The Physiology of Cornea
Balyen L*
Department of Ophthalmology, Harran University School of Medicine, Sanliurfa, Turkey
Submission: December 07, 2022;Published: December 19, 2022
*Corresponding author: Lokman Balyen, Assistant Professor, Department of Ophthalmology, Harran University School of Medicine, Sanliurfa, Turkey
How to cite this article: Balyen L. The Physiology of Cornea. JOJ Ophthalmol. 2022; 9(3): 555764. DOI: 10.19080/JOJO.2022.09.555764
Abstract
The cornea has important functions such as protecting the structures within the globe, contributing to the refractive power of the eye, and focusing the light rays on the retina with minimum scattering. Although the neurobiological complexity of the retina and the dynamic movement of the lens are absent in the cornea, it is one of the most critical components of the perfect visual system, thanks to its transparency. In order to provide sustainable transparency in the cornea, which is very important for visual function, it is possible with the intense metabolic activity in the cornea and the perfect operation of the balanced ion liquid pump. The cornea provides excellent vision thanks to the structure and functions necessary for its unique function to continue regularly. This review focuses on cornea and tear film structure and physiology.
Keywords: Cornea; Physiology; Tear Film; Vision
Abbreviations: ECM: Extracellular Matrix; MMPs: Matrix Metalloproteinases
Introduction
The cornea, smooth and transparent, is an avascular tissue with a convex outer surface and a concave inner surface (). This transparent avascular tissue that in conjunction with the sclera forms the outer portion of the bulbus oculi and plays a great role in maintaining clear vision and eye health. The main purpose of this layer is to protect structures inside the eye and transmit and focus light onto the retina with its transparency and smooth curved surfaces features. The cornea is connective tissue that also acts as a structural barrier, protects the eye against infections and other harmful particles, provides a proper anterior refractive surface for the eye together with the tear film and contributes to two-third of the refractive power of the eye. It is that acts as the primary barrier against infection and mechanical damage to the internal structures of the eye [1-3].
The cornea has a unique structure that is one of the few avascular and transparent tissue in the body. Corneal transparency is maintained owing to the balance between the pressures that cause either input of the fluid into stroma or output from it. While stromal swelling pressure and intraocular pressure draw water into the stroma, endothelial and epithelial ion transports ensure the dehydration of the cornea. Tear film evaporation is another factor that contributes to this dehydration state. Additionally, there are two barriers that prevent the movement of the fluid in both directions (anterior and posterior): the epithelial barrier and the endothelial barrier [4-6]. It is also one of the most extremely innervated and sensitive tissues in the body, it is known that the density of nerve endings of corneal tissue about 300-400 times greater compared to the skin. Due to numerous nerve density in the cornea, the corneal diseases may be painful [7-11]. The cornea is composed of cellular and acellular components. The cellular components include epithelial and endothelial cells, keratocytes, while acellular components include collagen and glycosaminoglycans.
The epithelial cells are derived from epidermal ectoderm, while keratocyte and endothelial cells are derived from neural crest. The structure of the human cornea consists of five layers from anterior to posterior: corneal epithelium (50μm thick), Bowman’s (basement) membrane (12μm), corneal stroma (480-500μm), Descemet’s (basement) membrane (8-10μm), and the inner surface endothelium (5μm) (Figure 2). However, a new cornea layer, Dua’s layer, has been also described recently. Each of these layers has its own role in order to maintain normal visual function [2,3,9-11]. In this paper, we reviewed the development, structure, function and physiological features of corneal epithelium, Bowman layer, corneal stroma, Dua’s layer, Descemet’s membrane and corneal endothelium from a new perspective.
Methods
We evaluated the structure and physiology of the cornea and and tear film by searching the Cochrane Library, PubMed, Medline, Embase, Science Direct, Web of Science, Scopus and Google Scholar databases for review research and original article studies published in English. The terms such as “cornea”, “tear film”, “physiology”, and “vision’’ were used for this current research. Articles focusing on the physiology of cornea and ocular surface were reviewed.
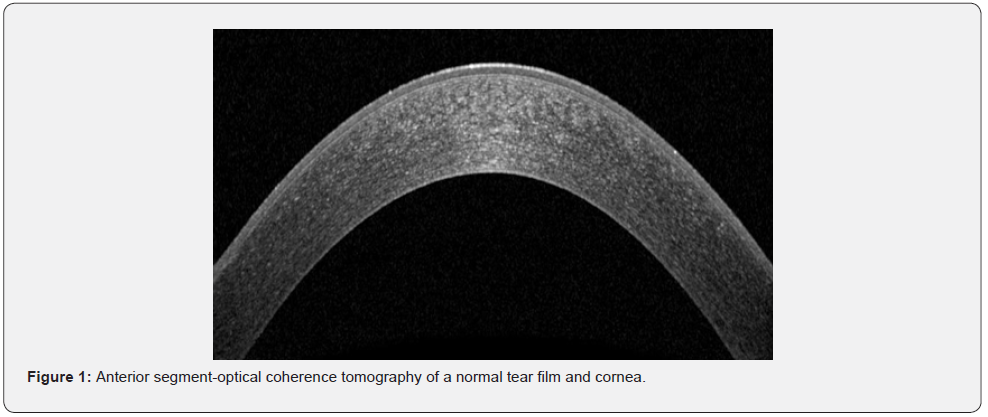
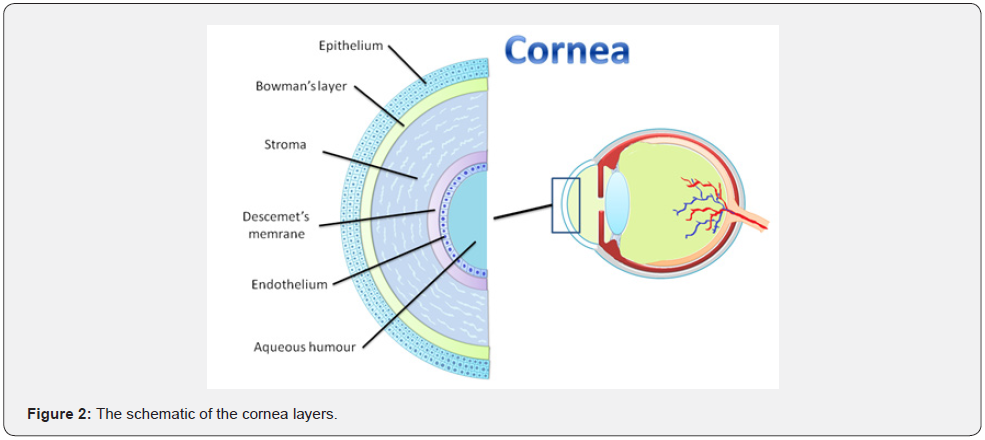
The Embryology of the Cornea
Corneal development begins with presumptive corneal epithelium formation from the cranial ectoderm adjacent to the lens placode. Neural crest cells migrate to the field between the lens and presumptive corneal epithelium to form the corneal endothelium and the stromal keratocytes. The interactions between neural ectoderm-derived optical vesicles and the cranial ectoderm create the presumptive corneal epithelium and other epithelium of the ocular surface. The sensory nerves that innervate the corneal stroma and epithelium stem from the neural crest- and ectodermal placode-derived trigeminal ganglion [12].
The Anatomy of the Cornea
The horizontal diameter of an average adult human cornea is 11.70mm and the vertical diameter is approximately 1.00mm less than the horizontal diameter. The diameter of cornea ranges from 11.04-12.50 mm in males and 10.70-12.58mm in females. The horizontal diameter of an average cornea is 11.77 ± 0.37 mm in adult males, while it is 11.64 ± 0.47 mm in adult females and the vertical diameter is approximately 1 mm less than the horizontal diameter. The cornea, convex and aspheric, is refractive index is 1.376. The anterior curvature is 7.80mm and posterior curvature is approximately 6.50mm.
The cornea, the lens, ocular media, and the axial length of the eye mainly constitute the total refractive power of the eye. The cornea refractive power ranges from 40 to 44 diopters, accounting approximately two-thirds of the total refraction power of the eye globe. There is a gradual increase in thickness from the central cornea to the limbus due to an increase in the amount of collagen in the peripheral stroma. However, corneal thickness decreases with age. In many studies, in normal eyes, the central corneal thickness is between 551μm and 565μm and the peripheral corneal thickness is between 612μm and 640μm. However, the human corneal thickness is generally 500μm -600μm in central area and 600μm-800μm in peripheral area [3,8,13-15].
Blood Supply And Lymphatics
The cornea of human is normally avascular. Because of the significance of optical transparency and smooth curved surfaces of the cornea for visual function, it does not include any blood vessels. The aqueous humor is the main source of nutrients for the survival and viability of the cornea. In addition to the aqueous humor, the cornea receives the nutrients and oxygen from small vessels at the outer edge of the cornea and gets from the end branches of the facial and ophthalmic arteries through the aqueous humor and the tear film. That is, it receives nutrients through diffusion from tear fluid externally and from aqueous humor internally. The nerve fibers innervating the cornea also provide it with neurotrophins [3].
In ocular lymphatic studies, corneal tissue has been well studied and characterized. Corneal lymphatics are induced after infectious, inflammatory, traumatic, toxic or chemical events. Lymphatics can be induced in the cornea independently of blood vessels. The lymphatic pathway plays a critical role in stimulating corneal transplantation immunity [16].
Nerves
The cornea is one of the most intensely innervated and sensitive tissues in the human body. Long ciliary nerves originating from the ophthalmic branch of the trigeminal nerve transport the sensory innervation of the cornea. The sensation is based on the nasociliary branch of the ophthalmic division of the trigeminal nerve. The corneal epithelium includes thousands of nerve endings and it has high sensitivity to contact with foreign bodies. Thick and straight stromal nerve bodies extend laterally and anteriorly to create progressively thin nerve fibers at various levels within the stroma. The nerve fibers perforate Bowman’s layer and create a dense nerve plexus beneath the basal epithelial cell layer characterized by nerve fibers interconnected by many nerves. The autonomic sympathetic nerve fibers also present in the cornea [3,17].
Tear Film
The tear film is the first layer of the cornea and provides lubrication and hydration to the ocular surface. It also has oxygen, immunoglobulins, lysozymes, lactoferrin, and α- and β-defensins. The tear film typically consists of three distinct layers, including lipid, aqueous, and mucin. The most superficial layer consists of lipid secretions of the meibomian glands. The outermost lipid layer acts as an important barrier against evaporation of the tear film. The aqueous middle layer lies beneath the lipid layer (Figure 3). It is secreted from the lacrimal gland located in the superior lateral orbit.
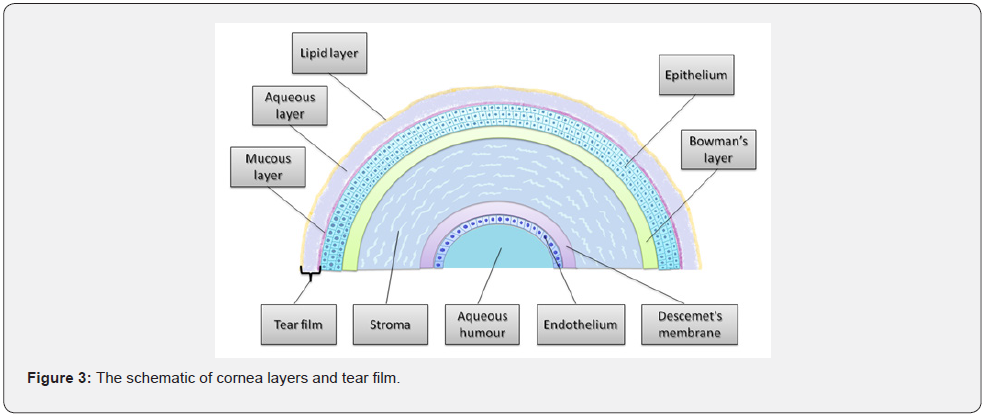
Apart from the main lacrimal gland, there are also many scattered accessory lacrimal glands embedded in the conjunctival stroma contributing to the aqueous layer. The conjunctival goblet cells mainly produce the innermost mucin layer, but the epithelial cells of the cornea and conjunctiva also play a role in the production of the underlying mucin layer. The current understanding is that the three layers together form a gel on the ocular surface. Conjunctival goblet cells appear to play an important role in performing the functions of debris removal and immune surveillance [3,18].
The Cornea Layers
The cornea is composed of cellular and acellular components. The cellular components include epithelial and endothelial cells, keratocytes, while acellular components include collagen and glycosaminoglycans. The epithelial cells are derived from epidermal ectoderm, while keratocyte and endothelial cells are derived from the neural crest. The structure of the human cornea consists of five layers from anterior to posterior: corneal epithelium (50μm thick), Bowman’s (basement) membrane (12μm), corneal stroma (480-500μm), Descemet’s (basement) membrane (8-10μm), and the inner surface endothelium (5μm) (Figure 2). However, a new cornea layer, Dua’s layer, has been also described recently. Each of these layers has its own role in order to maintain normal visual function [2, 3, 9-11].
Epithelium
The corneal epithelium, about 50μ in thickness, is composed quite uniformly of 5-7 stratified of cells. The epithelium is uniform to ensure a smooth regular surface and is composed of nonkeratinized squamous epithelium layer. The epithelium is formed surface ectoderm between 5 and 6 weeks of gestation. The tear film and underlying the epithelium have a close symbiotic relationship to provide hydrophilic spreading of the tear film on cornea with each eyelid blink. The mucin layer of the tear film produced by the conjunctival goblet cells is in direct contact with the corneal epithelium. Corneal epithelial cells have an average lifespan of 7 to 10 days.
As in lens epithelial cells, the asset of extreme concentrations of intracytoplasmic enzyme crystalline may play a critical role in maintaining optical transparency that very important for visual functions. The epithelium has 5-6 layers structure with three types of cells: superficial cells, wing cells, and basal cells. The superficial cells consist of 2-3 layers of polygonal cells. The superficial cells have the microvilli and desmosomes. The former increases the surface area, while the latter create the tight junction in between the superficial cells.
The wing cells are 2-3 layered and express 64-k Dalton keratins. The basal cells, single layer of the epithelium, are cuboidal or columnar and they are very active mitotically due to having plenty organelles. Besides stem cells, basal cells are the only corneal epithelial cells that can perform mitosis. Desmosomes are present along the epithelial cells to allow the epithelial cells adherent to each other. The hemidesmosomal system connects the basal cells and the underlying basement membrane together. The strong attachment is very important, thanks to this arrangement prevents the epithelium getting to separate from the underlying layers. With the abnormality of this adherence, it causes corneal erosions and nonhealing epithelial defects [3].
The basement membrane thickness of epithelial cells is approximately 40-60nm and consists of Type IV collagen and lamine secreted by basal cells. When the basement membrane is damaged, fibronectin levels are increased, and the healing process may last up to 6 weeks. The epithelium of central and peripheral cornea is different from each other. The epithelium has 5-7 layers in the central cornea. The basal cells are columnar without melanocytes or Langerhans cells. There is a smooth basal cell layer with keratan sulfate, and without lymphatics. On the contrary, the epithelium is 7-10 layered in the peripheral cornea. The basal cells are cuboidal with melanocytes and Langerhans cells. There are undulating extensions of basal layer without keratan sulfate and lymphatics [3].

The cornea has a unique structure that is one of the few avascular and transparent tissue in the body. Corneal transparency is maintained owing to the balance between the pressures that cause either input of the fluid into stroma or output from it. While stromal swelling pressure and intraocular pressure draw water into the stroma, endothelial and epithelial ion transports ensure the dehydration of the cornea. Tear film evaporation is another factor that contributes to this dehydration state. Additionally, there are two barriers that prevent the movement of the fluid in both directions (anterior and posterior): the epithelial barrier and the endothelial barrier (Figure 4) [4-6].
Bowman’s Membrane
The Bowman’s membrane is a 12μm structure and consists of type I and V collagen as well as proteoglycans. It only extends at the anterior of the stroma and is not a real membrane and helps to maintain the cornea’s shape. It has no regenerative ability and when injured, this layer does not regenerate and may result in a scar. It does not have the ability to regenerate and if it is injured, this layer can not be renewed and may cause a scar [3].
S t r o m a
Corneal stroma, the thickest layer of the cornea, is anatomically located between the outer epithelium and the inner endothelium and it plays a principal role in normal visual function. A variety of cellular products such as extracellular matrix (ECM) components, growth factors, cytokines, and kinases are produced by keratocytes in the stroma. These products support the cornea in order to provide the development, stability and hemostasis of the cornea [2].
The corneal stroma constitutes the majority of the structural framework of the cornea. In other words, it accounts for approximately 80%-85% of its thickness. Embryologically, th stroma is the result of the second neural crest migration wave that happens at the 7th gestational week after formation of the primitive endothelium. Due to flawless organization of stromal fibers and ECM, the stroma is characteristically transparent. Type I collagen is very common in corneal fibrils of the stroma layer. There are also type VI and type XII collagens in stroma layer [3,19].
The corneal stroma contains keratocytes and about 300 collagen lamellae arranged regularly. In addition to keratocytes, the corneal stroma is composed of ECM. The ECM is made of type I, III, V, and VI collagens and glycosaminoglycans. The glycosaminoglycans constitute keratan sulfate, chondroitin sulfate, and dermatan sulfate. The glycosaminoglycans are predominantly keratan sulfate. Less contribution is by dermatan sulfate and chondroitin sulfate. However, hyaluronan is seen in infancy. The main cell type of the stroma are keratocytes involved in maintaining the ECM environment. Keratocytes can synthesize collagen molecules and glycosaminoglycans, also creating matrix metalloproteinases (MMPs), all important in the maintenance of stromal homeostasis. Most of the keratocytes are present in the anterior stroma [3].
Dua’s Layer
There is a layer which is a novel, well-defined, acellular, strong layer in the pre-Descemet’s cornea between stroma and Descemet’s membrane. Cornea Dua’s layer was defined as a pre- Descemet’s membrane in 2013, with an average thickness of 10.15 ± 3.60 microns composed of 5 to 8 lamellae of predominantly type- 1 collagen bundles. The recognition of the Dua’s layer enlightens the corneal biomechanics, the posterior corneal surgery and pathology (acute hydrops, Descematocele and pre-Descemet’s dystrophies) [10].
Descemet’s Membrane
Descemet’s membrane has a 7μm thickness of structure and is made up of type IV collagen and laminin. It begins in uterus at the 8-week of gestation term. The Descemet’s membrane is continually secreted by endothelial cells. Before birth it has 3μm distinctive banded appearance while after birth it is unbanded and has an amorphous ultrastructural elastic texture. Descemet’s membrane can reach up to 10μm with age [3].
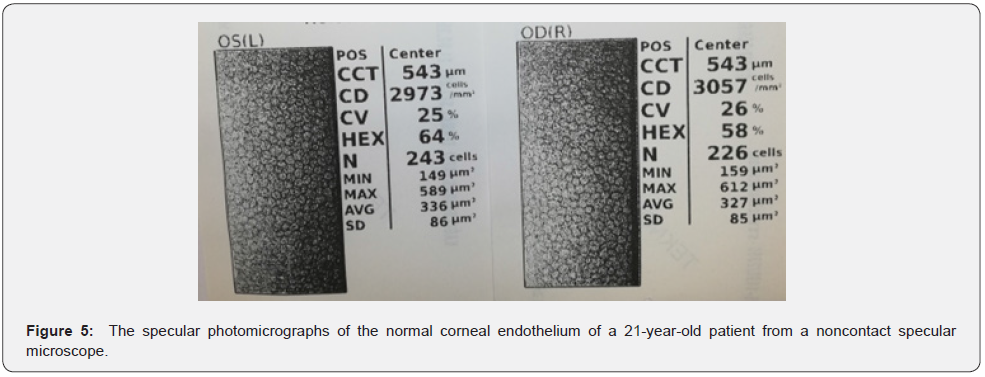
Endothelium
The endothelium, a monolayer of cells, is hexagonal cells of 5μm thick on the posterior corneal surface (Figure 5). The endothelium, metabolically active, has an endothelial pump regulating water content that transports water from the stroma into the anterior chamber. It looks like a honeycomb-like mosaic when viewed from the posterior side of endothelium. Adjacent cells have gap and tight junctions along the lateral borders and have extensive lateral associations. There are the high density of Na+/K+ ATPase pump sites in the lateral membrane. In short, this movement of water is vital for maintaining a transparent cornea and sustaining a normal visual function in this respect.
The corneal endothelium layer keeps corneal transparency by stabilizing its hydration through barrier and pump functions. If the corneal endothelial cells Corneal endothelial cells (CECs) are damaged, it can lead to serious visual impairment and even cause blindness. CECs are essential to maintain corneal transparency. If CECs lose their vital function after endothelial diseases or trauma, corneal edema and blindness are ultimately inevitable. Since mature human CECs do not proliferate in vivo and cannot be renewed, replacement of the diseased or damaged endothelium layer with corneal transplantation is currently the only treatment option [3,20-27].
The endothelium contains two substantial ion transport systems including the membrane-bound Na+/K+ ATPase and the intracellular carbonic anhydrase pathways. Thanks to the regular activity in both pathways, it produces the uninterrupted flux of ions from the stroma layer to the aqueous humor. The basal surface of the endothelium contains many hemidesmosomes to enhance adhesion to Descemet’s membrane. The endothelial layer is nearly 10μm thick at birth. Endothelial cell density continues to change from the twenties to the eighties of life. The cell density of hexagonal cells decreases from 3000 to 4000 cells/ mm2 to about 2600 cells/mm2. They decline nearly from 75% to 60%. Endothelial cell density is about 3500 cells/mm2 at birth. Human endothelial cell density declines by about 0.60% per year in normal corneas during adult life. However, endothelial cells are not renewed in adults.
An another layer of cornea, Dua’s layer, has been noteworthy recently. It is a well-identified, acellular, potent layer without keratocytes in the pre-Descemet’s cornea. This layer has 5-8 thin layer of collagen fibers ranging from 6 to 15μm in thickness. Specular microscopy, fluorophotometry and pachymetry are used for clinical prediction of endothelial cell density and function [3,21,23,27].
Endothelial cells in the cornea have Na+/K+ ATPase pumps that deport sodium ions from cytoplasm to extracellular region. The exported sodium ions pass through the tight junctions into the anterior chamber. Anterior chamber contains also chloride and bicarbonate that are transported here from corneal endothelium via the channels. A part of bicarbonate ions in the anterior chamber is produced by carbonic anhydrase IV from carbon dioxide molecules that come from the interior of the endothelium where they are formed by carbonic anhydrase II. The Sodium, chloride, and bicarbonate in the anterior chamber draw water, forming salts with each other (Figure 6) [28].
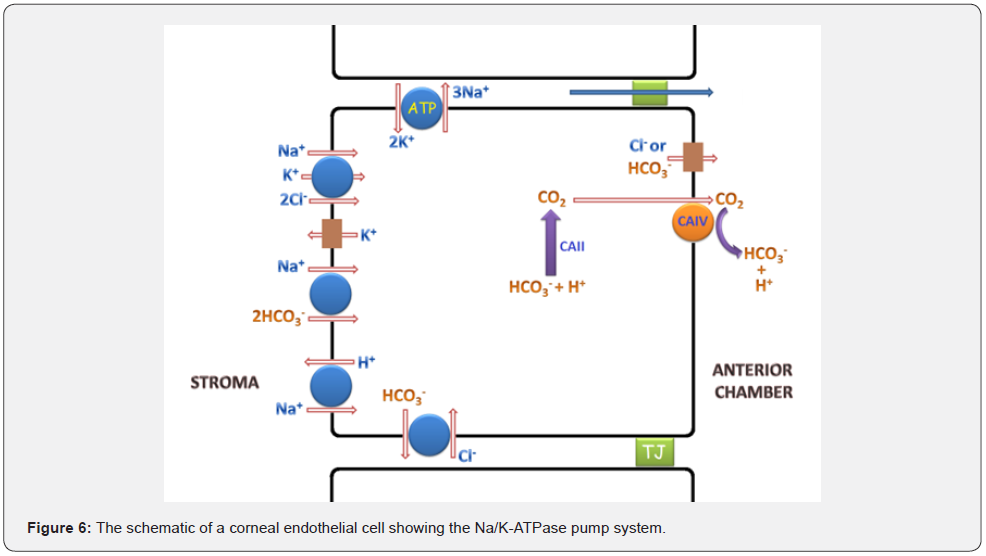
Conclusion
The tear film is the first layer of the cornea and provides lubrication and hydration to the ocular surface. The tear film typically consists of three distinct layers, including lipid, aqueous and mucin. The human cornea is one of the miraculous tissues of the human body. The cornea consists of cellular and non-cellular components. The human cornea consists of epithelium, Bowman membrane, stroma, Dua’s layer, Descemet’s membrane, and endothelium. Each of these layers has its own role to maintain normal visual function. The cornea, a transparent avascular connective tissue, is the most important refractive component of the ocular system together with the overlying tear film. Additionally, thanks to its protective, transparent, and refractive features, it protects the sensitive content of the eyeball and also provides a clear view. A transparent cornea is needed for ocular surgeries as well as for imaging of the anterior chamber, iris, posterior chamber, lens, vitreous, retina, macula, and optic nerve head. However, both experimental and clinical studies are needed for a holistic understanding of the cornea in the future.
References
- DelMonte DW, Kim T (2011) Anatomy and physiology of the cornea. J Cataract Refract Surg 37: 588-598.
- Zhang L, Anderson MC, Liu CY (2017) The role of corneal stroma: A potential nutritional source for the cornea. J Nat Sci 3(8): e428.
- Sridhar MS (2018) Anatomy of cornea and ocular surface. Indian J Ophthalmol 66(2): 190-194.
- Edelhauser HF (2006) The balance between corneal transparency and edema: the Proctor Lecture. Invest Ophthalmol Vis Sci 47(5): 1754-1767.
- Srinivas SP (2010) Dynamic regulation of barrier integrity of the corneal endothelium. Optom Vis Sci 87(4): E239-E254.
- Zavala J, Lopez Jaime GR, Rodriguez Barrientos CA, Valdez-Garcia J (2013) Corneal endothelium: developmental strategies for regeneration. Eye (Lond) 27(5): 5795-5788.
- Rózsa AJ, Beuerman RW (1982) Density and organization of free nerve endings in the corneal epithelium of the rabbit. Pain 14(2): 105-120.
- Rüfer F, Schröder A, Erb C (2005) White-to-white corneal diameter: Normal values in healthy humans obtained with the orbscan II topography system. Cornea 24: 259-261.
- Nishida T (2005) Neurotrophic mediators and corneal wound healing. Ocul Surf 3(4): 194-202.
- Dua HS, Faraj LA, Said DG, Gray T, Lowe J (2013) Human corneal anatomy redefined: a novel pre-Descemet's layer (Dua's layer). Ophthalmology 120(9): 1778-1785.
- Navaratnam J, Utheim TP, Rajasekhar VK, Shahdadfar A (2015) Substrates for Expansion of Corneal Endothelial Cells towards Bioengineering of Human Corneal Endothelium. J Funct Biomater 6(3): 917-945.
- Lwigale PY (2015) Corneal Development: Different Cells from a Common Progenitor. Prog Mol Biol Transl Sci 134: 43-59.
- Astbury N, Ramamurthy B (2006) How to avoid mistakes in biometry. Community Eye Health 19(60): 70-71.
- Fares U, Otri AM, Al-Aqaba MA, Dua HS (2012) Correlation of central and peripheral corneal thickness in healthy corneas. Cont Lens Anterior Eye 35: 39-45.
- Feizi S, Jafarinasab MR, Karimian F, Hasanpour H, Masudi A (2014) Central and peripheral corneal thickness measurement in normal and keratoconic eyes using three corneal pachymeters. J Ophthalmic Vis Res 9(3): 296-304.
- Chen L (2009) Ocular lymphatics: state-of-the-art review. Lymphology 42(2): 66-76.
- Oliveira-Soto L, Efron N (2001) Morphology of corneal nerves using confocal microscopy. Cornea 20(4): 374-378.
- Gipson IK (2007) The ocular surface: The challenge to enable and protect vision: The friedenwald lecture. Invest Ophthalmol Vis Sci 48(10): 4390.
- Meek KM, Boote C (2004) The organization of collagen in the corneal stroma. Exp Eye Res 78(3): 503-512.
- Murphy C, Alvarado J, Juster R, Maglio M (1984) Prenatal and postnatal cellularity of the human corneal endothelium. A quantitative histologic study. Invest Ophthalmol Vis Sci 25(3): 312-322.
- Tuft SJ, Coster DJ (1990) The corneal endothelium. Eye (Lond) 4(Pt 3): 389-424.
- Joyce NC, Meklir B, Joyce SJ, Zieske JD (1996) Cell cycle protein expression and proliferative status in human corneal cells. Invest Ophthalmol Vis Sci 37(4): 645-655.
- Bourne WM, Nelson LR, Hodge DO (1997) Central corneal endothelial cell changes over a ten-year period. Invest Ophthalmol Vis Sci 38(3): 779-782.
- Joyce NC, Harris DL, Mello DM (2002) Mechanisms of mitotic inhibition in corneal endothelium: contact inhibition and TGF-beta2. Invest Ophthalmol Vis Sci 43(7): 2152-2159.
- Tan DT, Dart JK, Holland EJ, Kinoshita S (2012) Corneal transplantation. Lancet 379(9827): 1749-1761.
- Joyce NC (2012) Proliferative capacity of corneal endothelial cells. Exp Eye Res 95(1): 16-23.
- Rio-Cristobal A, Martin R (2014) Corneal assessment technologies: Current status. Surv Ophthalmol 59(6): 599-614.
- Bonanno JA (2012) Molecular mechanisms underlying the corneal endothelial pump. Exp Eye Res 95(1): 2-7.