khan MS, Akhter F, Khan MS, Khan A, et al. (2014) Glycoxidation of biological macromolecules: A critical approach to halt the menace of glycation. Glycobiology 24(11): 979-990.
- Marnett LJ (2002) Oxy radicals, lipid peroxidation and DNA damage. Toxicology181-182: 219-222.
- Chetyrkin S, Mathis M, Pedchenko V, Sanchez OA, Hayes W, et al. (2011) Glucose Autoxidation Induces Functional Damage to Proteins via Modification of Critical Arginine residues. Biochemistry 50(27): 6102-6112.
- Thornalley PJ (1985) Monosaccharide Autooxidation in Health and Disease. Environ Health Perspect 64: 297-307.
- Thornalley PJ, Langsborg A, Minhas HS (1999) Formation of glyoxal, methyl glyoxal and 3-deoxyglucosone in the glycation of proteins by glucose. Biochem J 344: 109-116.
- Yang K, Qiang D, Delaney S, Mehta S, Bruce WR, et al. (2011) Differences in glyoxal and methylglyoxal metabolism determine cellular susceptibility to protein carbonylation and cytotoxicity. Chem Biol Interact 191(1-3): 322-329.
- Gugliucci A (2017) Formation of Fructose-Mediated Advanced Glycation End Products and Their Roles in Metabolic and Inflammatory Diseases. Adv Nutr 8(1): 54-62.
- Dodda D, Ciddi V (2014) Plants used in the management of diabetic complications. Indian J Pharm Sci 76(2): 97-106.
- Soboleva A, Schmidt R, Vikhnina M, Grishina T, Frolov A (2017) Maillard Proteomics: Opening New Pages. Int J Mol Sci 18(12): 2677.
- Lima M, Assar SH, Ames JM (2010) Formation of N(epsilon)-(Carboxymethyl)lysine and loss of Lysisne in Casein Glucose-Fatty Acid Model System. J Agric Food Chem 58(3): 1954-1958.
- Ferreira AE, Ponces Freire AM, Voit EO (2003) A Quantitative Model of the Generation of N - (Carboxymethyl) lysine in the Maillard Reaction Between Collagen and Glucose. Biochem J 376: 109.
- Rosenberg AJ, Clark DA (2012) The Total Synthesis of Pentosidine. Org Lett 14(17): 4678-4681.
- Grandhee SK, Monnier VM (1991) Mechanism of formation of the Maillard Protein Crosslink Pentosidine. J Biol Chem 266(18): 11649-11653.
- Biemel KM, Reihl O, Conrad J, Lederer MO (2001) Formation Pathways for Lysine-Arginine Cross-links Derived from Hexoses and Pentoses by Maillard Processes. J Biol Chem 276(26): 23405-23412.
- Monnier VM, Sun W, Sell DR, Fan X, Nemet I, et al. (2014) Glucosepane: a poorly understood advanced glycation end product of growing importance for diabetes and its complications. Clin Chem Lab Med: 52(1): 21-32.
- Rufián HJA, Guera HE, García VB (2004) Pyrraline content in enteral formula processing and storage and model systems. Eur Food Res Technol 219(1): 42-47.