The Use of Rosenmund Reduction in Organic Synthesis
Ajoy K Banerjee1*, Lisbeth Mendoza1, Liadis Bedoya1 and Elvia V Cabreram2,3
1Chemistry Center, Venezuelan Institute of Scientific Research (IVIC), Caracas-1020A, Venezuela
2Chemical Engineering Faculty, Central University of Ecuador, Quito-170129, Ecuador
3Molecules and Functional Materials Research Group (MoleMater), Av. universitaria, Quito-170129, Ecuador
Submission: March 28, 2023;Published: April 13, 2023
*Corresponding author: Ajoy K Banerjee, Centro de Quimica, IVIC, Caracas-1020A, Venezuela
How to cite this article: Ajoy K Banerjee*, Lisbeth Mendoza, Liadis Bedoya and Elvia V Cabrera. The Use of Rosenmund Reduction in Organic Synthesis. Organic & Medicinal Chem IJ. 2023; 12(2): 555832. DOI: 10.19080/OMCIJ.2023.12.555832
Abstract
The diverse uses of commercially available Palladium (Pd) on barium sulfate, known as Rosenmund catalyst are described. Hydrogenation of acid chloride to aldehyde with poisoned palladium catalyst (Pd-BaSO4) is known as Rosenmund reduction. The Rosenmund reduction has also been used in the conversion of alkynes to cis-alkenes and hydrogenolysis of various functional groups. The regioselective opening of 1,2-disubstituted epoxide has been recorded with Rosenmund reduction.
Keywords: Rosenmund reduction; Palladium bisulfate; Quinoline; Sulfur
Introduction
The palladium catalyzed hydrogenation has played an important role in organic synthesis [1-3]. Palladium catalyst resembles platinum catalyst in activity and usefulness. It increases the contact of the catalyst with hydrogen. Palladium supported catalysts commonly used are palladium on charcoal, calcium carbonate or barium sulfate. The palladium catalyzed hydrogenation of acid chloride 1 in presence of barium sulfate to an aldehyde 2 is known as Rosenmund reduction [4,5]. Pd on BaSO4 commonly used contains palladium 5%, occasionally 2-3%. Some acid chloride undergoes reductive removal of the -COCl group to yield hydrocarbon 3 (Scheme 1).
Mechanism
It is presumed that the acid chloride 1 forms an organopalladium spicies 4 which reacts with hydrogen to form the intermediate 4(i) and this on reductive elimination yields the aldehyde 2 [5] (Scheme 2).
Discussion
The reduction is carried out by bubbling hydrogen through which a hot solution of acid chloride 1 in which the catalyst is suspended in xylene or toluene. This process removes the hydrogen chloride and the aldehyde 2 is isolated. Better yields (around 90%) may be obtained by adding a base, e.g., quinoline to the reaction mixture in order to remove the hydrogen chloride [6]. A number of side reactions may be observed with the Rosenmund reduction which lowers the yield of the aldehyde. The catalyst may reduce the aldehyde 2 to the alcohol 5 which may react with aceylchloride 1 to yield to yield an ester 6 (Scheme 3). A small amount of water present causes partial hydrolysis to the acyl chloride to yield acid 7 which may react with acetyl chloride 1 to give a carboxylic anhydride 8.
It is not possible to generalize concerning the extent to which reducible groups other than the –COCl may interfere with the Rosenmund reduction. The yield of the aldehyde in Rosenmund reduction varies greatly. Most of the acid chlorides give aldehyde over 50%. The yield over 80% are not uncommon. Some acid chlorides contain very little aldehyde while with some acid chlorides, no aldehyde is obtained. The reduction of the aldehyde to the alcohol can be prevented by the use of an appropriate “catalyst” or regulator which inactivates the catalyst towards reduction of aldehyde but not acid chloride. The commonly used regulator is quinolone-sulfur [7,8]. Other regulators which have been recommended are pure thiourea and tetramethylthio urea [7,9]. The amount of regulator reported varies widely. About 10 mg of “quinolone-S” per gram of catalyst per gram of catalyst is quite satisfactory [10].
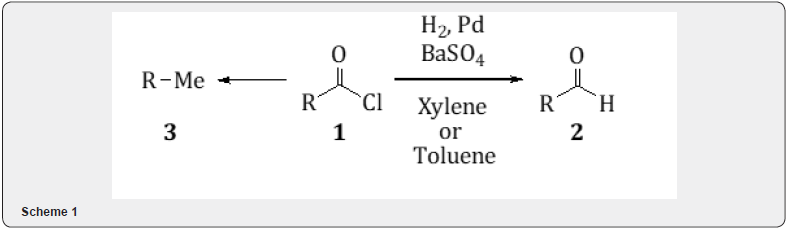
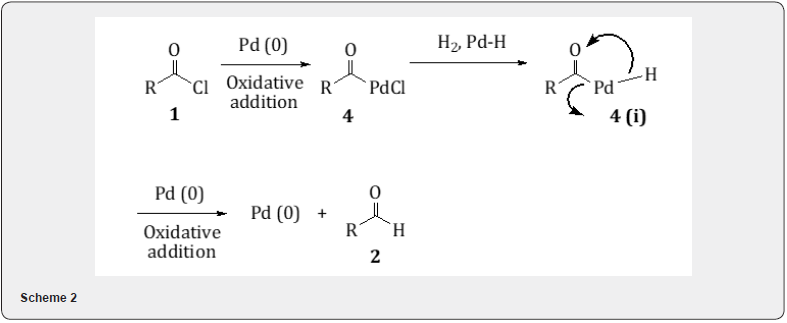
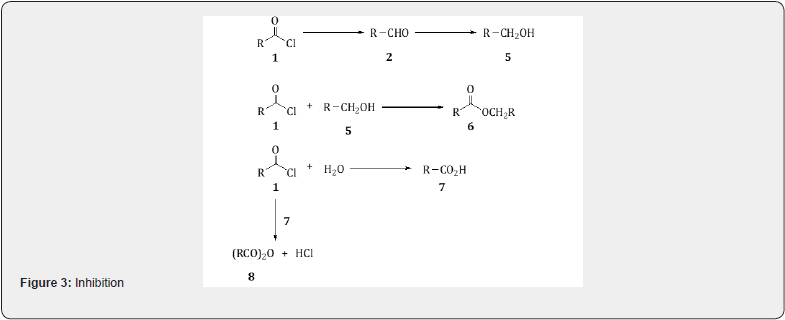
Whenever the necessity of a regulator arises, it is recommended to use freshly prepared “quinoline-S” for the sake of reproducibility. It is known that the “poison” blocks the most active catalyst sities and thus prevents undesired side reactions but the recent observation of Maier and collaborators [11] have shown that the poisons used to deactivate palladium catalyst do not block the active sites but rearranges the surface structure of the palladium. In many reductions, however, it is not necessary to use a regulator and in some reductions the use of poison appears to be definitely disadvantageous. The decisive factor in the acid chloride reduction is the temperature [12]. If the temperature is kept near the lowest point at which hydrogen chloride is evolved, the aldehydes are obtained in optimum yields. It has been observed that one equivalent of 2,6-dimethylpyridine with acyl chloride in a solution of tetrahydrofuran sufficiently dilute (˂0.25M) to prevent poisoning of the palladium catalyst provides a generally faster and superior method that is especially suitable for sensitive aldehydes [6].
Depending on the amount and activity of the catalyst, the hydrogenation is usually complete in 1-2 h at room temperature or below, except for aromatic acyl chlorides, which require a higher temperature with a hydrocarbon solvent and a quinoline-S poisoned catalyst. The method gives excellent yield and is appreciable to acyl chlorides that give poor results by the standard Rosenmund or other procedures. The use of 3-methyl-1-phenyl- 2-phospholine 9 allows an alternative for the acid reduction of aromatic acid chloride 10.The intermediate 11 on treatment with water forms the complex 11(i) which gets decomposed to the aldehyde 12 (scheme 4) [13]. Nitro and hydroxy-acid chlorides appear to form a complex with 9 which are not decomposed by water to give aldehydes. The corresponding reaction with aliphatic acid chlorides give the acid and free phospholine 9.
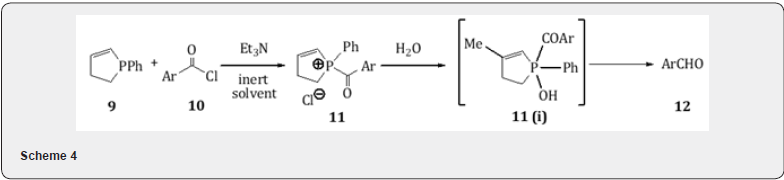
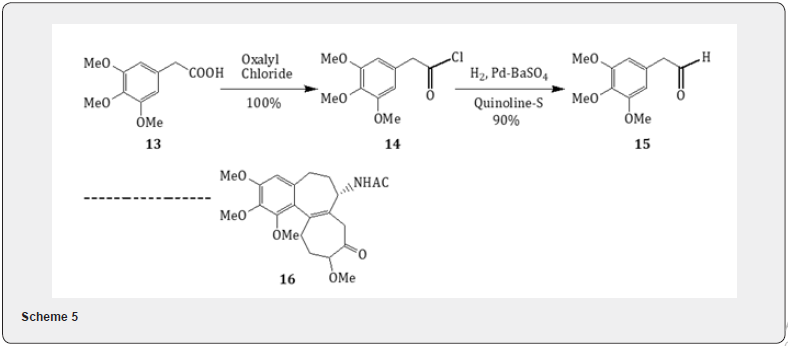
Applications
Several applications of Rosenmund reduction have been givessrved during the synthesis of organic compounds and natural products.The principal use of the Rosenmund reduction consists in the selective hydrogenation of an acyl chloride to the corresponding aldehyde. Through the following examples it will be easy to appreciate the importance of the Rosenmund catalyst in organic synthesis.
(i) Synthesis of Colchinine
Rosenmund reduction has been applied for the synthesis [14] of the alkaloid colchicine as described in Scheme 5. The acid 13, commercially available, was converted to acid chloride 14 by the treatment with oxalyl chloride and pyridine. The conversion of the acid chloride to the aldehyde 15 was accomplished by Rosenmund catalyst. The aldehyde has proved to be a potential intermediate for the synthesis of the alkaloid colchicine 16 which is used in the treatment of gout and exhibits mitosis in biological research.
(ii) Synthesis of 2-Oxoethoxycoumarins
Rosenmund reduction has been frequently used for the synthesis of
2-oxoethoxycoumarins [15]. The hydroxycoumarin 17 on alkylation with ethylbromoacetate 18 yield ester (98%) which on alkaline hydrolysis yields the acid 19 (100%). Treatment with thionyl chloride leads to the formation of chloride 20 . The catalytic hydrogenation of the chloride using Rosenmund catalyst leads the formation of the 7-(2-oxoethoxy)coumarin 21 as shown in Scheme 6. Similarly, alkylation of hydroxycoumarin 22 with 2-bromopropionate 23 followed by alkaline hydrolysis leads the formation of acid whose acid chloride 24 is converted into 4-(2-oxoethoxy)-coumarin 25 by Rosenmund reduction as exhibited in Scheme 7. These oxoethoxy coumarins are useful for the synthesis of natural products such as geiparvarin and psoralens.
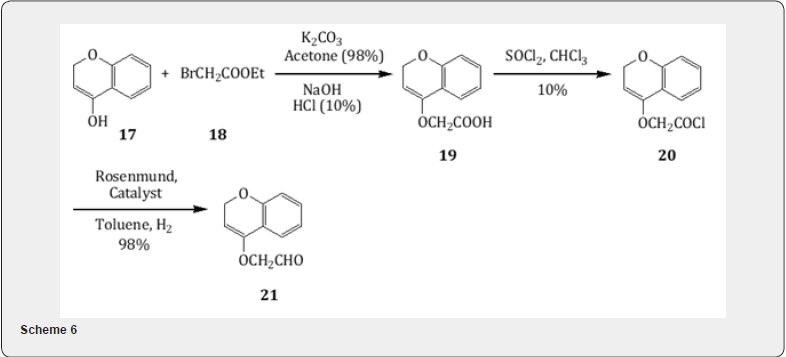
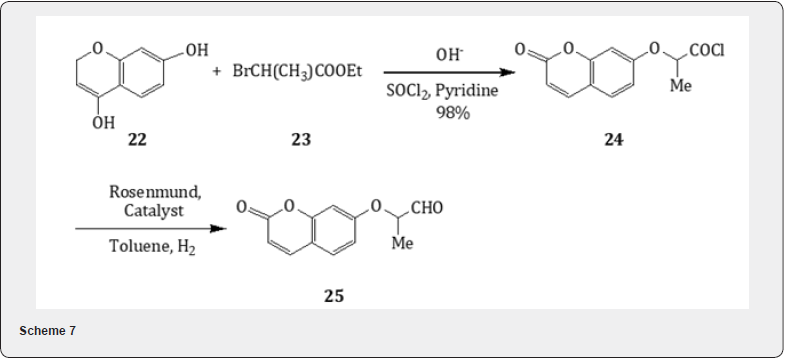
(iii) Synthesis Of Psoralens
The Rosenmund reduction has been utilized for the synthesis of psoralens [16] known as furocoumarins which are naturally occurring tricyclic aromatic compounds and exhibit interesting photobiological activities . Following the method [17] the easily available 7-hydroxy coumarins (26-29) have been converted into the ring substituted 2-(coumarin-7-yl)oxyaldehydes (30 -33) in excellent yield (Scheme 8). Chimichi and collaborators have observed [16] that the oxyaldehydes 30-33 on treatment with alkaline solution undergo cyclization leading the formation of a mixture of linear psoralens 34-37 and angular psoralens 38- 41 (Scheme 9) respectively. It is worthwhile to mention that the aldehyde [16] 42 on treatment with base affords furocoumarin sphondin 43 in poor yield (4-5%). The yield could not be improved under various conditions. Spondin is a natural product useful for its antifungal activity.
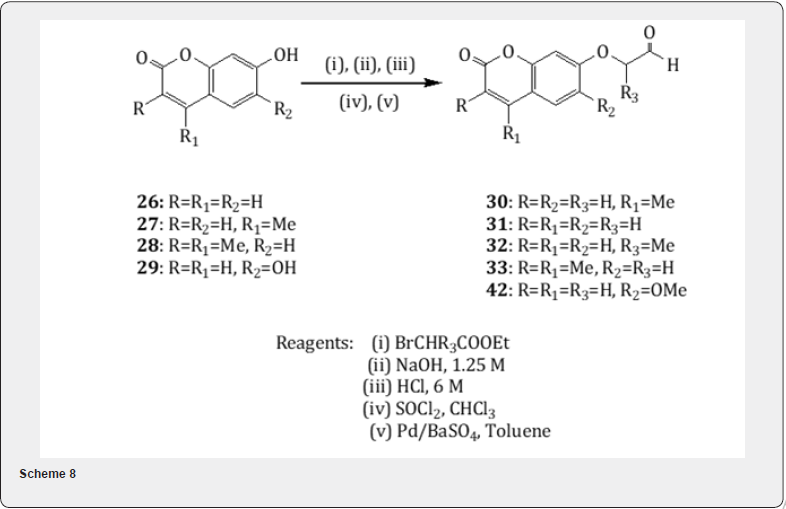
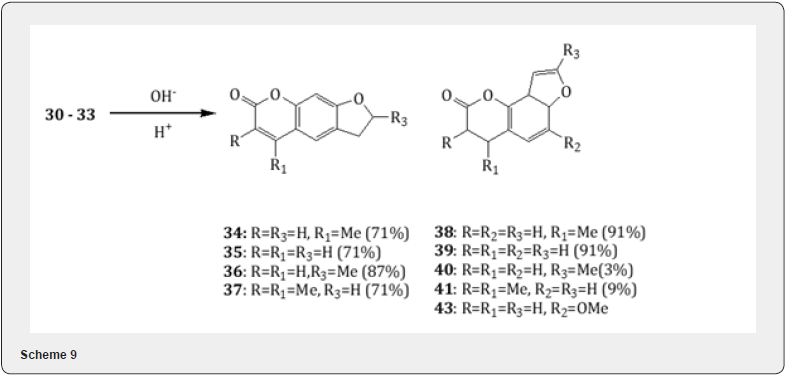
(iv) Synthesis of 10-Undecenal
Yadav and Chandalia [18] have applied Rosenmund reduction for the synthesis of perfumery aldehyde 10-undecenal 46 (Scheme 10). Thus, the undecanoic acid 44 , a castor oil based product, is converted acid chloride 45 by the treatment with thionyl chloride and N,N-dimethylformamide. The transformation of the chloride 45 to the aldehyde 46 is accomplished by Rosenmund reduction. The main byproduct is the alcohol 47 which is formed by the subsequent reduction of the aldehyde 46. Some amount of saturated alcohol 48 is also formed. The formation of alcohol 47 can be prevented by the use of catalyst poison or regulator [19] such as quinoline-sulfur which deactivates the catalyst and thus suppresses the formation of the alcohol and increases the selectivity to the aldehyde. It has been observed that the Rosenmund hydrogenolysis of the chloride 45 can be conveniently carried out at atmospheric pressure and in the temperature range 30-90oC by using low-boiling solvents such as acetone and ethyl acetate in presence of 5% Pd/C without an inhibitor like-quinoline sulfur with as high as 93% selectivity to the 10-undecenal 46. The method does not reduce the activity of the catalyst. In addition, the conventional catalyst (Pd on activated charcoal, barium sulfate etc) Pd supported on polymers [20] has been utilized to perform the Rosenmund reduction. The catalyst 5% (Pd-PPTA) [PPTApoly( p-phenylene terephthalimide)] has also been considered but less active. The catalyst 5% Pd/C gives 53% overall conversion in 2 h while a comparable conversion of 56% is obtained in 6 h with 5% Pd/PPTA. It is interesting to note with 5%Pd/C the major side product is the unsaturated alcohol 48 whereas with 5% Pd/PPTA the side product is the saturated aldehyde 49. The use of acetone, ethylacetate and toluene gives comparable results. The catalyst 5% Pd/C can be used four times with no significant effect on the conversion to and the yield of the unsaturated aldehyde.The Rosenmund reduction has also been tried with p-anisoyl chloride and terephthaloyl chloride.
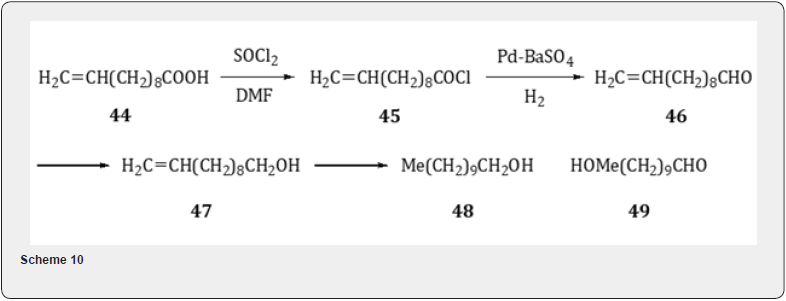
(v) Synthesis Of M-Phenoxybenzaldehyde
Rosenmund reduction has also been utilized for the synthesis [21] of m-phenoxy benzaldehyde (MPB) which is an important intermediate for the manufacture of synthetic pyrethroids . The pyrethroids possess high negative insecticidal activity for army worms and also are herbicidal antidotes for cotton and legumes. The synthetic details of MPB are given below:Ullmann condensation of the potassium salt of m-cresol 50 with chlorobenzene 51 (Scheme 11) in presence of copper salt and polyethylene glycol (PEG) as cosolvent gives 86% conversion m-phenoxytoluene 52 (MPT) with 97% selectivity in 12 hr. The rate of reaction decreases towards the end of reaction due to salt effect of potassium chloride. The formation of the residue is less than 3% when the reaction is carried out for 12 hr at 250oC. In order to achieve the conversion of MPT to MPB ,to a solution of MPT in acetic acid air is bubbled in presence of cobalt acetate and sodium bromide acetate as catalyst and sodium bromide as catalyst promoter. The major products obtained are identified as carboxylic acid 53, aldehyde 54 and acetate 55. The carboxylic acid 53 in toluene is converted to acid chloride by thionyl chloride and N,N-dimethyl- formamide which on hydrogenation with Pd/C under Rosenmund reduction leads 93% of formation m-phenoxybenzaldehyde 54 with a selectivity of 86% at 10% substrate concentration. With the increase in substrate concentra- tion the conversion to the aldehyde gets decreased. The catalyst Pd/C can be reused three times with marginal decrease in the conversion level.
(vi) Synthesis of Atovaquone
Rosenmund reduction proved useful for the synthesis [22] of Atovaquone, whose trade name is Mepron and used for the treatment and prophylaxis of Pneumocystis carnii infection. In order to develop an alternative synthesis of Atavaquone, the carboxylic acid 56 is treated with oxalyl chloride and catalytic amount dimethyl formamide and ethylacetate. The resulting chloride is subjected to Rosenmund reduction to obtain aldehyde 57. Standard palladium on charcoal has proved a superior choice to the classic poisoned palladium on barium sulfate catalyst due to the relative stability of the aliphatic acid chloride. The condensation of the aldehyde 57 with 1,4-isochromandione 58 using isobutylamine and acetic acid yields the conjugated amine 59 which is converted to atovaquone 60 by treatment with sodium methoxide in methanol (Scheme 12). The 1,4-isochromandione 58 is prepared (Scheme 13) by bromination of 2-acetyl benzoic acid 60 with bromine and chlorobenzene. Once the bromination is complete, water is added to the brominated products 61 and 62. The resulting biphasic mixture is heated to obtain the dione 58 (Scheme 13).
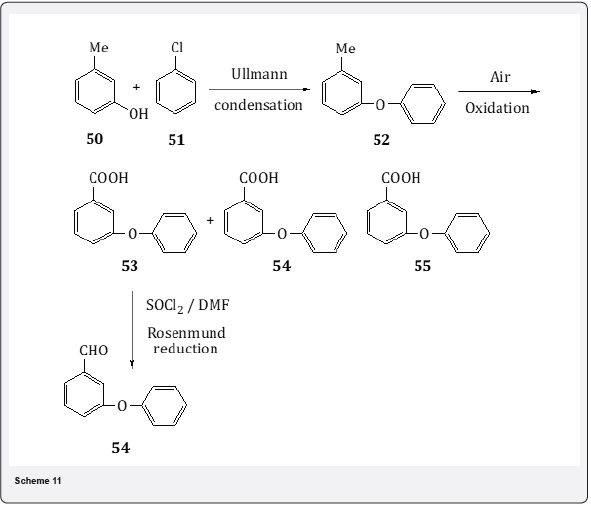
Conclusion
A brief description of Rosenmund reduction Is described. It is necessary to know that exist many methods for the conversion of the carboxylic acid to aldehyde and therefore the the Rosenmund reduction does not enjoy the same importance as it enjoyed several years ago. Hersberg and Cason [23] recommended the addition of poison to ensure controlled conditions. The omission of catalyst poisons is common and the original Rosenmund procedure[4] has been successful with acid halides containing other functional groups or condensed benzenoid or heterocyclic systems [5] .Danishefsky and collaborators [24] following the original Rosenmund procedure have converted acid chloride to aldehyde in quantitative yield. The amine modified Rosenmund reduction has been studied [25]. Both aliphatic and aromatic acid chlorides are reduced smoothly at room temperature and atmospheric pressure to aldehydes with 10% Pd/C as catalyst, acetone or ethyl acetate as solvent and ethyldiisiporopylamine as HCl acceptor. The reaction proceeds with high selectivity, over reduction is less than 1% and nitro and chloro substituents in benzoyl chloride are unaffected as is double bond in cinnamoyl chloride. The broad range of poisons used to cause identical or similar modifications of the catalytic activity of the supported palladium to give Rosenmund catalysts is suggestive that the poisons are not involved in the catalytic process but rather change the surface of the catalyst. It has been recorded that “poison” blocks the most active sites of catalyst and thus prevents undesired further reactions. Maier and collaborators [11] have shown that the poisons do not block certain active sites but rather act to rearrange the palladium structure in a very drastic way. A detailed description on the use of the Rosenmund reduction can be obtained in an article of Siegel [26].
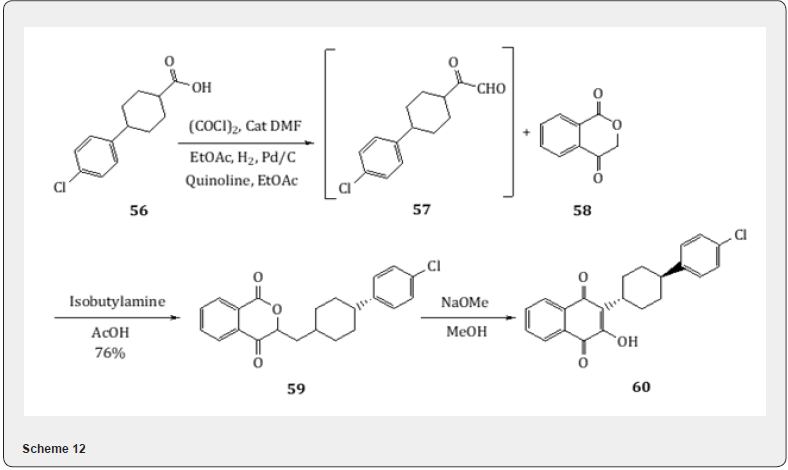
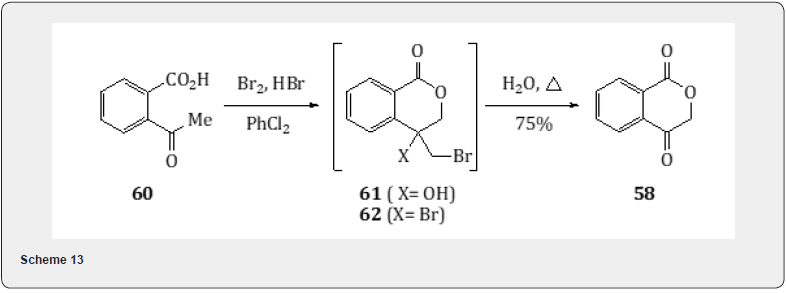
References
- Tsuji J (1980) Organic Synthesis with Palladium Compounds, Springer, Berlin, Germany.
- Heck RF (1985) Palladium Reagents in Organic Synthesis. New York, United States.
- Tsuji J (1995) Palladium Reagents and Catalysis. Wiley, New York, United States.
- Rosenmund KW (1918) Uber eine neue methode zur dartstellung von aldehyden. 1. mitteilung Ber 54: 585-593.
- Mosettig E, Mozingo R (1948) The Rosenmund reduction of acid chlorides to aldehydes. Org Reaction 4: 362-377.
- Burgstahler AW, Weigel LO, Shaefer CG (1976) Improved modification of the Rosenmund reduction. Synthesis:767-768.
- Rosenmund KW, Zetzsche F (1921) Uber katalysator-beeinflussung und spezifisch wirkende katalysatoren. Chem. Ber 54: 425-437.
- Rosenmund KW, Zetzsche F , Heise F(1921) Die beeinflussung der wirksamkeit von Katalysatoren. Chem Ber 54: 638-647.
- Affrossman S, Thomson SJ (1962) Selective poisoning of the catalyst in the Rosenmund reaction. J Chem Soc PP: 2024-2029.
- Hershberg EB, Cason J (1941) β-Naphthaldehyde (2-Naphthaldehyde). Org Syn 21: 84-88.
- McEwen AB, Guttieri MJ, Maier WF, Laine RM, Shvo Y (1983) Metallic Palladium, the actual catalyst in Lindlar reductions. J Org Chem 48: 4436-4438.
- Boehm T, Schumann G, Hansen HH (1933) Untersuchungen in der kumarinreihe. Synthese einidreirung del saurechloridesche hyscheger kumarinaldehyde; mit Bemerkungen uber die katalytische hydrierung der saurechloride. Arch Pharm 271: 490-513.
- Smith DG, Smith DJH (1975) The preparation of aromatic aldehydes from acid chlorides. Chem Comm PP: 459-460.
- Scott AI, McCapra F, Buchanan RL, Day AC, Young DW (1965) Total synthesis of colchicine modelled on a biogenetic theory. Tetrahedron 21(12): 3605-3611.
- Chimichi S, Boccalini M, Cosimelli B (2002) A new convenient route to 2-oxoethoxycoumarins: key intermediate in the synthesis of natural products. Tetrahedron 58(24): 4851-4858.
- Chimichi S, Boccalini M, Cosimelli B, Viola G, Vedaldi D, et al. (2002) A convenient synthesis of psoralens. Tetrahedron 58(24): 4859-4863.
- Sardari S, Mori Y, Horita K, Micetich RG, Nishibe S, et al. (1999) Synthesis and antifungal activity of coumarins and angular furanocoumarins. Bioorg &Med Chem 7(9): 1933-1940.
- Yadav VG, Chandalia SB (1997) Synthesis of aldehydes by Rosenmund Reduction. Org Proc Res Dev 1: 226-232.
- Vogel AI (1978) Vogel’s textbook of quantitative inorganic analysis. 4th edition, Longman, pp: 474.
- Gaimpietro C, Gallo R, Galvango S, Spadaro A, Vitarelli P (1984) Polymer- Supportrd catalysts-selective hydrogenation of acid chlorides over palladium/polyamide. J Chem Technology and Biotechnology 34(8): 416-422.
- Chandnani KH, Chandalia SB (1999) Synthesis of m-Phenoxybenzaldehyde starting from chlorobenzenzene and m—cresol: some aspects of process development. Org Process Res Dev 3: 416-424.
- Britton H, Catterick D, Dwyer AN, Gordon AH, Leach SG, et al. (2012) Discovery and development of an efficient process to Atovaquone. Org Process Res Dev 16: 1607-1617.
- Hershberg EB, Cason J (1955) β-Naphthaldehyde. Org Syn Coll 3: 626-630.
- Danishefsky S, Hirama M, Gombatz HC, Harayama T, Berman E, et al. (1979) Total Synthesis of dl-pentalenolactone. J Am Chem Soc 100: 7020-7031.
- Peters JA, van Bekkum H (1981) The synthesis of aldehydes by means of a modified Rosenmund reduction of acid chlorides. Recl Trav Chim Pays-Bas 100(1): 21-24.
- Siegel S (2005) Encyclopedia of reagents I for organic Synthesis, John-Wiley & Sons, New Jersey, United States, pp: 3861-3865.