Differences in Tomato and Watermelon Cultivar Responses to Increasing Doses of Dicambart
Timothy C Rice, Sarah E Dixon, Xi Xiong and Reid J Smeda*
Plant Science & Technology, Purdue University, USA
Submission: July 24, 2023; Published: August 15, 2023
*Corresponding author: Reid J Smeda, Plant Science & Technology, Purdue University, USA, Email: SmedaR@missouri.edu
How to cite this article:Timothy C R, Sarah E Dixon, Xi X, Reid J S. Differences in Tomato and Watermelon Cultivar Responses to Increasing Doses of Dicamba. JOJ Pub Health. 2023; 7(4): 555719. DOI: 10.19080/JOJPH.2023.07.555719
Abstract
Dicamba can result in severe plant damage to sensitive crops, but differential tolerance to dicamba may be present among cultivars of these sensitive crops. Identifying tolerant cultivars of sensitive crops can limit the potential damage for growers if incidents of dicamba off-target movement occur. In central Missouri, a greenhouse study using seven tomato (Solanum lycopersicum) and five watermelon (Citrullus lanatus) cultivars was established to compare the responses of different specialty crop cultivars to sub-lethal doses of dicamba. A preliminary dose response with dicamba revealed 50% inhibiting doses (ID50) of 76 g ae ha-1 and 255 g ae ha-1 for ‘Mountain Merit’ tomato and ‘Crimson Sweet’ watermelon, respectively. Tomato cultivars included ‘Better Boy (Figure 1).’, ‘Brandywine Pink’, ‘Carolina Gold (Figure 1), ‘Mountain Merit (Figure 1), ‘Red Grape (Figure 1), ‘Roma VF’ and ‘Super sweet 100 (Figure 1) while watermelon cultivars included ‘Black Tail Mountain Organic’, ‘Crimson Sweet’, ‘Millionaire Figure 1, ‘Sangria Figure 1 and ‘Yellow Doll figure 1. Plants were grown until four true leaves developed and then treated with a range of dicamba doses (25.6 g ae ha-1 to 766.8 g ae ha-1). Visual injury was assessed at 7, 14 and 21 days after treatment (DAT), with plant dry weight determined at 21 DAT. Dry weight and visual injury data indicate that the range of tomato responses clearly fell into three groups: highly sensitive (‘Roma’); moderately sensitive (‘Better Boy’, ‘Brandywine Pink’ and ‘Super sweet’) and sensitive (‘Carolina Gold’, ‘Grape’ and ‘Mountain Merit’). Visual injury data for watermelon revealed that all cultivars overall responded similarly. However, plant biomass of ‘Millionaire’ and ‘Yellow Doll’ were reduced by 25% compared to their untreated controls at a dose of 28 g ae ha-1, while ‘Black Tail Mountain’, ‘Crimson Sweet’ and ‘Sangria’ dry weights required a dose of 250 g ae ha-1 to reduce biomass by 25%. Tomato and watermelon producers may consider selection of more tolerant cultivars in areas where the risk of off-target damage from dicamba exists.
Keywords: Sensitive Crops; Plant Damage; Mountain Merit; Crimson Sweet; Brandywine Pink; Black Tail Mountain Organic
Abbreviations: DT: Dicamba Tolerant; DAP: Days after planting
Introduction
The introduction of Dicamba Tolerant (DT) cropping systems in 2017 resulted in 43% of all soybeans (Glycine max) production area being planted with DT varieties in 2018 and 69% of cotton (Gossypium hirsutum) by 2019 [1,2]. However, this level of adoption also led to reports of dicamba damage to adjacent sensitive crops. In 2017 alone, 2,708 dicamba-related injury cases on sensitive crops were reported across the United States (Bradley, 2017). Potato (Solanum tuberosum), tomato and watermelon are a few crops proven to be susceptible to dicamba at low doses [3-7]. Exposure of sensitive crops to low doses of dicamba mainly occurs through off-target movement [8]. Off-target movement allows dicamba to move large distances and damage has been detected up to 250 m from an applied area [9]. Off-target movement can occur during the application of dicamba when spray droplets are carried to adjacent areas or following application via volatilization from treated areas [9]. Factors that influence particle drift include mechanical factors such as application height, herbicide formulation, spray droplet size, nozzle spacing and application speed in addition to environmental factors such as size of production area and high wind speeds. After application, high temperatures and low humidity may cause dicamba particles to volatilize into vapor particles [3]. In vapor form, dicamba is even more susceptible to off target movement via high wind speeds or temperature inversions.
Although dicamba can move long distances via off-target movement [5], significant damage to tomato, potato and watermelon has also been recorded at representative drift rates. [4] found that watermelon treated 20 days after planting (DAP) with 7.5 g ae ha-1 of the label rate of dicamba exhibited 40% visual injury and had vine lengths 49% the length of the non-treated check [6] found that tomatoes treated with 4.5 g ae ha-1 of the label rate of dicamba caused 50% visual injury 21 DAT. [7] found up to 30% visual injury on potatoes with 40% total tuber reductions at a rate of 2.8 g ae ha-1. Specialty crops such as tomato, potato and watermelon are a major concern for off-target movement due to their dicamba sensitivity, but also for their high economic value and impact on human health [4,10]. Across the United States approximately 110,000, 380,000 and 41,000 hectares of tomatoes, potatoes and watermelon were planted, respectively in 2021. These crops were worth $1.5 billion, $4 billion and $530 million, respectively in 2021 (USDA NASS, 2022). Additionally, these vegetables are also important food sources highly beneficial to human health [11]. They contain valuable food ingredients such as carbohydrates, vitamins and minerals which are vital to maintaining and repairing the body, in addition to preventing diseases [12]. To protect these valuable crops, it is essential to find new management strategies that minimize dicamba damage to these crops.
Although growers cannot control unwanted chemical trespassing from dicamba, one potential method to minimize dicamba impact is production of less susceptible cultivars. In soybeans, cultivar differences have been widely recorded for metribuzin sensitivity [13,14] recorded that some soybean cultivars exposed to soil applied metribuzin (1.7 kg ai ha -1) exhibited 100% visual injury while others exhibited as low as 35% visual injury at 4 weeks after planting. Soybean has also demonstrated cultivar differences in susceptibility to other herbicides including: bentazon, bromoxynil, chloroxuron, and 2,4-DB [15]. Significant cultivar differences in other crops such as eggplant (‘Black Beauty’ and ‘Santana’), snap bean (‘Bush Blue Lake 274’ and ‘Caprice’) and cucumber (‘Burpless Beauty’ and ‘Python’) were noted in a dicamba dose response by [16]. In tomato, potato and watermelon there is limited evidence that variability in herbicide response exists between cultivars. [17] compared the residue quantity and decay rates of 28 pesticides on two different cultivars of tomato (‘Birikino’ and ‘Tombola’) that exhibited different surface/weight ratios. The study found that the cultivar with a higher exposed leaf surface area contained more residues but also demonstrated higher residue decay rates for 18 herbicides [17].
The impact of tomato leaf surface area on herbicide susceptibility was also noted by [18] who recorded a correlation between leaf surface area and dicamba exposure. A study by [19] further demonstrated cultivar differences in tomato where the severity of injury from dicamba drift was cultivar dependent in a field setting. Limited information is available on watermelon cultivar differences to herbicides. However, a study by [20] demonstrated differences between watermelon and closely related cucurbit species, such as muskmelon, after exposure to acetochlor. Concerning potatoes, little research is available on cultivar differences to herbicide exposure. One study by [21] did demonstrate cultivar differences in the ability of potato to translocate and distribute metribuzin. This may indicate differences in cultivar metabolism which is a known method of herbicide tolerance for crops and weeds [22,23]. With broad genetic variability for traits such as fruit size, color, leaf shape and taste, tomato, potato, and watermelon cultivars may exhibit inherent differences in tolerance to dicamba. This study aimed to determine the sensitivities of seven different tomato cultivars and five different watermelon cultivars to low dose applications of dicamba. This information will help growers understand the impact dicamba has on their specialty crops. Additionally, it may reveal cultivars of these crops that demonstrate natural tolerance to dicamba at low rates.
Materials and Methods
Greenhouse experiments with tomato and watermelon were conducted in Columbia, MO between August 2021, and March 2022. Research focused on seven tomato cultivars including ‘Better Boy Figure 1’, ‘Brandywine Pink’, ‘Carolina Gold Figure 1, ‘Mountain Merit Figure 1, ‘Red Grape Figure 1, ‘Roma VF’ and ‘Supersweet 100 Figure 1and five watermelon cultivars including ‘Black Tail Mountain Organic’, ‘Crimson Sweet’, ‘Millionaire Figure 1, ‘Sangria Figure 1 and ‘Yellow Doll Figure 1. Cultivars were selected due to their differences in representative traits such as fruit size, color, growth rate, leaf shape or seed type. Seed was purchased from Harris Seeds Company (Rochester, NY, USA). Plastic pots (10 cm) were filled with a 30:70 mixture of Mexico silt loam (fine, smectitic, mesic vertic epiaqualfs) (USDA Official Soil Series Descriptions, 2022) and peat-based greenhouse media (Pro-Mix BX Mycorrhizae; Hummert International, Earth City, MO, USA). Approximately 60 seeds of each cultivar were planted across 40 pots. As seedlings emerged, plants were thinned as needed and 36 plants were selected for treatment based on the desired growth stage. Plants were exposed to a 15-hour photoperiod supplemented by high-pressure sodium lights and were handwatered daily. A macronutrient fertilizer (Peters Professional 20-20-20 General-Purpose Water-Soluble Fertilizer; Hummert International) was applied bi-weekly at 200 ppm N starting 3 weeks after planting until the end of the experiment. Tomatoes were treated when the second set of true leaves had developed, and plants were 12 to 16 cm in height. Watermelon was treated at 10 to 12 cm in height, with vining just initiating.
Dicamba application rates were determined from a prescreening trial using the tomato cultivar ‘Mountain Merit’ and the watermelon cultivar ‘Crimson Sweet’ applied with dicamba at rates of 25.6 to 511.1 g ae ha -1. These representative cultivars revealed that inhibiting doses of approximately 76 and 255 g ha-1 were required to reduce the dry weights and visual injury of tomato and watermelon by 50% (ID50), respectively. Dicamba doses used in the cultivar experiment were derived from these ID50s. Seedlings from both crops and all cultivars were exposed to nine treatments including eight different doses of dicamba ranging from 25.6 to 383.5 g ae ha-1 (tomato) or 76.7 to 766.8 g ha-1 (watermelon), along with an untreated control (Table 1). All dicamba treatments contained the diglycolamine salt of dicamba (3,6-dichloro-o-anisic acid) and Impetro® 2 (MFA Agri Service, Columbia, MO, USA) at 1% v/v. Treatments were applied using an air pressurized track sprayer calibrated to deliver 140 L ha-1 using an 8001E nozzle (TeeJet Technologies, Urbandale, IA, USA).
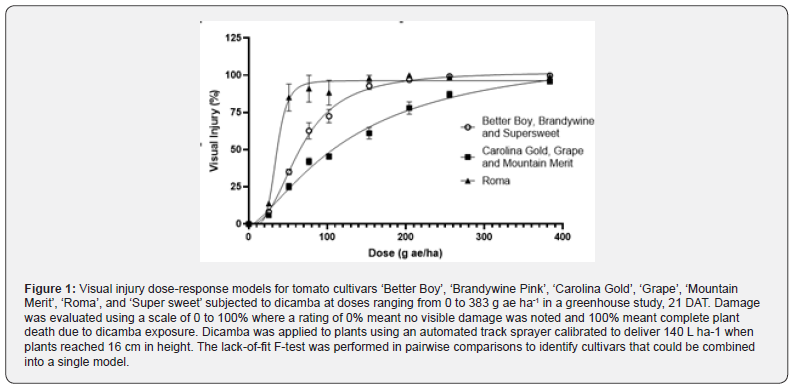
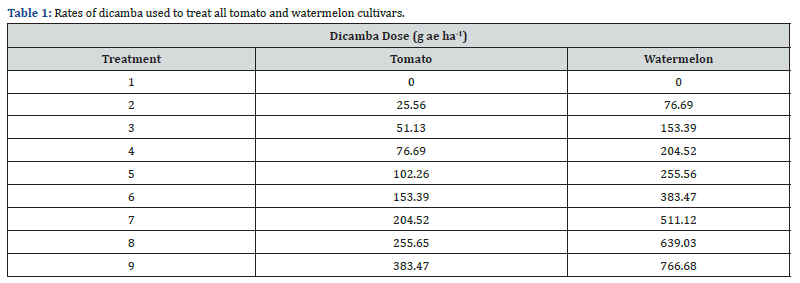
Assessment of tomato and watermelon response to dicamba included visual evaluation of damage. Damage was evaluated using a scale of 0 to 100% where a rating of 0% indicated no visible damage and 100% indicated complete plant death due to dicamba exposure. Evaluations were conducted 7, 14 and 21 days after treatment (DAT). Each plant was cut at the soil level 21 DAT and placed in paper bags, dried for seven days at 32°C and weight recorded. Once dried, each plant was weighed individually. To facilitate comparison of different cultivars, dry weights for treated plants were converted to percentage of control plants using the mean for untreated control for each respective cultivar. The experimental design was a randomized complete block design with four replications. Each experiment with all cultivars was repeated. A total of 8 plants were used to generate each data point. Dicamba dose and cultivars were considered fixed variables, whereas replications within treatments were considered random. Visual injury and dry weight data were analyzed using a log-logistic analysis [24] of non-linear regression models in GraphPad-Prism 9.1.0 (Graphpad Software Inc., San Diego, CA, USA).
Results and Discussion
Tomato. A log-logistic equation was used to determine the best line of fit for the visual injury responses of each tomato cultivar. A pairwise comparison of models for each cultivar was done using a lack-of-fit F-test to test the hypothesis that the curves have the same slope when α = 0.05. Alternatively, this test determines if the more complex model (nonparallel curves) can be reduced to a simpler model (parallel curves). Nonsignificant pairs were identified and combined into models, and the lack-offit F-test was repeated. The 7 tomato cultivars were separated into 3 model groups considered to be significantly different (P < 0.0001): highly sensitive (‘Roma’); moderately sensitive (‘Better Boy’, ‘Brandywine Pink’ and ‘Super sweet’) and sensitive (‘Carolina Gold’, ‘Grape’ and ‘Mountain Merit’) (Table 2). This process was repeated for dry weights which resulted in the same grouping based on relative sensitivity (Table 3).
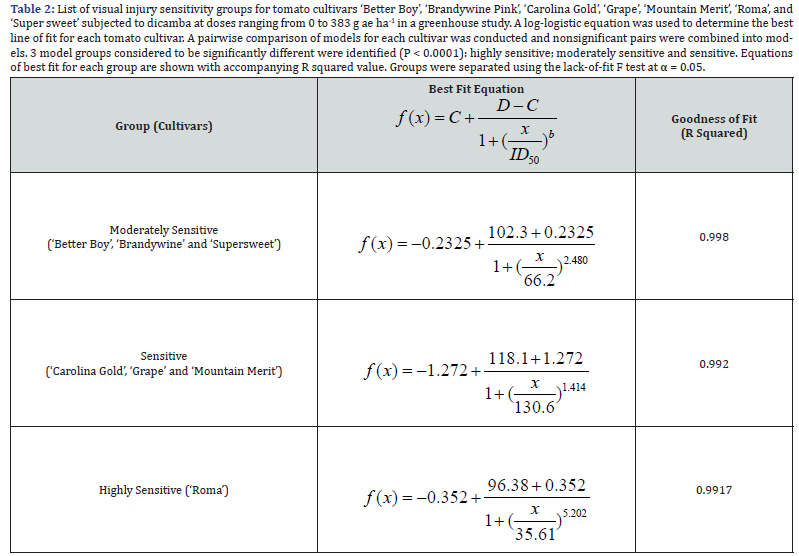
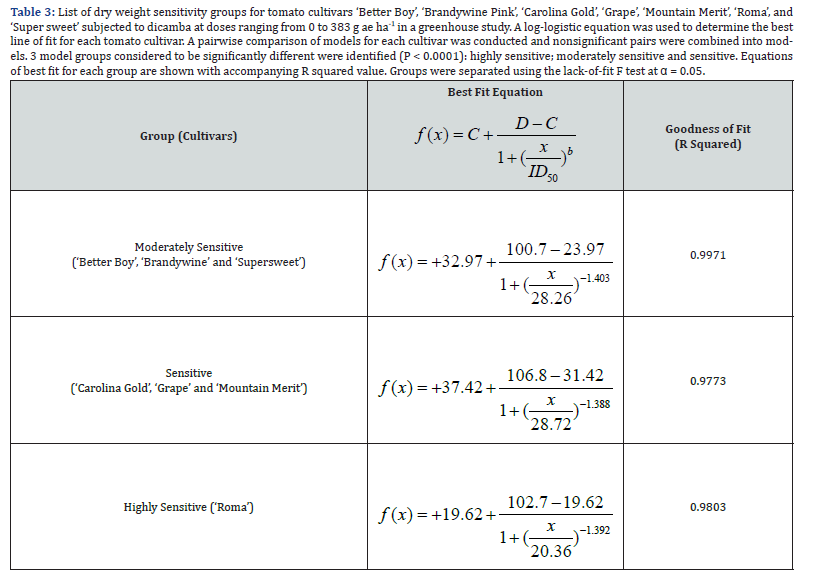
Although visual injury from dicamba occurred on treated tomatoes at all observation dates with optimum symptomology for cultivars noted at 21 DAT. Therefore, only this date was selected to show the results. Dicamba injury to tomato was primarily manifested as leaf cupping, stem thickening and stem twisting with callous formation across all timings. By 21 DAT, leaf defoliation and necrosis were observed at higher rates. At a dose of 51.1 g ha-1, highly sensitive tomatoes exhibited almost complete plant death (85% visual injury) while moderately sensitive and sensitive tomatoes sustained damage of 35% and 25%, respectively Figure 1. At a dose of 102.3 g ae ha-1, highly sensitive, moderately sensitive, and sensitive tomatoes displayed visual damage of 88.3%, 72.5% and 45%, respectively. The visual injury ID50 of each group were 35.61, 66.2 and 130.6 g ha-1 for highly sensitive, moderately sensitive, and sensitive tomatoes, respectively. At the highest dose of 383.5 g ha-1, plants from all three groups died.
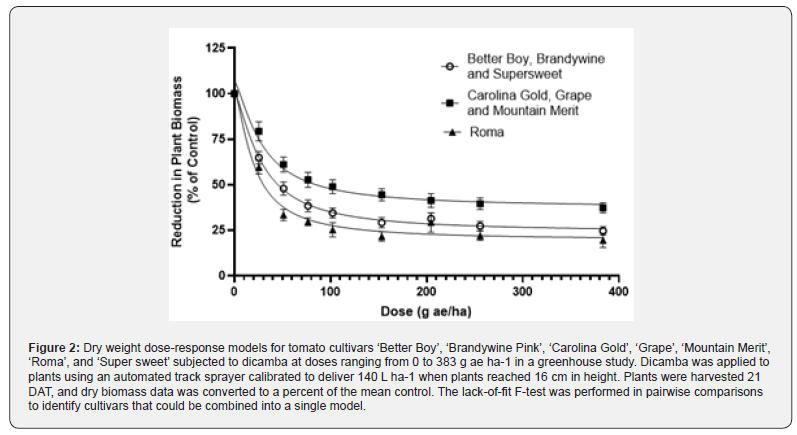
Tomato dry weight data resulted in the same sensitivity groupings as the visual injury (Figure 2). At a dose of 102.3 g ha -1, highly sensitive, moderately sensitive, and sensitive tomatoes had mean dry weights of 25.3, 34.5 and 48.9% of the untreated control, respectively. The dry weight ID50 of each group was 20.4, 28.3 and 28.7 g ha-1 for highly sensitive, moderately sensitive, and sensitive tomatoes, respectively. At the highest dose of 383.5 g ha -1, highly sensitive, moderately sensitive, and sensitive tomatoes had dry weights of 19.5, 24.7 and 37.4% of the untreated control, respectively. Watermelon. A log-logistic equation was used to determine the best line of fit for the dry weight responses of each watermelon cultivar (Table 4). A pairwise comparison of models for each cultivar was done using a lack-of-fit F-test to test the hypothesis that the curves have the same slope when α = 0.05. Alternatively, this test determines if the more complex model (nonparallel curves) can be reduced to a simpler model (parallel curves). Non-significant pairs were identified and combined into models, and the lack-of-fit F-test was repeated. The 5 watermelon cultivars were separated into 2 model groups considered to be significantly different (P < 0.0001): highly sensitive (Millionaire and Yellow Doll) and sensitive (Black Tail Mountain, Crimson Sweet and Sangria) (Table 4). This process was repeated for visual injury but there were no significant differences between watermelon cultivars (Table 5).
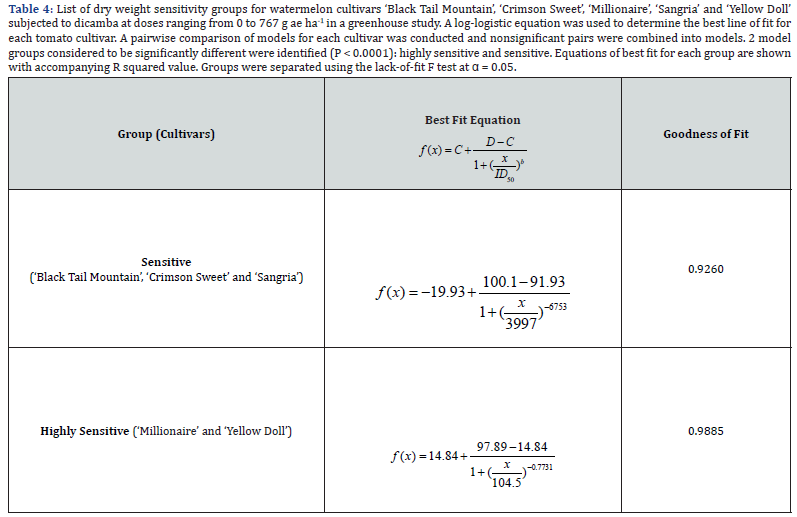
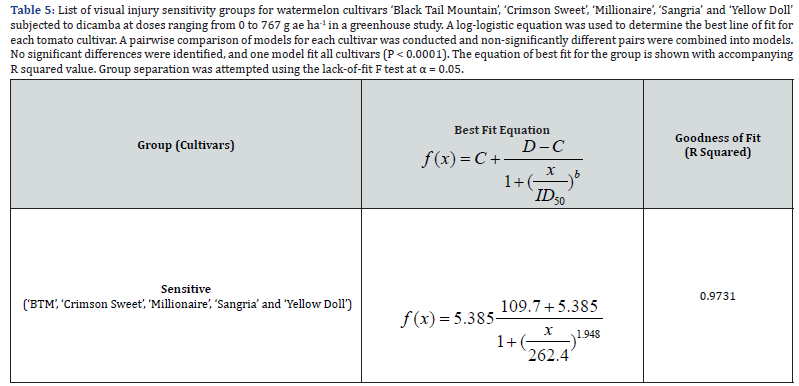
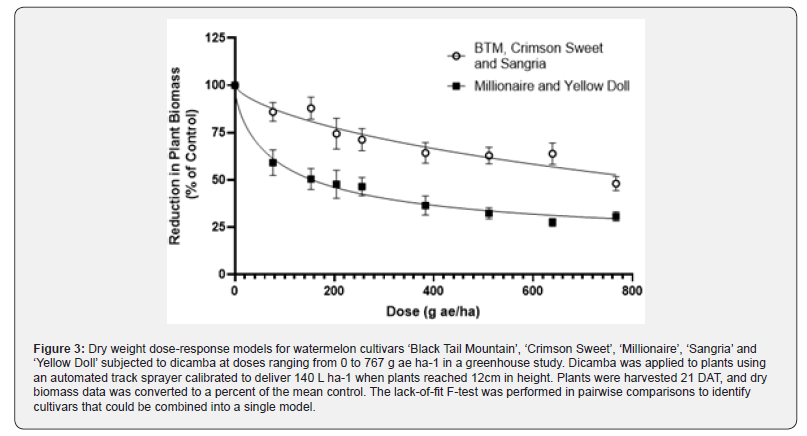
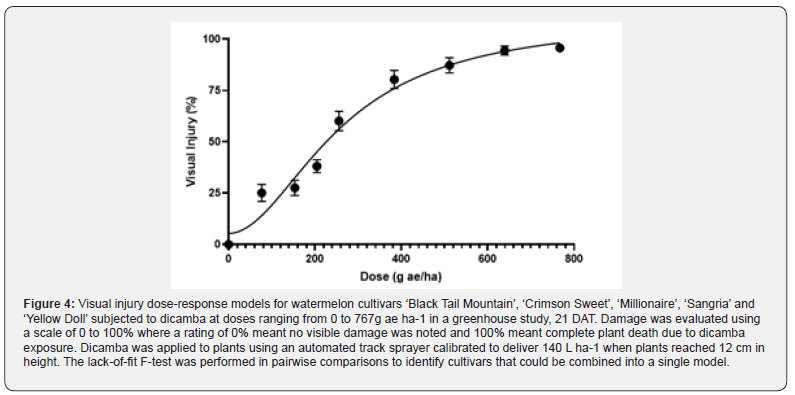
Dry weight data suggest that highly sensitive watermelon had consistently lower dry weights when compared to sensitive watermelon (Figure 3). At a dose of 204.5 g ha-1, highly sensitive and sensitive watermelon had dry weights of 47.7 and 74.4% of the untreated control, respectively. The dry weight ID50 of each group was 104.5 and 262.7 g ha-1 for highly sensitive and sensitive watermelon, respectively. At the highest dose of 766.7 g ha-1, highly sensitive and sensitive watermelon had dry weights of 30.7% and 48.1% of the untreated control, respectively. Similar to tomato, the visual injury data from 21 DAT proved most effective at highlighting cultivar differences for watermelon and injury was primarily observed as stem thickening and twisting with callous formation across all timings. By 21 DAT, leaf defoliation and necrosis were also observed at higher rates. The extra sumof- squares F test indicated that one curve fit all 5 watermelon cultivars (P < 0.1026) (Table 5). This curve indicates that a dose of approximately 262.4 g ha-1 dicamba was sufficient to result in a 50% visual injury rating for all 5 cultivars (Figure 4).
Results from this study reinforce that tomato and watermelon plants are sensitive to low dose applications of dicamba, although, the ID50 concentration was approximately four-fold higher for watermelon when compared to tomato. [4,6] recorded damage on tomatoes and watermelon treated with 4.5 and 7.5 g ae ha-1 of dicamba at 21 and 20 DAT, respectively. Results from this study determined that multiple cultivars of tomatoes and watermelon exhibited injury with dicamba rates as low as 25.6 and 76.7 g ha-1, respectively. These doses also reduced dry weights of tomato and watermelon by approximately 25% when compared to the untreated controls. [4,6] used rates lower than those used in this experiment, but doses remained low when compared to the recommended use rate of dicamba (560 g ae ha-1). This study also reinforces that herbicide sensitivities can vary between cultivars. [25] found cultivar differences in tomato response to dicamba, while earlier studies demonstrated differences in herbicide sensitivities between cultivars of soybean, cucumber, eggplant, and other crops [13,14,16]. The seven tomato cultivars tested in this study were shown to have 3 different levels of sensitivity: highly sensitive (‘Roma’); moderately sensitive (‘Better Boy’, ‘Brandywine Pink’ and ‘Super sweet’) and sensitive (‘Carolina Gold’, ‘Grape’ and ‘Mountain Merit’) (Tables 2 & 3). These groupings were reinforced by both the visual injury and dry weight data (Figures 1 & 2). This data indicates that cultivars grouped as ‘sensitive’ may be good cultivars to be used in areas where dicamba off-target movement may occur. Furthermore, the data indicates that the highly sensitive cultivar ‘Roma’ should potentially be avoided in the same high-risk areas.
The five watermelon cultivars tested in this study were shown to have 2 different levels of sensitivity: highly sensitive (‘Millionaire’ and ‘Yellow Doll’) and sensitive (‘Black Tail Mountain’, ‘Crimson Sweet’ and ‘Sangria’) (Table 4). However, these groupings were only reinforced by dry weight data (Figure 3). Visual injury data indicated no cultivar differences in sensitivity to dicamba (Figure 4). Differences in dry weight for watermelon may be attributed to differences in growth rates observed in untreated controls. Even though all watermelon cultivars were applied with dicamba at the same heights, untreated controls continue to grow at normal rates. Cultivars with naturally slower growth rates may experience reduced effects from dry weight reductions due to dicamba exposure. Additionally, the gradual decline of the dose response curves for watermelon dry weights indicates that there is less acute sensitivity for all watermelon cultivars towards dicamba (Figure 3). Considering these factors and the visual injury data, it is possible that these five watermelon cultivars do not significantly differ in dicamba sensitivity. However, dry weight data still indicates that watermelon grouped as ‘sensitive’ may provide a small amount protection in areas where dicamba offtarget movement may occur. Additionally, the ‘highly sensitive’ cultivars may be more susceptible and should potentially be avoided in high-risk areas. The differences in sensitivity noted in this study could be related to natural growth rates as discussed above, leaf surface area as demonstrated by [17,18] or due to increased herbicide metabolism in some cultivars [22,23]. However, more work is needed to accurately identify tolerant cultivars of both crops and reduce the impact of dicamba damage on affected specialty crops in production areas. The data from this study reinforces that watermelon shows decreased sensitivity to dicamba when compared to tomato. Additionally, cultivar differences in both tomato and watermelon sensitivity to dicamba were observed under the conditions of this greenhouse experiment [24-39].
References
- Wechsler S J, Smith D, McFadden J, Dodson L, Williamson S (2019) The use of genetically engineered dicamba-tolerant soybean seeds has increased quickly, benefiting adopters but damaging crops in some fields. Amber Waves: The Economics of Food, Farming, Natural Resources, and Rural America.
- Dodson L, Wechsler SJ, Williamson S, McFadden J, Smith D (2021) Adoption of genetically engineered dicamba-tolerant cotton seeds is prevalent throughout the United States. Amber Waves: The Economics of Food, Farming, Natural Resources, and Rural America.
- Bohnenblust EW, Vaudo AD, Egan F, Mortensen DA, Tooker JF (2016) Effects of the herbicide dicamba on nontarget plants and pollinator visitation. Environ Toxicol Chem 35(1): 144-151.
- Culpepper AS, Sosnoskie LM, Shugar J, Leifheit N, Curry M, et al. (2018) Effects of low-dose applications of 2, 4-D and dicamba on watermelon. Weed Technology 32(3): 267-272.
- Jones G T, Norsworthy J K, Barber L T, Scott R C (2015) Comparison of two dicamba formulations for risk of off-target movement to soybean. Soybean Research Studies pp. 124.
- Knezevic SZ, Osipitan OA, Scott JE (2018) Sensitivity of grape and tomato to micro-rates of dicamba-based herbicides. J Hortic 5: 229.
- Wall DA (1994) Potato (Solanum tuberosum) response to simulated drift of dicamba, clopyralid, and tribenuron. Weed Science 42(1): 110-114.
- Fagliari JR, Oliveira RSD, Constantin J (2005) Impact of sublethal doses of 2, 4-D, simulating drift, on tomato yield. J Environ Sci Health B 40(1): 201-206.
- Soltani N, Oliveira MC, Alves GS, Werle R, Norsworthy JK, et al. (2020) Off-target movement assessment of dicamba in North America. Weed Technol 34(3): 318-330.
- Kruger G R, Johnson W G, Doohan D J, Weller S C (2012). Dose response of glyphosate and dicamba on tomato (Lycopersicon esculentum) injury. Weed Technology 26(2): 256-260.
- Oguntibeju OO, Truter E, JEsterhuyse A J (2013) The role of fruit and vegetable consumption in human health and disease prevention. Diabetes Mellitus-Insights and Perspectives 3(2): 172-180.
- Hanif R, Iqbal Z, Iqbal M, Hanif S, Rasheed M (2006) Use of vegetables as nutritional food: role in human health. Journal of Agricultural and Biological Science 1(1): 18-22.
- Hardcastle WS (1974) Differences in the tolerance of metribuzin by varieties of soybeans. W. S. Hardcastle 14(3): 181-184
- Wax LM, Stoller EW, Bernard RL (1976) Differential response of soybean cultivars to metribuzin1. Agronomy Journal 68(3): 484-486.
- Wax LM, Bernard RL, Hayes RM (1974) Response of soybean cultivars to bentazon, bromoxynil, chloroxuron, and 2, 4-DB. Weed Science 22(1): 35-41.
- Wasacz MH, Ward DL, VanGessel MJ, Besancon E (2022) Sensitivity to sublethal rates of dicamba for selected mid-Atlantic vegetable crops. Weed Technol 36: 207-213.
- Dedola F, Cabizza M, Satta M (2014) Determination of 28 pesticides applied on two tomato cultivars with a different surface/weight ratio of the berries, using a multiresidue GC-MS/MS method. J Environ Sci Health B 49(9): 671-678.
- Zangoueinejad R, Alebrahim MT, Tseng TM (2020) Absorption and translocation of dicamba in dicamba-tolerant wild tomato. Canadian Journal of Plant Science 101(1): 30-38.
- Warmund M, Ellersieck M, Smeda RJ (2022) Agriculture 12(9): 1489.
- Song C, Teng C, Tian L, Ma H, Tao B (2006) Seedling growth tolerance of Cucurbits crops to herbicides Stomp and Acetochlor. Gen Appl Plant Physiology 32(3-4): 165-174.
- Gawronski SW, Haderlie LC, Callihan RH, Dwelle RB (1985) Metribuzin absorption, translocation, and distribution in two potato (Solanum tuberosum) cultivars. Weed Science 33(5): 629-634.
- Ohkawa H, Tsujii H, Ohkawa Y (1999) The use of cytochrome P450 genes to introduce herbicide tolerance in crops: a review. Pesticide Science 55(9): 867-874.
- Smeda RJ, Vaughn KC (1997) Mechanisms of resistance to herbicides. Molecular Mechanisms of Resistance to Agrochemicals pp. 79-123.
- Seefeldt SS, Jensen JE, Fuerst EP (1995) Log-logistic analysis of herbicide dose-response relationships. Weed Technol 9(2): 218-227.
- Warmund M, Ellersieck M, Smeda RJ (2022) Agriculture 12(9): 1489.
- Gawronski SW, Haderlie LC, Callihan RH, Dwelle RB (1985) Metribuzin absorption, translocation, and distribution in two potato (Solanum tuberosum) cultivars. Weed Science 33(5): 629-634.
- Hanif R, Iqbal Z, Iqbal M, Hanif S, Rasheed M (2006) Use of vegetables as nutritional food: role in human health. Journal of Agricultural and Biological Science 1(1): 18-22.
- Hatzi Regulation (1997) Regulation of enzymatic systems detoxifying xenobiotics in plants Springer Science & Business Media
- Heap IM (2010) International survey of herbicide resistant weeds. Online. WeedScience.org, Corvallis, OR.
- Johnson B, Young B, Matthews J, Marquardt P, Slack C, et al. (2010) Weed control in dicamba-resistant soybeans. Crop Management 9(1): 1-23.
- Lovelace ML, Talbert RE, Scherder E, Hoagland RE (2007) Effects of multiple applications of simulated quinclorac drift rates on tomato. Weed Science 55(2): 169-177.
- Monsanto Corporation (2016) Xtendimax® product label. St. Louis, Monsanto.
- Seefeldt SS, Jensen JE, Fuerst EP (1995) Log-logistic analysis of herbicide dose-response relationships. Weed Technol 9(2): 218-227.
- Soil Survey, Natural Resources Conservation Service, United States Department of Agriculture. Official Soil Series Descriptions.
- Song C, Teng C, Tian L, Ma H, Tao B (2006) Seedling growth tolerance of Cucurbits crops to herbicides Stomp and Acetochlor. Gen. Appl. Plant Physiology 32(3-4): 165-174.
- USDA- National Agricultural Statistics Service (USDA NASS) (2022) Statistics by Subject.
- Warmund M, Ellersieck M, Smeda R J (2022) Agriculture 12(9): 1489.
- Young BG, (2006) Changes in herbicide use patterns and production practices resulting from glyphosate-resistant crops. Weed Technol 20: 301-307.
- Zangoueinejad R, Alebrahim MT, Tseng TM (2020) Absorption and translocation of dicamba in dicamba-tolerant wild tomato. Canadian Journal of Plant Science 101(1): 30-38.