Evaluation of Chronic Oral Nicotine Treatment in Food Consumption, Body Weight and [125I] Epibatidine Binding in Adult Mice
Pei-San Huang1, Louise C. Abbott1 and Ursula H Winzer-Serhan2*
1Department of Veterinary Integrative Biosciences, Texas A&M University, USA
2Department of Neuroscience and Experimental Therapeutics, Texas A&M University, USA
Submission: October 17, 2015; Published: November 25, 2015
*Corresponding author: Dr. Ursula H Winzer-Serhan, Department of Neuroscience and Experimental Therapeutics, Medical Research & Education Bldg, 8447 State Highway 47MS 1359, Bryan, TX 77807-3260, Tel: 1 979 436-0330; Email: uwserhan@tamhsc.edu
How to cite this article:Pei-San H, C. Abbott L, Winzer-Serhan HU. Evaluation of Chronic Oral Nicotine Treatment in Food Consumption, Body Weight and [125I] Epibatidine Binding in Adult Mice. Open Access J of Tox. 2015; 1(1): 555552. DOI: 10.19080/OAJT.2015.01.555552
Abstract
Despite its abuse potential, nicotine, acting on nicotinic acetylcholine receptor, has possible medicinal uses, in particular in treating neuro degenerative diseases. Therefore, animal models to evaluate exposure need to be characterized. Administration via drinking water is a stressfree route of administration but often results in low blood nicotine levels. Here we evaluated chronic exposure to low, medium and high concentrations of nicotine in drinking water. Three-month-old C57BL/6 male mice were treated for 23 days with 20, 120 or 300 ^g/ml nicotine in 2% saccharin water, corresponding to 5, 30 and 55 mg/kg/d, respectively. Food intake and body weight were monitored, blood nicotine and cotinine levels, and 125I-epibatidine-binding sites were determined at day 23.Average blood cotinine levels of 11.7, 151.8 and 192.0 ng/ ml were detected in mice receiving the low, medium and high dose, respectively. In contrast, nicotine was only consistently measured in the group receiving 300 μg/ml, with an average blood level of 6.2 ng/ml and was the only treatment group to exhibit significantly decreased food intake (p=0.005) and body weight (p=0.043), as well as increased I125-epibatidine binding in cortex (p=0.055) and hippocampus (p = 0.019). We evaluated possible effects of chronic nicotine (300^g/ml) exposure on anxiety-like behavior using the open field test. An anxiolytic effect was found compared to controls and there was no evidence for anxiogenic effects of chronic nicotine. Thus, a high concentration of nicotine in drinking water was necessary to achieve consistent blood nicotine levels in mice, which correlated with markers considered hallmarks of chronic nicotine treatment.
Keywords: Nicotine; nAChR; Hippocampus; Receptor Binding; Body Weight; Food Intake; Anxiety
Abbreviations: AD: Alzheimer's Disease; ANOVA: Analysis of Variance; DG: Dentate Gyrus; nAChR: Nicotinic Acetylcholine Receptor; ROD: Raw Optical Density.
Introduction
The plant alkaloid nicotine interacts with neuronal nicotinic acetylcholine receptors (nAChRs), which are pentameric ligand gated cation channels that are either homomerically or heteromerically formed by different subunit combinations. Among the possible subunit combinations, heteromeric α4β2 nAChRs are the most widely expressed heteromeric nAChR subtype in the mammalian brain and exhibit high affinity for nicotine [1,2]. Nicotine, the major psychoactive ingredient in tobacco smoke [3], facilitates dopamine release and activates the reward pathway [4,5], and as such is considered an addictive drug, and strongly implicated in tobacco dependence [6,7]. However, nicotine may also have beneficial effects; the drug has shown cognitive enhancing, anxiolytic and neuro protective properties in animals and humans [8-10], may slow age-related neuronal decline, and reduce neuronal degeneration in Alzheimer's or Parkinson's disease [11,12]. Neuro protective effects of nicotine are the result of nAChR activation; in particular, activation of heteromeric nAChRs seems to be important. Transgenic mice that lack the β2 subunit (β2 nAChR knockout (KO) mice) and thus do not express high affinity nAChRs, have reduced adult neurogenesis [13] and exhibit accelerated aging in cortical areas, underscoring the importance of heteromeric nAChRs to maintain a healthy mature brain [14,15]. Thus, medicinal use of nicotine or related nAChR agonists could have great beneficial effects for human health. Therefore, despite the known potential for abuse, nicotine and nicotinic receptor agonists are being evaluated for therapeutic uses [16-18]. Medicinal use of nicotine would often require long-term drug administration. To study effects of nicotine administration in adult animal models over several weeks or month, the route of nicotine administration becomes very important. Voluntary oral consumption of nicotine via drinking water is non-invasive, stress-free and requires no additional animal handling. Therefore, this route of drug is suitable for long-term treatment in preclinical animal models, and is a viable alternative to daily injections or use of osmotic mini pumps. In addition, administration via drinking water results in nicotine fluctuations during a 24-hour period, which allows time for re-sensitization of desensitized nAChRs. On the other hand, oral nicotine administration results in poor bioavailability, which means that the fraction of administered nicotine reaching systemic circulation is lower compared to other routes, and results in low and more variable levels of nicotine in the brain. This is due to ion trapping of nicotine in the acidic milieu in the stomach [19], slow absorption through the gastrointestinal tract, and rapid first pass metabolism in the liver [20,21]. In mice in particular, nicotine is rapidly metabolized, and strain specific metabolic rates for nicotine may differ [22,23].On the other hand, nicotine can be absorbed directly through the buccal cavity where first pass metabolism is not a factor [19]. Together, these properties make it difficult to estimate effective doses of orally administered nicotine based solely on dose [24,25]. Two hallmarks of chronic nicotine exposure have been described for decades: an anorexic effect that results in decreased food consumption and body weight [26-29], and an increase in high affinity nAChR binding sites [30-33]. Together with determining blood nicotine and cotinine levels, we used these two hallmarks to evaluate central effects of chronic oral nicotine administration in adult C57BL/6 mice. In addition, there are reports that chronic nicotine exposure affects anxiety in humans and in animal models [34-38]. Therefore, we also evaluated the effects of chronic nicotine administration on anxiety-like behavior.
Materials and Methods
Animals and Drug Administration
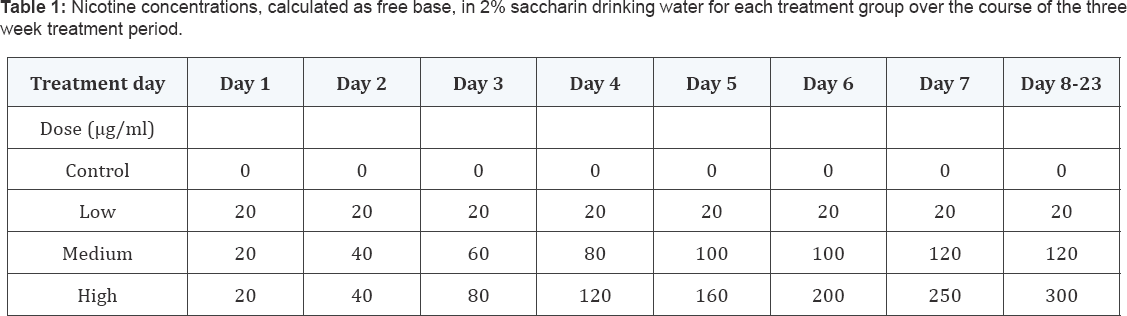
Young adult male C57BL/6 mice were obtained from a breeding colony established at Texas A&M University's Laboratory Animal Research and Resource facility from mice originally obtained from the Jackson Laboratory, Bar Harbor, ME. At three months of age, mice were individually caged and randomly assigned to different dosing groups (n = 5). Then all mice received 2% saccharin (saccharin sodium salt hydrate, >98%) (Sigma, St. Louis, MO, USA) in their drinking water starting two weeks prior to actual dosing with nicotine to establish their individual daily drinking volume. (-) -Nicotine (hydrogen tartrate) (Sigma) was administered in 2% saccharin containing water. Four nicotine concentrations (given as free base) were tested: control - 0 μg/ml (0 mg/kg/day), low dose(LD) - 20 μg/ ml, medium dose (MD)- 120 μg/ml and high dose (HD)- 300 μg/ml resulting in approximately 5, 30 and 55 mg/kg/day free base nicotine, respectively, calculated based on average liquid consumption and body weight. Animals in the MD-and HD- groups initially received 20 μg/ml of nicotine on the first day of treatment and the concentrations were gradually increased until the final concentrations were reached on day 7 and day 8 of treatment, respectively (Table 1). Individual water consumption was not significantly affected until a concentration of 200 μg/ ml was reached; at 300 μg/ml nicotine, water intake was 86.1% (77.7 - 98.08 %) of the initial individual consumption levels (p=0.018). All mice were treated until day 23. Access to food and drinking solutions was ad libitum. Food intake and body weight were assessed every three days, starting from day 1 to day 19, and normalized to the first measurement of each individual animal. On days 20 to 22, the mice were subjected to behavioral testing, and then euthanized on day 23. All procedures were approved by the Texas A&M University Animal Use Committee and carried out in accordance with the National Institutes of Health Guide for the Care and Use of Laboratory Animals (National Institutes of Health Publication No. 85-23, revised 1996).
Open Field Test
To test locomotor, exploratory and anxiety behavior, on day 20 of nicotine treatment, mice were placed individually in an open field (length x width x height: 20 x 20 x 30 cm) (Versa max animal activity monitor and analyzer, AccuScan Instruments, Columbus, OH, USA) equipped with vertical and horizontal infrared beam sensors. The apparatus was constructed using transparent Plexiglas, located in a quiet room, and environmental cues including lighting and experimenter were kept constant during testing. Mice were acclimated to the experimental room for 5 minutes, and then placed in the open field for 30 minutes. Activitywas recorded every minute. The open field chamber was cleaned with 50% ethanol between mice. Horizontal activity, total distance, movement time, rest time, stereotype counts, stereotype time, margin distance, margin time, center distance, center time, rearing activity, and rearing time were automatically recorded.
Tissue preparation
All mice were anesthetized using isoflurane (IsoFlo, North Chicago, IL, USA) and decapitated on day 23 in the morning. Approximately 1 ml of trunk blood was collected from each mouse, kept on ice, and centrifuged at 4°C at 3000 rpm for 20 minutes. One hundred to 300 μl of serum was collected from each mouse and sent to Bio analytical Core Laboratory Service Center (Department of Pharmaceutics, School of Pharmacy, Virginia Commonwealth University, Richmond, VA, USA) for analysis of nicotine and cotinine levels. The brains were rapidly dissected, frozen on powdered dry ice, and stored at -80°C.
[125I]-Epibatidine Binding
Radioactive, iodinated [125I]-epibatidine ligand was used to evaluate the relative levels of heteromeric nAChR binding sites. Receptor autoradiography was performed as previously described [39]; briefly, tissue sections were warmed to room temperature (RT) and pre-incubated 5 minutes in Tris-HCl buffer (50 mMTris-HCl, 120 mMNaCl, 5 mMKCl, 2.5 mM CaCl2, 1 mM MgCl2, pH 7.4). Then sections were incubated with 0.4 nM [125I]-epibatidine (PerkinElmer Life Science NEX358, Boston, MA, USA, specific activity: 2200 Ci/mmol) for 1 hour at RT. For non-specific binding, sections were incubated with the presence of 400 μM (-)-nicotine hydrogen tartrate. Sections were washed in ice-cold Tris-HCl buffer twice for 1 minute each, followed by 10 seconds in cold ddH2O, and dried under air stream for 1 hour Sections were dried at RT over night before exposure to BioMax MR Film (Kodak, Rochester, NY, USA). After 1-day of exposure, films were developed in D19 Kodak developer for 4 minutes, rinsed in water, fixed in Kodak Rapid Fixer for 5 minutes, and air-dried.
Data Analyses
Quantitative analysis of auto radiograms was done using a PC-based image analysis system, MCID basic (InterFocus Imaging Ltd, Haverhill, Suffolk, UK). Receptor binding levels were measured as raw optical density (ROD) in the hippocampus (combined measures in the molecular layer of the dentate gyrus (DG) and the stratum lacunosum molecular of the CA1 in hippocampus proper), thalamus (the post thalamic nucleus and the ventral posteriomedial thalamic nucleus), and cerebral cortex, specifically layers IV-V of the visual and somato sensory cortex. All anatomical structures were determined according to the Mouse Brain Library atlas. SPSS 14.0 was used for statistical analysis. Significance was defined as p ≤ 0.05. For food intake and body weight changes, repeated measurements were used for between-subject effects after nicotine reached the highest dose in all groups (day 8), and Student's t-tests were used to determine significant differences at each time point between nicotine and control groups. For receptor binding, one-way analysis of variance (ANOVA) was used and Tukey HSD was performed as a post hoc test when needed.For open field test, Student's t-test was used to compare nicotine and control groups. All data are presented as average ± standard error the mean (SEM).
Results
Blood Nicotine and Cotinine Levels

Nicotine or cotinine were not detected in the serum of control mice (< 2 ng/ml and < 1 ng/ml, respectively).In the LD-and MD- group,4 out of 5 mice had undetectable levels of nicotine (< 2 ng/ml) in blood serum, with one mouse in the LD group with 2.9 ng/ml and one mouse in the MD group with 19.7 ng/ml nicotine. Cotinine levels ranged from of 3.2 to 25.0 ng/ml with an average of 11.7 ng/ml in the LD group (20 μg/ ml), and from 2.0 to 586.9 ng/ml with average of 151.8 ng/ml in the MD group (120 μg/ml) (Table 2). In the HD group (300 μg/ml) four out of five mice had detectable blood nicotine levels ranging from 2.1 to 21.1 ng/ml with an average of 6.2ng/ml and cotinine concentrations had a range of 41.4 to 578.1 ng/ ml and an average of 192 ng/ml. There were no differences in food intake and body weight between mice in the control, LD- and MD-groups (food intake: repeated measurements, between- subject effect, p = 0.52; body weight: repeated measurements, between-subject effect, p=0.88). In addition, numbers of nACh Rreceptor binding sites detected with receptor autoradiography using 125I-epibatidine binding, showed no significant difference between brain sections derived from mice in the control versus LD-or MD-groups in cortex, hippocampus or thalamus (one-way ANOVA; cortex:p = 0.63; hippocampus: p = 0.56; thalamus: p =0.45). Thus, low and medium doses of nicotine administered via drinking water, did not result in consistent blood nicotine levels and did not affect food intake, body weight or nicotinic receptor binding (Table 2). Only the highest dose of nicotine tested in this study resulted in significant differences in these measures, which we will describe in more detail.
Food Intake and Body Weight
Food intake at the first measurement time point was similar in control- and HD-groups (control: 3.61 ± 0.14 g/day; HD- group: 3.75 ± 0.07 g/day). Subsequent measurements were normalized to the first measurement for each individual mouse, and comparisons were made between groups starting from day 8, when the high dose of 300μg/ml nicotine in the drinking water was reached (Table 1). After day 8, the average daily food intake was significantly lower in mice in the HD-group compared to control-group (control: 4.30 ± 0.26 g/day; HD-group: 3.79 ± 0.13 g/day; repeated measurements, between-subject effect, p=0.005) (Figure 1A). Overall, mice in the HD-group decreased their food intake by about 12% compared to control mice. Body weights on the first day of treatment were identical between groups (control: 26.59± 1.00 g; HD-group: 26.39 ± 0.70 g), and subsequent measurements were normalized to the initial weight of each individual animal. Body weights in control mice increased continuously, over the treatment period and mice gained, on average, 0.16 ± 0.04 g/day (Figure 1B). In contrast, after day 8, when the full-dose of nicotine was reached in the HD-group, mice exhibited a slight loss in body weight of 0.02 ± 0.07 g/day. This resulted in increased body weight in control mice but not in mice from the HD-group on day 19 compared to their individual weight on day 1 (Student's t-test, control: p=0.02; nicotine: p=0.814), and subsequently a 4% decrease in body weight between treatment groups on day 19 (control: 27.59 ± 0.83 g; HD-group: 26.39 ± 0.51 g; body weight, repeated measurements, between-subject effect, p = 0.043).

Nicotinic receptor autoradiography
Nicotinic receptor autoradiography using 125I-epibatidine, was performed to evaluate nicotinic receptor binding sites after chronic treatment to validate nicotine exposure in the brain [40]. Non-specific binding was negligible, and the specific binding pattern was similar to those previously reported with strong binding in the thalamus, medial habenula, and fasciculus retroflexus, moderate binding in cortex and low levels of binding in the hippocampus [41,42] (Figure 2A). In the hippocampus, relative 125I-epibatidine binding, measured in stratum lacunosum molecular of the CA1 and in the molecular layer of the dentate gyrus, was significantly higher in brain slices from mice in the HD-group compared to control mice (ROD control: 0.39 ± 0.010; nicotine: 0.42 ± 0.003; Student's t-test, p = 0.019) (Figure 2B). In the cortex, relative 125I-epibatidine binding level was slightly higher in mice from the HD-group compared to control mice (control: 0.48 ± 0.011; nicotine: 0.50 ± 0.007; Student's t-test, p = 0.055) (Figure 2B). In contrast, in the thalamus, relative 125I-epibatidine binding was not affected by high dose nicotine treatment (control: 0.70 ± 0.024; HD-group: 0.68 ± 0.011, p = 0.435) (Figure 2B).

Effects on anxiety like behavior
Nicotine, when used for medicinal purposes, should not have anxiogenic effects. Therefore, we used the open field test to evaluate anxiety-like behavior in mice from control- and HD- group. On day 20 of treatment, locomotor activity, exploratory- and anxiety-like behaviors were assessed in the open field test. Of the 12 parameters measured (see material and method section), 10 parameters showed no significant differences (Student’s t-test, p >0.05) indicating that locomotor activity and exploratory behaviors were neither impaired nor augmented in the HD-group compared to control. Only margin and center time, two parameters that indicate anxiety levels, were significantly affected. Control mice spent 78% of the time at the margins, significantly longer than mice from the HD-group, which spent only 64% of the time at the margins (30 minutes overall, Student’s t-test, p = 0.025) (Figure 3A). When activity was analyzed in 10 minute intervals, control mice spent significantly more time in the margin area compared to mice from the HD-group during the first and second 10 minute intervals (first interval: control: 425 ± 17 sec, HD-group: 352 ± 24 sec, Student’s t-test, p = 0.037; second interval: control: 496 ± 19 sec, HD-group: 392 ± 35 sec, Student’s t-test, p = 0.031). Group differences diminished during the last ten minutes when mice in the HD-group increased the time spent at the chamber’s margins (control: 475 ± 36 sec; HD- group: 411 ± 20 sec) (Figure 3A). These results suggested that chronic nicotine had no anxiogenic effects, but to further assess this possibility, we calculated the thigmotaxis index (margin distance / total distance), which has been suggested to bean index of anxiety in mice [43]. The thigmotaxis index was slightly higher in control mice compared to mice from the HD-group, with significant differences during the second 10 minute interval (control: 0.71 ± 0.04; HD-group: 0.59 ± 0.03; Student’s t-test, p = 0.021) (Figure 3B), which also suggested reduced anxiety in mice treated with the high dose of nicotine.

Discussion
The goal of this study was to determine an oral dose of nicotine that is well tolerated by mice, and induces central effects typically associated with chronic nicotine. In this study we show that voluntary oral consumption of nicotine-containing drinking water can result insignificant decreases in food intake and subsequently body weight, and increased nicotinic receptor binding, measures which are considered hallmarks of chronic nicotine exposure. However, these effects were only seen with the highest dose of nicotine (300 μg/ml) administered in the drinking water, which was also the only dose that resulted consistently in measurable blood nicotine levels in the adult male mice used in this study. We used three different concentrations of nicotine in the drinking water (20, 120 and 300 μg/ml) and measured the resulting blood nicotine and cotinine levels after three weeks of exposure. All three doses were well tolerated by the mice with no obvious negative effects. The low and medium doses (20 and 120 μg/ml) resulted in undetectable blood nicotine concentrations in 4 out of 5 mice. Only mice treated with the highest dose had measurable blood nicotine levels in most animals (4 out of 5 mice) with an average concentration of 6.2 ng/ml. Blood nicotine concentrations of up to 21.1 ng/ ml were measured, which are comparable to concentrations seen in people using nicotine replacement products, smoking a cigarette or using smokeless tobacco products such as chewing tobacco or snuff [19]. However, variability in the HD groups was high because of the rapid degradation of nicotine in mice. With increasing nicotine concentrations in the drinking water, we saw increases in blood cotinine levels, the major metabolite of nicotine. However, at all three doses, blood cotinine levels exhibited high variability, which seems to be characteristic for the oral administration route. At 300μg/ml, blood cotinine concentrations ranged from 41.4-578.1 ng/ml with an average of 192 ng/ml. These levels are comparable to findings from other groups, which have shown that in mice treated with nicotine via drinking water (200 to 500 μg/ml), plasma cotinine levels from 652 to 1450 ng/ml were found [44,45]. The large variability of plasma cotinine levels between and within studies is likely due to the relatively short half-life of cotinine in mice (20-40 minutes) compared to other species (rats 5-6 hours and human 19 hours) [24,25,46,47]. Thus, blood cotinine concentration may not be a reliable surrogate measure of blood nicotine levels in mice, but does indicate recent nicotine consumption. Over the years, a number of studies have shown that chronic nicotine reduces body weight and/or suppresses food intake in humans and laboratory animals [48-52]. In neonates, even very low doses of nicotine reduces body weight gain [53], and people using nicotine replacement therapy during smoking cessation, also exhibit reduced weight gain [54,55]. In this study, an anorexic effect of nicotine was only observed in the HD-group, whereas in the LD- group and MD-group, there was no effect on either food intake or body weight. This is consistent with other studies which used the oral route of nicotine administration and reported that chronic treatment with lower nicotine concentrations via drinking water does not affect weight gain or food intake [56]. Furthermore, nicotine's anorexic effect did not start until treatment day 8, when the highest dose of nicotine was reached. This result correlated with more consistently measurable blood nicotine levels in the HD-group, and suggests that nicotine consumed through the drinking water can exert central anorexic effects. However, decreases in body weight and food intake have not consistently been reported with chronic oral nicotine administration [57], or were only seen after prolonged treatment [45]. It is possible that sufficient blood nicotine levels where not reached for long enough time periods to trigger an anorexic effect [57], or where only reached after the highest dose was administered [45]. Another hallmark for the central effects of chronic nicotine exposure is an increase in heteromeric high affinity nicotinic binding sites in the brain, which have also been observed in oral nicotine administration models using mice [33,57,58]. Using receptor autoradiography, we determined that only the highest treatment dose of nicotine increased 125I-epibatidine binding to heteromeric nAChRs in cortical and hippocampal regions. In contrast, low and medium doses had no effect, although, cotinine measurements indicated recent consumption of nicotine. The receptor binding results correlate well with the lack of consistently detectable blood nicotine concentrations and lack of an anorexic effect in the LD- and MD-groups, and suggest that nicotine has to be chronically present at sufficient levels in order to up regulate nicotinic binding sites. Taken together, nicotine and cotinine blood levels, food intake, body weight, and receptor binding results indicate that nicotine consumed via the drinking solution, is effective in reaching relevant levels of nicotine in the mouse brain comparable to those seen in human smokers or patients using nicotine replacement medications. However, due to the rapid metabolism of nicotine in mice, relatively high levels of nicotine doses need to be consumed to achieve levels that reliably induce central effects. Chronic exposure to nicotine can affect anxiety levels in humans and animals, but the issue is complex. Most human smokers experience lower anxiety levels than non-smokers when challenged with stress, and increased anxiety levels during smoking cessation, suggesting that chronic nicotine exposure can reduce anxiety in humans [34-38]. However, in rodents, nicotine’s effects on anxiety are less consistent. Chronic or acute nicotine administration can result in either an anxiolytic or anxiogenic response, depending on several confounding factors such as age, dose, route of administration, sex and species [37,38]. Nicotine or other nAChR agonists given chronically for medicinal purpose should not induce anxiety. Very few studies have evaluated the effects of chronic nicotine exposure administered via drinking water on behavioral measures. In one such study, female but not male mice exhibited increased anxiety-like behavior after chronic oral nicotine administration [59], whereas a sex independent anxiogenic effect was found in wild type mice in another study [60]. Therefore, we evaluated anxiety-like behavior in the HD- group using the open field test. In this study, locomotor and exploratory activities were not changed, but two parameters, margin time and thigmotaxic index, were lower in mice from the HD-group compared to controls, indicating a trend towards reduced anxiety levels. This indicates that, chronic oral nicotine treatment with a dose sufficient to up regulate heteromeric nAChR binding sites and to affect body weight, did not increase anxiety-like behavior in adult male mice, but might have mild anxiolytic effects. These findings are consistent with results found in adult male mice after chronic oral administration of nicotine, which also did not result in increased anxiety-like behavior [59], but contrast with results from a study which used a lower dose of nicotine [60]. The reasons for the discrepancies between these studies are not clear.
Conclusion
Oral self-administration of nicotine via drinking water provides a stress-free route of chronic drug treatment, but requires relatively high doses to reach biological active levels of nicotine in the mouse brain. Three weeks of nicotine consumption decreased food intake and body weight, and increased expression of high affinity nicotine binding sites, hallmarks of central effects of chronic exposure to nicotine. Furthermore, chronic voluntary oral consumption of nicotine at levels high enough to exhibit central effects of chronic nicotine exposure,and did not have anxiogenic effects, making this route and this dose suitable for long-term treatment of adult mice.
Conflict of Interest
The authors declare that there are no conflicts of interest.
Acknowledgement
The open access publishing fees for this article have been covered by the Texas A&M University Online Access to Knowledge (OAK) Fund, supported by the University Libraries and the Office of the Vice President for Research.
References
- Cooper E, Couturier S, Ballivet M (1991) Pentameric structure and subunit stoichiometry of a neuronal nicotinic acetylcholine receptor. Nature 350(6315): 235-238.
- Sargent PB (1993) The diversity of neuronal nicotinic acetylcholine receptors. Annu Rev Neurosci 16: 403-443.
- Abbott LC, Winzer-Serhan UH (2012) Smoking during pregnancy: lessons learned from epidemiological studies and experimental studies using animal models. Crit Rev Toxicol 42(4): 279-303.
- Kleijn J, Folgering JH, van der Hart MC, Rollema H, Cremers TI, et al. (2011) Direct effect of nicotine on mesolimbic dopamine release in rat nucleus accumbens shell. Neurosci Lett 493(1-2): 55-58.
- Zhang L, Dong Y, Doyon WM, Dani JA (2012) Withdrawal from chronic nicotine exposure alters dopamine signaling dynamics in the nucleus accumbens. Biol Psychiatry 71(3): 184-191.
- Benowitz NL (2010) Nicotine addiction. N Engl J Med 362(24): 2295-2303.
- Dani JA, Balfour DJ (2011) Historical and current perspective on tobacco use and nicotine addiction. Trends Neurosci 34(7): 383-392.
- Picciotto MR, Zoli M (2008) Neuroprotection via nAChRs: the role of nAChRs in neurodegenerative disorders such as Alzheimer’s and Parkinson’s disease. Front Biosci 13: 492-504.
- White HK, Levin ED (2004) Chronic transdermal nicotine patch treatment effects on cognitive performance in age-associated memory impairment. Psychopharmacology 171(4): 465-471.
- Mudo G, Belluardo N, Fuxe K (2007) Nicotinic receptor agonists as neuroprotective/neurotrophic drugs. Progress in molecular mechanisms. J Neural Transm (vienna) 114(1): 135-147.
- O'Neill AB, Morgan SJ, Brioni JD (1998) Histological and behavioral protection by (-)-nicotine against quinolinic acid-induced neurodegeneration in the hippocampus. Neurobiol Learn Mem 69(1): 46-64.
- Socci DJ, Arendash GW (1996) Chronic nicotine treatment prevents neuronal loss in neocortex resulting from nucleus basalis lesions in young adult and aged rats. Mol Chem Neuropathol 27(3): 285-305.
- Harrist A, Beech RD, King SL, Zanardi A, Cleary MA, et al. (2004) Alteration of hippocampal cell proliferation in mice lacking the beta 2 subunit of the neuronal nicotinic acetylcholine receptor Synapse 54(4): 200-206.
- Huang PS, Son JH, Abbott LC, Winzer-Serhan UH (2011) Regulated expression of neuronal SIRT1 and related genes by aging and neuronal beta2-containing nicotinic cholinergic receptors. Neuroscience 196: 189-202.
- Zoli M, Picciotto MR, Ferrari R, Cocchi D, Changeux JP (1999) Increased neurodegeneration during ageing in mice lacking high- affinity nicotine receptors. EMBO J 18(5): 1235-1244.
- Bencherif M, Hauser TA, Jordan KG, Gatto GJ (2006) Therapeutic potential of novel selective drugs targeting nicotinic acetylcholine receptors. J Mol Neurosci 30(1-2): 17-18.
- Hurst R, Rollema H, Bertrand D (2013) Nicotinic acetylcholine receptors: from basic science to therapeutics. Pharmacol Ther 137(1): 22-54.
- Levin ED, Rezvani AH (2002) Nicotinic treatment for cognitive dysfunction. Curr Drug Targets CNS Neurol Disord 1(4): 423-431.
- Hukkanen J, Jacob P 3rd, Benowitz NL (2005) Metabolism and disposition kinetics of nicotine. Pharmacol Rev 57(1): 79-115.
- Le Houezec J, Martin C, Cohen C, Molimard R (1989) Failure of behavioral dependence induction and oral nicotine bioavailability in rats. Physiol Behav 45(1): 103-108.
- Matta SG, Balfour DJ, Benowitz NL, Boyd RT, Buccafusco JJ, et al. (2007) Guidelines on nicotine dose selection for in vivo research. Psychopharmacology (Berl) 190(3): 269-319.
- Alsharari SD, Siu EC, Tyndale RF, Damaj MI (2014) Pharmacokinetic and pharmacodynamics studies of nicotine after oral administration in mice: effects of methoxsalen, a CYP2A5/6 inhibitor. Nicotine Tob Res 16(1): 18-25.
- Siu EC, Tyndale RF (2007) Characterization and comparison of nicotine and cotinine metabolism in vitro and in vivo in DBA/2 and C57BL/6 mice. Mol Pharmacol 71(3): 826-834.
- Miller RP, Rotenberg KS, Adir J (1977) Effect of dose on the pharmacokinetics of intravenous nicotine in the rat. Drug Metab Dispos 5(5): 436-443.
- Pekonen K, Karlsson C, Laakso I, Ahtee L (1993) Plasma nicotine and cotinine concentrations in mice after chronic oral nicotine administration and challenge doses. European Journal of Pharmaceutical Sciences 1(1): 13-18.
- Bellinger LL, Wellman PJ, Cepeda-Benito A, Kramer PR, Guan G, et al. (2003) Meal patterns in male rats during and after intermittent nicotine administration. Pharmacol Biochem Behav 80(3): 437-444.
- Hajek P, Jackson P, Belcher M (1988) Long-term use of nicotine chewing gum. Occurrence, determinants, and effect on weight gain. JAMA 260(11): 1593-1596.
- Wager-Srdar SA, Levine AS, Morley JE, Hoidal JR, Niewoehner DE (1984) Effects of cigarette smoke and nicotine on feeding and energy. Physiol Behav 32(3): 389-395.
- Jo YH, Talmage DA, Role LW (2002) Nicotinic receptor-mediated effects on appetite and food intake. J Neurobiol 53(4): 618-632.
- Marks MJ, Burch JB, Collins AC (1983) Effects of chronic nicotine infusion on tolerance development and nicotinic receptors. J Pharmacol Exp Ther 226(3): 817-825.
- Perry DC, Davila-Garcia MI, Stockmeier CA, Kellar KJ (1999) Increased nicotinic receptors in brains from smokers: membrane binding and autoradiography studies. J Pharmacol Exp Ther 289(3): 1545-1552.
- Collins AC, Bhat RV, Pauly JR, Marks MJ (1990) Modulation of nicotine receptors by chronic exposure to nicotinic agonists and antagonists. Ciba Found Symp 152: 68-82.
- Andreasen JT, Nielsen EO, Redrobe JP (2009) Chronic oral nicotine increases brain [3H]epibatidine binding and responsiveness to antidepressant drugs, but not nicotine, in the mouse forced swim test. Psychopharmacology (Berl) 205(3): 517-528.
- Gilbert DG, Robinson JH, Chamberlin CL, Spielberger CD (1989) Effects of smoking/nicotine on anxiety, heart rate, and lateralization of EEG during a stressful movie. Psychophysiology 26(3): 311-320.
- Perkins KA, Grobe JE (1992) Increased desire to smoke during acute stress. Br J Addict 87(7): 1037-1040.
- Tate JC, Pomerleau OF, Pomerleau CS (1993) Temporal stability and within-subject consistency of nicotine withdrawal symptoms. J Subst Abuse 5(4): 355-363.
- Picciotto MR, Brunzell DH, Caldarone BJ (2002) Effect of nicotine and nicotinic receptors on anxiety and depression. Neuroreport 13(9): 1097-1106.
- Picciotto MR, Lewis AS, van Schalkwyk GI, Mineur YS (2015) Mood and anxiety regulation by nicotinic acetylcholine Receptors: A potential pathway to modulate aggression and related behavioral states. Neuropharmacology 96(Pt B): 235-243.
- Huang LZ, Winzer-Serhan UH (2006b) Effects of paraformaldehyde fixation on nicotinic acetylcholine receptor binding in adult and developing rat brain sections. J Neurosci Methods 153(2): 312-317.
- Corringer PJ, Sallette J, Changeux JP (2006) Nicotine enhances intracellular nicotinic receptor maturation: a novel mechanism of neural plasticity? J Physiol Paris 99(2-3): 162-171.
- Huang LZ, Winzer-Serhan UH (2006) Chronic neonatal nicotine upregulates heteromeric nicotinic acetylcholine receptor binding without change in subunit mRNA expression. Brain Res 1113(1): 94-109.
- Perry DC, Kellar KJ (1995) [3H]epibatidine labels nicotinic receptors in rat brain: an autoradiographic study. J Pharmacol Exp Ther 275(2): 1030-1034.
- Simon P, Dupuis R, Costentin J (1994) Thigmotaxis as an index of anxiety in mice. Influence of dopaminergic transmissions. Behav Brain Res 61(1: )59-64.
- Brunzell DH, Russell DS, Picciotto MR (2003) In vivo nicotine treatment regulates mesocorticolimbic CREB and ERK signaling in C57B1/6J mice. J Neurochem 84(6): 1431-1441.
- Pietila K, Ahtee L (2000) Chronic nicotine administration in the drinking water affects the striatal dopamine inmice. Pharmacol Biochem Behav 66(1): 95-103.
- Benowitz NL, Kuyt F, Jacob P 3rd, Jones RT, Osman AL (1983) Cotinine disposition and effects. Clin Pharmacol Ther 34(5): 604-611.
- Petersen DR, Norris KJ, Thompson JA (1984) A comparative study of the disposition of nicotine and its metabolites in three inbred strains of mice. Drug Metab Dispos 12(6): 725-731.
- Nesil T, Gorkem Y, Gonca M, Kanit L, Pogun S (2011) Previous chronic exposure eliminates the conditioning effect of nicotine in rats. Brain Res Bull 85(6): 339-345.
- Mesa-Gresa P, Perez-Martinez A, Redolat R (2013) Behavioral effects of combined environmental enrichment and chronic nicotine administration in male NMRI mice. Physiol Behav 115: 65-76.
- Bura SA, Burokas A, Martin-Garcia E, Maldonado R (2010) Effects of chronic nicotine on food intake and anxiety-like behaviour in CB(1) knockout mice. Eur Neuropsychopharmacol 20(6): 369-378.
- Mineur YS, Abizaid A, Rao Y, Salas R, DiLeone RJ, et al. (2011) Nicotine decreases food intake through activation of POMC neurons. Science 332(6035): 1330-1332.
- Ijomone OM, Olaibi OK, Nwoha PU (2014) Effects of chronic nicotine administration on body weight, food intake and nitric oxide concentration in female and male rats. Pathophysiology 21(3): 185190.
- Huang LZ, Winzer-Serhan UH (2007) Nicotine regulates mRNA expression of feeding peptides in the arcuate nucleus in neonatal rat pups. Dev Neurobiol 67(3): 363-377.
- Allen SS, Hatsukami D, Brintnell DM, Bade T (2005) Effect of nicotine replacement therapy on post-cessation weight gain and nutrient intake: A randomized controlled trial of postmenopausal female smokers. Addict Behav 30(7): 1273-1280.
- Ferguson SG, Shiffman S, Rohay JM, Gitchell JG, Garvey AJ (2011) Effect of compliance with nicotine gum dosing on weight gained during a quit attempt. Addiction 106(3): 651-656.
- Rowell PP, Hurst He, Marlowe C, Bennett BD (1983) Oraladministration of nicotine - its uptake and distribution after chronic administration in mice. J Pharmacol Methods 9(4): 249-261.
- Sparks JA, Pauly JR (1999) Effects of continuous oral nicotine administration on brain nicotinic receptors and responsiveness to nicotine in C57Bl/6 mice. Psychopharmacology (Berl) 141(2): 145153.
- Nuutinen S, Ahtee L, Tuominen RK (2005) Time and brain region specific up-regulation of low affinity neuronal nicotinic receptors during chronic nicotine administration in mice. Eur J Pharmacol 515(1-3): 83-89.
- Caldarone BJ, King SL, Picciotto MR (2008) Sex differences in anxiety-like behavior and locomotor activity followingchronic nicotine exposure in mice. Neurosci Lett 439(2): 187-191.
- Weiss S, Nosten-Bertrand M, McIntosh JM, Giros B, Martres MP (2007) Nicotine improves cognitive deficits ofdopamine transporter knockout mice without long-term tolerance. Neuropsychopharmacology 32(12): 2465-2478.