Influence of Donor and Acceptor Substituents in 1,3-Oxazole Derivatives and Their Anti-Cancer Activity
Nataliya V Obernikhina*
Department of Bioorganic and Biological Chemistry, O.O. Bogomolets National Medical University, Ukraine
2 Department of Chemistry GDC Kulgam, University of Kashmir, Jammu and Kashmir, India
Submission: August 24, 2019; Published: September 13, 2019
*Corresponding author: Nataliya V Obernikhina, O O Bogomolets National Medical University, Department of Bioorganic and Biological Chemistry, 13 T. Shevchenko boul., 01601, Kyiv, Ukraine
How to cite this article: Nataliya V Obernikhina. Influence of Donor and Acceptor Substituents in 1,3-Oxazole Derivatives and their Anti-Cancer Activity. Organic & Medicinal Chem IJ. 2019; 9(1): 555751. DOI: 10.19080/OMCIJ.2019.09.555751
Introduction
The heterocyclic compounds with branched conjugated systems including oxazole, thiazole or pirazole moieties have shown themselves as powerful scaffold in drug design, especially, highly active anticancer agents [1]. Consequently, the 1,3-oxazole skeleton could regard as applicable biologically active fragment. This molecular moiety can generate the enough “rigid” structure with the many biopolymers, what is considered as an important condition for increasing of selectivity to potential targets
Thus, 1,3-oxazole derivatives were studied as a potent inhibitor of inosine monophosphate dehydrogenase [2], as a N1-substituted imdazole-based peptide [3], as novel antitubulin agents [4]. But the anticancer properties of 1,3-oxazole derivatives were studied pointwise [5]. It is to be considered that biological activity or generation of the stable “rigid” structure with the receptors depends on the chemical constitution of target, including its possible electron structure. Therefore, 1,3-oxazole could be considered as perspective moiety in the design andfurther synthesis of novel biologically active agents that exert anticancer activity [1,6].
Then, we can assume that the π-electron system of the 1,3-oxazole with different in their electronic structure substituents should effective take part in the generation of the stable “rigid” complex with the receptors, transmembrane channels and enzymes. Chemically, introducing of donor or/and acceptor substituents in the oxazole cycle can considerably influence on the positions of its frontier levels and hence on the donor/acceptor properties of oxazole derivatives. Consequently, we could propose that such intentional change of molecular level energies may influence on the biological activity.
This paper presents the results of the computational study, firstly, quantum-chemical modeling of the new promising biologically active agent ligands, as well as comparing these results with the pharmacological testing of synthesized compounds with anti-cancer activity.
Materials and Methods
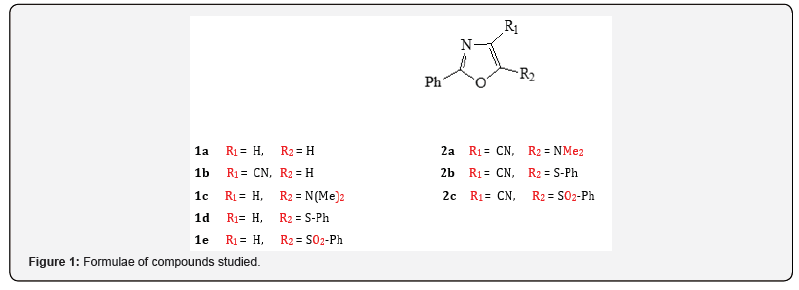
In present work the 1,3-oxazole derivatives were restricted by phenyl substituents in position 2 and variable donor/acceptor substituent in positions 4 and 5 so far as they have demonstrated the high selectivity on the biological activities; therefore, they are suitable for comparing of influence of the substituents on the molecules/biological properties. The molecules investigated can be presented by a general formula 1,2 pictured in Figure 1. Here, the molecules 1 are reference molecules, while the compounds 2 were synthesized and were screened of anticancer activity. The synthesis of compounds 2 was described earlier [7].
study of the qualitative structure-activity relationship (QSAR) of the some substituted 2-phenyl-oxazoles 1 were early performed [6]. The results of molecular docking have indicated the formation of the interaction between the conjugated system of the oxazole ligand and peptide fragment (with the distance at 3.5 Å) which stabilizers the protein-ligand complex. Consequently, the whole -electron system of the oxazole derivatives takes part in the origination of stable complex with receptor and hence influences on the first stage of the bioactivity process
Quantum-Chemical Calculations
The optimization of molecular geometry and calculation of charge distribution as well as the change frontier level positions were calculated by DFT/6-31 (d, p)/CAM-B3LYP method (package GAUSSIAN 03 [8]. In the quantum-chemical calculations, we have studied the reference molecules to estimate the influence of the variable donor/acceptor substituents. As the simplest reference molecule, the unsubstituted (R2 = R3 = H) oxazole was choose
Results and Discussion
To understand the connection between the chemical constitution of the derivatives of oxazole and their biological activity, particularly, as potential anticancer agents, the detailedstudy of the qualitative structure-activity relationship (QSAR) of the some substituted 2-phenyl-oxazoles 1 were early performed [6]. The results of molecular docking have indicated the formation of the interaction between the conjugated system of the oxazole ligand and peptide fragment (with the distance at 3.5 Å) which stabilizers the protein-ligand complex. Consequently, the whole -electron system of the oxazole derivatives takes part in the origination of stable complex with receptor and hence influences on the first stage of the bioactivity process
We could believe that electron structure, essentially, the donor/acceptor properties of the oxazole derivatives should undoubtedly influence on the stability of the generated complex agent-biopolymer. The it was interesting to fulfil the quantumchemical calculations of some model molecules in order to study the possible connection between the main electron characteristics of the molecules studied and their biological activity or, more correctly, stability of the complex. Mainly, it can propose that the electron levels and charge distribution of the central conjugated part of molecules 1,2 are connected with the stability of the former complex and hence the relative position of the frontier level (connecting experimentally with electron affinity and first ionization potential) gives the substantial contribution in total biological activity.
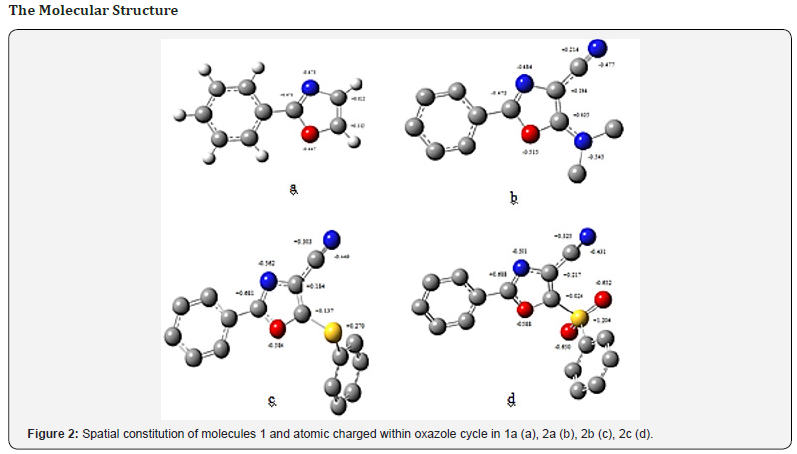
The optimized molecular geometry of some main model compounds is pictured in Figure 2. The simplest model unsubstituted molecule 1 (Figure 2a) is planar with the usual thickness of -system: 1.4 Å. The same thickness’ is for the conjugated cyano- substituent: -CN. The other substituents are out of the plane. Though, only the bulk methyl groups at the nitrogen atom in the amino derivatives 2a do not lie in the oxazole plane (Figure 2b). In contrast, both sulfur containing residues are situated so, that the phenyl group at the sulfur atoms do not conjugated with the oxazole cycle (Figures 2c & 2d). It is to bepointed that sulfur contains two lone electron pairs; one of them can conjugate with the -system of the oxazole. The introduced substituents can increase appreciably the effective space occupied by whole molecules 1,2. Besides, the phenyl group at the Sulphur atoms (2b and 2c) can rotate around single bond and hence can additionally increase the space of the formed complex.
Also, introducing of the substituents R1 and R2 influences appreciably on the atomic charges. We consider the charge distribution only in the oxazole cycle as an active center which should form the complex with the suitable target. The performed calculations have shown that the substituents R1 and R2 do not practically effect on the atomic charges within phenyl substituent in the position 2 of the oxazole cycle. The electron density is seen from Fig 2a to be distributed non-uniformly at the oxazole atoms because of considerable polarization of the heterodonts CN and CO.
Of course, the calculations give the substantial negative charges at the nitrogen and oxygen atoms. Two heteroatoms of higher electronegative causes simultaneously in the neighborhood positions the considerable deficit of electron density at the carbon atom in position 2 (+0.470), while the positive charges at carbon atoms in positions 4, 5 are essentially lesser. Contrary, introducing of CN group with formation of the non-polarized practically CC conjugated bond (Figure 2b) does not considerably effect on the charge distribution at the atoms within the oxazole cycle, except the carbon atom connected with the cyan substituent: its positive charge increase from +0.022 to +0.194. The considerable effect on the charge distribution is obtained upon introducing donor amino group in the position 5: Fig. 2b shows that the positive charge at the atom C-5 increases sharply because of two adjacent heteroatoms, O and N. The charges at rest atoms are lower sensitive to the such substituent, although the appreciable negative charge is located at the nitrogen atom in the donor substituent R2 = N(CH3)2.
Introducing of another donor substituent, R2 = S-Ph, in the same position 5 when oxazole cycle is bonded with the sulfur atom of the low electronegativity, influences comparativelyweakly effect on the atomic charges in the oxazole moiety, as one can see from Figure 2c. First, the sulfur atom bonded directly with oxazole cycle bears the no negative charge (in contrast to the donor dimethyl-amino group), but the positive charge +0.270. The positive charge at the carbon atom in the position 2 in the molecule 2b increases, in compare with the compound 2a. Because of the negligible difference in the electronegativities of the carbon and Sulphur atoms, the positive charge at the carbon atom in position 5 is considerably lower than in molecule 2a (compare the Figure 2c and 2b).
On the contrary, another sulfur-containing substituent, SO2N(CH3)2, in the molecules 1e and 2c should be considered as an acceptor. The oxygen atoms and phenyl residue do not conjugate with oxazole cycle, what is seen clearly from the spatial constitution (Figure 2d). As regard to the charge at the sulfur atom, its value is appreciably larger than in the donor substituent, R2 = SPh. The calculation gives also the considerable negative charges at both oxygen atoms bonded with the tetra-coordinated sulfur atom.
Thus, in spite of so different nature of the donor/acceptor substituent in the positions 5 of the compounds 1 and 2, the charge distribution in the oxazole cycle remains qualitative the same; then differences in biological activity should be connected with the other characteristics of the electron structure of the in the main oxazole cycle as an active biological center
Relative position of frontier levels and the donor/ acceptor properties
The positions of the highest occupied levels and the lowest vacant levels affect directly on the donor and acceptor ability of the compound studied, and hence to a biological activity. So, it was stated above that the oxazole derivatives exhibit the biological activity and could generate the complex with the biological polymers by the non-covalent interaction between ligand and polymer molecule. Then, we could assume the non-covalent interaction should depend on donor-acceptor properties of the probe molecules.

The calculated energies of some molecules 1 with different model donor and acceptors are collected in Table 1. The analysis of data in Table 1 shows unambiguously that the introduced substituents affect essentially on the positions of the molecular levels. So, going from the reference compound 1 with R2 = H, R3 = H to compounds with the acceptor residue CN or in position 4 is accompanied by the appreciable shift down of the highest occupied level, in the exact accordance with their electronic nature, while the shift of the lowest vacant levels is different, what is connected with the different change of the energy gap.
The more significant levels shifting is obtained upon introducing of both donor and acceptor substituents in the position 5. Simultaneously, the appreciable change of the energy gap (distance between the frontier levels) occurs. Therefore, it is difficult to estimate the donor or/and acceptor properties of any molecule using only one frontier level: lowest vacant or highest occupied; also, it is incorrect to compare the donor/acceptor ability basing on the energies of the separate levels. Apparently, it will be correct to estimate the donor/acceptor properties of any conjugated molecule basing on the relative position of the frontier levels.
It was proposed to estimate the donor/acceptor ability by special parameter 0 which calculated as the relative position of the frontier levels [9]. For neutral conjugated molecules, corresponds such dispositions of the frontier levels when the donor and acceptor properties are mutually balanced, for example, in long unsubstituted polyenes: then 0 = ½, i.e. the energy gap is disposed symmetrically in respect to the imaginary level .
The Calculation of the Comparatively Long Polyene, C12H24, Gives
LUMO = -1.296 eV; HOMO = -5.747eV and = -3.524 eV; consequently, 0 = 0.5. Then, the shifting of the energy gaps up and hence increasing of the parameter 0> 0.5 indicates on the predominately donor properties of the conjugated molecules. Whereas, if the parameter0< 0.5 and the energy is shifteddown, then the molecule is predominately acceptor.
Table 1 gives the values 0 calculated for the compounds studied. One can see that introducing of acceptor substituents in position 4 not only decreases the lowest occupied level, but also decreases the donor/acceptor parameter 0, relatively to the model molecule: compare data for 1a, R1 = H, R2 = H and 1b, R1 = CN, R2= H. Also, the decreasing of parameter0 and hence shifting down of the highest occupied levels occur upon introducing of acceptor group R2 = SO2NMe2 in the position 5.
On the contrast, introducing of donor substituent, NMe2, in position 5 (model molecule 1c, R1 = H, R2 = NMe2) causes shifting of the highest occupied and the lowest vacant levels up; therefore, the donor/acceptor parameter naturally increases, so that 0 > 0.5. Simultaneously introducing of donor and acceptor substituents in the adjacent positions (compound 2a, R1 = CN, R2 = NMe2) is accompanied by decreasing of the donor/acceptor parameter: 0 =0.53 (compare with 0 =0.49 for reference molecule). In other hand, it is logical that simultaneously introducing of two acceptor substituents in the positions 4 and 5 induces the substantial effect: parameter 0 decreases to 0.36 for the compound 2c with R1= CN, R2 = SO2NMe2. Parallelly, the calculations give the considerable shifting of both highest occupied and lowest vacant levels.
Comparing three 1,3-oxazole derivatives 2 with the same substituent CN in the position 4, we can state the index 0 decreases regularly in series: 2a, 2b and 2c. We could suppose that complex stabilities should increases in this series, what should influence on the biological activity.
In Vitro Evaluation of the Anticancer Activity
All the selected compounds were evaluated for their anticancer activity. Primary in vitro one dose anticancer assay was performed in full NCI 60 cell panel representing leukemia, non-small cell lung cancer, melanoma and cancers of colon, brain, breast, ovary and prostate in accordance with the protocol of the NCI, USA [7,10].
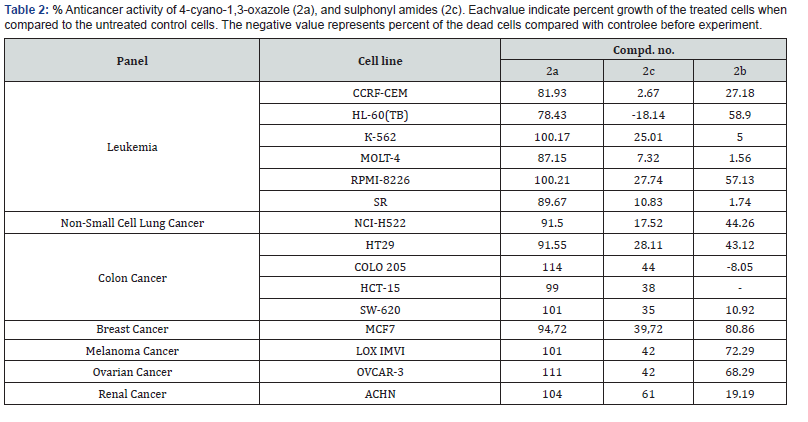
The compounds were added at a single concentration (10-5M). Results for each compound is presented as a Table 2 of the percent growth of the treated cells by compounds under investigation. Compound under investigation exhibited different anticancer activity against tested cell lines. It has been demonstrated that SO2-containing oxazole’s 1e and 2c exhibited better cytotoxic and cytostatic activity compared with its non SO2-analogs 2a. With regard to the effectivity against some individual cell lines (Table 2) the compounds 1e and 2c showed high activity against Leukemia К-562 (25 and 8 %), Leukemia SR (11 and 12%), Non-Small Cell Lung Cancer NCI-H522 (17,5 and -18%), and Colon Cancer SW- 620 (35 and 26% respectively) compared with its non-sulphonyl analog 2a exhibited worse cytotoxic activity (Leukemia К-562 (100 and 70 %), Leukemia SR (90 and 81%), Non-Small Cell Lung Cancer NCI-H522 (92 and 78%), and Colon Cancer SW-620 (101 and 97% respectively). Compounds 1e and 2c 6 showed moderate cytostatic activity against HL-60(TB) (-18,14%) and NCI-H522 (-18,31%) cells respectively. 2-Phenyl-1,3-oxazole-4-carboxylic acid methyl ester 2c containing SO2-group demonstrated high cytotoxic activity (Leukemia К-562 (3 and 5 %), Leukemia SR (3,70 and 1,74%), and Colon Cancer SW-620 (3 and 5%) and high cytostatic activity against COLO 205, ACHN and NCI-H522 cells (-63,88, -86,23 and -25,83%, compound 2c).
Table 2 % Anticancer activity of 4-cyano-1,3-oxazole (2a), and sulphonyl amides (2c). Eachvalue indicate percent growth of the treated cells when compared to the untreated control cells. The negative value represents percent of the dead cells compared with control before experiment
Conclusion
Thus, the analysis shows unambiguously that compounds 1-2 with the high acceptor substituents (-CN and -SO2R) (e.g. 2а) in the positions 4 and 5 have demonstrated the appreciable higher level of the effective inhibition of the cell growth whereas the oxazole derivatives (e.g. 2с) which contain the donor substituents (NHR or NH2) in position 5 do not practically effect on the inhibition process.
Acknowledgement
We would like to thank US Public Health Service and National Cancer Institute, USA, for in vitro evaluation of anticancer activity (providing the NCI-60 cell testing) within the framework of Developmental Therapeutic Program (http://dtp.cancer.gov), and Enamine Ltd for the material and technical support.
Disclaimer
This material should not be interpreted as representing the viewpoint of the National Institute of Allergy and Infectious Diseases (USA) and its Collaborative Antiviral Testing Group
References
- Havrylyuk D, Zimenkovsky B, Vasylenko O, Craig WD, Smee DF, et al. (2013) Synthesis of pyrazoline-thiazolidinone hybrids with trypanocidal activity. Eur J Med Chem 66:228.
- Turchi IJ, Dewar MJS (1975) Chemistry of oxazoles. Chem Rev 75(4): 389.
- Turchi IJ (1981) Oxazole chemistry. A review of recent advances. Id Eng Chem prod Res Dav 20:32.
- Brovarets V, Zyabrev V (2014) (Eds) Lambert Academic Publishing. Saarbruken, Doutshland, 449.
- Kornienko AN, Pil o SG, Kozachenko AP, Prokopenko VM, Rusanov EB, Brovarets VS (2014) Chem Het Comp 50(1): 76.
- Kovalishyn V, Kopernyk I, Chumachenko S, Shablykin O, Kondratyuk K, et al. (2014) QSAR studies, design, synthesis and antimicrobial evaluation of azole derivatives. Comput Biology and Bioinf 2(2):25.
- Kachaeva MV, Obernikhina NV, Veligina ES, Zhuravlova MYu, Prostota YaO, et al. (2019) Chem Het Comp 55(4-5): 448.
- Frisch MJ, Trucks GW, Schlegel HB, et al. (2003) A potential energy profile of the catalytic cycle of pyruvate decarboxylase. Gaussian, Inc., Wallingford CT.
- Obernikhina N, Kachaeva M, Shchodryi V, Prostota Y, Kachkovsky O, et al. (2019) Polycyc Arom Comp 39(1):1.
- Kachaeva M, Hodyna D, Obernikhina N, Pilyo S, Kovalenko Yi, et al. (2019) J Het Cem in press.