Nanoparticles for Live Cell Ad In Vivo Applications
Gerardo Byk* and Sarin Palakkal
Department of Chemistry, Bar Ilan University, Israel
Submission: November 28, 2018;Published: December 07, 2018
*Corresponding author: Gerardo Byk, Department of Chemistry, Laboratory of Nano-Biotechnology, Bar Ilan Universit, Ramat Gan 52900, Israel
How to cite this article: Gerardo B, Sarin P. Nanoparticles for Live Cell Ad In Vivo Applications. Glob J Nano. 2018; 4(5): 555649. DOI: 10.19080/GJN.2018.04.555649
Abstract
Functionalizing nanoparticles (NPs) with biological signals is critical for many biomedical and biotechnological applications. While most synthetic monomers allow creating NPs with carefully tuned structures and properties, they lack any biologically recognized functionality, and at best, facilitate non-specific interactions (e.g., protein adsorption) that can be exploited with limited control. Thus, the development of strategies to tether biological signals on biologically compatible nano-metric systems is of great interest. We have developed new NPs well tolerated both in vitro and in vivo with the particularity that peptide synthesis can be carried out on their surface. The platform composed of the NPs and the synthetic peptide is a useful tool for developing imaging methods for intracellular localization of the NPs using microscopy and for in vivo tracking.
Keywords: Self-assembly; Cellular tracking; Thermo-responsive material; Nanoparticle; Magnetic matrix; Solid phase peptide synthesis
Introduction
The main motivation for synthesizing peptides on nanometric supports would be to use them in biological applications while linked to the NPs. The NPs serve as nucleation centers for the targeting ligand by increasing its local concentration, and as flags for tracking bio-relevant ligands exposed on their surface. A platform composed of a NP and a peptide synthesized on its surface, might be exploited for several biological applications such as in vitro/in vivo screening, high throughput screening or in vivo tracking. During the last 20 years, controlled peptide mixtures have been synthesized on macro-particles (beads) using various combinatorial approaches and screened “on-bead”-based in vitro assays for affinity to isolated proteins/antibodies with significant success [1-7]. Nevertheless, these combinatorial systems had only a limited number of applications in live cells screening since the particle carrying the peptide is significantly bigger than cells. Finally, due to the nature and size of the particles carrying the peptides they were excluded from any in vivo application.
The synthesis of peptides on NPs open the nano-metric scale to combinatorial chemistry and allows planning combinatorial screening on live cells or even in vivo. However, the basic condition for any biological application of such platform is the biocompatibility of the NPs. Thus, before embarking on NPs peptide synthesis, it is necessary to generate NPs well suited for peptide synthesis but, at the same time, to be compatible with biological applications.
Biocompatible Nanoparticles for Organic Chemistry
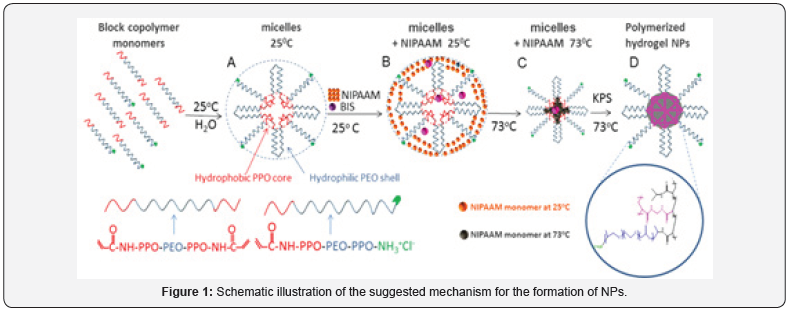
In a recent research [8], we have presented a new type of NPs with varied sizes (from 20 to 400 nm) having the particularity of being generated from very same materials combined in a coreshell like structure composed of a core, rich in poly-N-isopropyl acrylamide- polypropylene oxide (PNIPAAM-PPO), and a shell, rich in polyethylene oxide (PEG) well-known as one of the most biocompatible materials [9,10]. The NPs are obtained by a mechanism where a mixture of monomers and macro-monomers form first self-assembled micelles at high temperature facilitated by the thermo-responsiveness of the monomers mixture. Different ratio of the monomers in the mixtures allows generating micelles of different sizes at high temperature. The presence of an initiator and cross linker freeze the micelles’ structure by radical polymerization producing NPs of varied sizes (see mechanism in Figure 1).
The peculiar composition allows keeping intact the biocompatibility along the different sizes of NPs as proved by their lack of cell toxicity (tested up to 5 mg/ml concentration) and their in vivo compatibility in zebra fish model (see Figure 2).
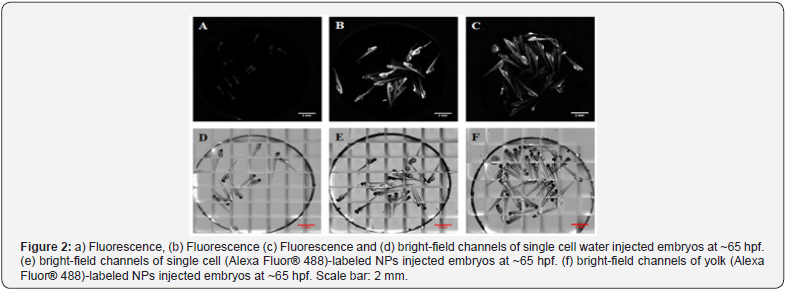
The shell of the NPs bears free amino groups that can be used for labeling or peptide synthesis. Furthermore, the NPs are robustly cross-linked to permit repeated chemical reactions on their surface using organic solvents without promoting their chemical/physical degradation. Fluorescently labeled NPs were injected to zebra fish embryos at one cell stage and observed in real time along the development of the animals. No apparent toxicity could be established: the animals developed normally (see Figure 2) and interestingly, the fluorescent NPs were distributed into different organs in their body (see Figure 3). These results suggest that the NPs can be used for in vivo tracking of stem cells loaded with NPs without significant risk of toxicity.
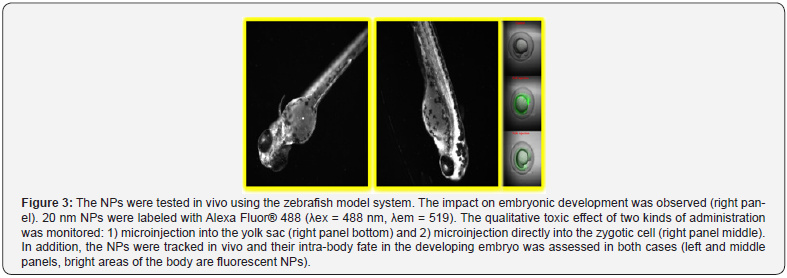
The new NPs can be tracked in live-cell assays using confocal microscopy and their uptake can be quantified (see Figure 4, panels A and B). We have shown that the uptake of fluorescently labeled NPs into cells is inversely proportional to their size (see Figure 4, panel C). The effect is substantial since we observed exponential increments from size to size. On the other hand, the incubation time for a given NP size showed only a linear increase. At this time, we could establish the kinetic tracking-window of the NPs that can be used for tracking ligand-modified NPs in the future. Figure 4 display results calculated from confocal microscopy experiments carried out on PC-3 cells.
A New Method for Peptide Synthesis on Biocompatible Nanoparticles
With these biocompatible NPs in hand it was possible to envision peptide synthesis on their surface. NPs modification techniques are mostly based on the conjugation of a single molecule (protein etc.) by covalent bonding of complementary functional groups found on both components. Because of the difficulty in isolating NPs by filtration/precipitation or dialysis, conjugation is mostly restricted to a single coupling reaction due to the difficulty in removal of excess reagents after reactions. Some multistep reactions have been done using nano-carriers that are soluble in organic solvents under conjugation conditions and precipitate upon washing with protic solvents. In this way, peptides were synthesized on polymers and gold NPs [11,12].
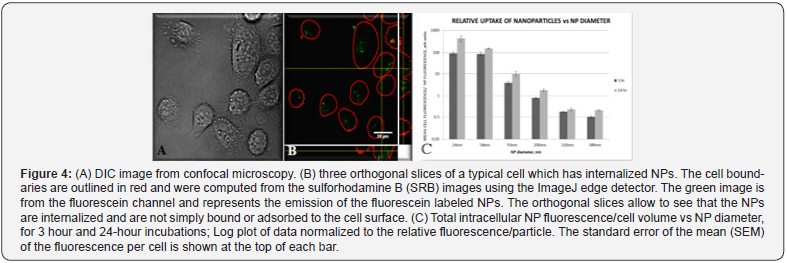
One of the big challenges for NP functionalization is peptide conjugation. Up to now, peptides have been tethered to NPs by conjugating a pre-synthesized peptide with a NP using complementary functional groups that react to form a bridge between the two moieties. We choose to develop a model for full peptide synthesis on NPs as an option to conjugation. Despite to bio and chemical compatibility of these developed NPs, their surface modification by multi-steps reactions remains a difficult task due to their non-aggregative behavior that prevents their precipitation or filtration since they undergo extrusion or merely cross filters. This physical barrier was overcome by a new approach that exploits materials with magnetic susceptibility for easy and fast separation of NPs [13].
While searching for an approach to “trap” NPs, we focused on a known method for fabricating mesoporous inorganic catalysts. With this method, an inorganic material is prepared in the presence of an organic polymer. The obtained product is composed of crystals of the inorganic material in a network that contains a large number of cavities filled with the organic polymer. In the classical method this product is burned at high temperature, resulting in a mesoporous material with a high number of free catalytic cavities previously occupied by the organic polymer [14]. We thought that this approach might be useful for our purpose if the inorganic shell is removed at the end of the process (instead of burning the organic material), leaving the filling organic polymer intact. Thus, the magnetic material is a permeable, external and disposable matrix that embeds and retains the NPs during the multistep peptide synthesis, and then can be removed at the end of the process. The strategy allows the conservation of the biocompatible chemical composition of the NPs, and avoids the biocompatibility limitations associated with previous approaches, where the NPs contained in their structures magnetic material suspected to be toxic [15].
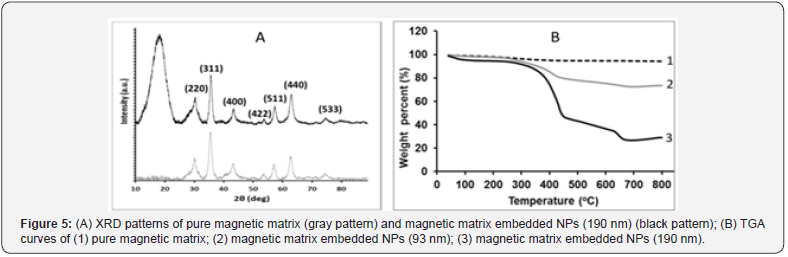
As proof of concept, we described the synthesis of bio-relevant fluorescently labeled model peptides that were fully characterized [13]. This technique is especially interesting because it can be applied to organic/polymeric NPs of different sizes, without utilizing tedious washing methods such as ultracentrifugation or dialysis, which in the past has always been necessary for intermediate steps of reactions on the surface of NPs.
Formation of a magnetic matrix with embedded NPs was accomplished by impregnation of the hydrophilic NPs with a mixture of Fe+2/Fe+3 followed by their co-precipitation in an ammonia solution. This process generated a “matrix” composed of a magnetic network with the NPs “embedded’ within this network. We adapted a method for obtaining super-paramagnetic microspheres, to our system [16]. The obtained matrix can be easily retained by a Nd-Fe-B magnet for repeated washings after reactions. The embedment of the NPs was proved by X-ray diffraction (XRD) where the pure magnetic matrix (grey pattern in Figure 5A) showed the classic fingerprint obtained for a crystalline iron phase. On the other hand, a magnetic matrix with embedded NPs (black pattern in Figure 5A) showed a very broad peak at small angles, known to be generated by amorphous material such as our polymeric NPs that appears together with the diffraction peaks belonging to the crystalline iron oxide phase exactly as in the grey pattern. Both samples produced several relatively strong and slightly broadened reflection peaks in the 2θ region of 20o-80o, quite similar to those of pure-phase maghemite particles reported by other groups, indicating that the particles have mainly the maghemite crystal structure [17].
Direct proof for the presence of the NPs in the magnetic matrix was obtained by thermo-gravimetric analysis (TGA). A pure magnetic matrix (Figure 1) behaved as expected, with a very small weight loss change (~ 5%) over the temperature range of 100-800oC. On the other hand, the magnetic matrix embedded NPs displayed a significant weight loss in the same temperature range (Figure 1). The total weight loss was about 30% for 100 nm and 71% for 190 nm embedded NPs, which represents the relative quantity of NPs within the magnetic matrix.
The overall nanoparticle peptide synthesis (NPPS) process recently published [13] is shown in scheme 1. The method consists of embedding appropriate NPs into a magnetic matrix (step 1), followed by the multistep synthesis of the desired peptides facilitated by the magnetic susceptibility of the magnetic matrix necessary for washings/reactions along the intermediate steps (step 2). Once the synthesis is complete, the matrix is treated with HCl for 6 h and after appropriate neutralization, the NPs are dialyzed once for removal of iron salts (step 3).
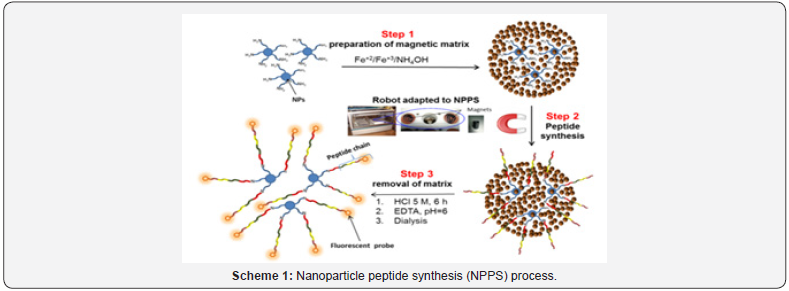
As proof of feasibility, we synthesized a nuclear localization sequence (NLS) peptide from the sv40 large T antigen (PKKKRKV) [18] and a HIV Tat-derived peptide (GRKKRRQRRRPPQ) [19] using NP of various diameters. These peptides have been shown to penetrate into cells and/or localize in the nucleus. In addition, we synthesized a tumour homing pro-apoptotic peptide [KLAKLAK]2 fused to GGKRK (KLA). This peptide has been shown to be specifically targeted to tumours [20]. Cell penetration processes mediated by these peptides, can be observed if fluorescent probes are linked to the peptide-NPs. One of the possible applications for NLS/TAT NPs is the facilitated penetration of NPs loaded with drugs into targeted cells, but they can also be exploited for observing transport to and from the nucleus.
Conclusion
Overall, we have designed and synthesized biocompatible non-toxic NPs and developed a new matrix assisted peptide synthesis method for peptide synthesis on NPs. Embedding the NPs into a magnetic matrix allows multistep peptide synthesis on them using the well-known Merrifield synthetic methodology. The peptide-NPs can be recovered and eventually used “as is” for cell signaling via the molecules synthesized on their surface and appropriate molecular probes. A crucial advantage of the current approach is that magnetic susceptibility for the synthesis on the NPs is mediated by an external, permeable and disposable magnetic matrix that embeds the NPs and can be removed at the end of the multistep process, leaving the polymeric biocompatible NPs intact, with no residual toxic magnetic material. This method might be useful for different types of organic NPs which we are currently testing, and for a variety of applications such those we have recently shown in the field of cell and combinatorial screening [21,22].
Acknowledgment
These works were financed by the Israel Science Foundation grant number 830/11, the Israeli Ministry of Sciences and The Marcus Centre for Medicinal Chemistry of Bar Ilan University. We are indebted to the BIU President Scholarships and to Israel Council for Higher Education for the Converging Technologies Fellowship.
References
- Nallagatla SR, Heuberger B, Haque A, Switzer C (2009) Combinatorial synthesis of thrombin-binding aptamers containing iso-guanine. J Comb Chem 11(3): 364-369.
- Heizmann G, Hildebrand P, Tanner H, Ketterer S, Pansky A, et al. (1999) A combinatorial peptoid library for the identification of novel MSH and GRP/bombesin receptor ligands. J Recept Signal Transduction Res 19(1-4): 449-466.
- Murray JK, Farooqi B, Sadowsky JD, Scalf M, Freund WA, et al. (2005) Efficient Synthesis of a β-Peptide Combinatorial Library with Microwave Irradiation. J Am Chem Soc 127(38): 13271-13280.
- Murray JK, Sadowsky JD, Scalf M, Smith LM, Tomita Y, et al. (2008) Exploration of Structure−Activity Relationships among Foldamer Ligands for a Specific Protein Binding Site via Parallel and Split-and- Mix Library Synthesis. J Comb Chem 10(2): 204-215.
- Kodadek T, Bachhawat-Sikder K (2006) Optimized protocols for the isolation of specific protein-binding peptides or peptoids from combinatorial libraries displayed on beads. Mol Bio Syst 2(1): 25-35.
- Lam KS, Salmon SE, Hersh EM, Hruby VJ, Kazmierski WM, et al. (1991) A new type of synthetic peptide library for identify in ligand-binding activity. Nature 354(6348): 82-84.
- Shin DS, Kim DH, Chung WJ, Lee YS (2005) Combinatorial solid phase peptide synthesis and bioassays. J Biochem Mol Biol 38(5): 517-525.
- Khandadash R, Machtey V, Shainer I, Gottlieb HE, Gothilf Y, et al. (2014) Novel biocompatible hydrogel nanoparticles: generation and sizetuning of nanoparticles by the formation of micelle templates obtained from thermo-responsive monomers mixtures. J Nanopart Res 16: 2796.
- Stadler V, Kirmse R, Beyer M, Breitling F, Ludwig T, et al. (2008) PEGMA/MMA Copolymer Graftings: Generation, Protein Resistance, and a Hydrophobic Domain. Langmuir 24(15): 8151-8157.
- Tessmar JK, Gopferich AM (2007) Customized PEG-derived copolymers for tissue-engineering applications. Macromol Biosci 7(1): 23-39.
- Barth M, Fischer R, Brock R, Rademann J (2005) Reversible crosslinking of hyperbranched polymers: A strategy for the combinatorial decoration of multivalent scaffolds. Angew Chem Int Ed 44(10): 1560- 1563.
- Fan J, Chen S, Gao Y (2003) Coating gold nanoparticles with peptide molecules via a peptide elongation approach. Colloids Surf B 28(2): 199-207.
- Khandadash R, Machtey V, Weiss A, Byk G (2014) Matrix-assisted peptide synthesis on nanoparticles. J Pept Sci 20(9): 675-679.
- Yu BY, Kwak SY (2010) Assembly of magnetite nanocrystals into spherical mesoporous aggregates with a 3-D wormhole-like pore structure. J Mater Chem 20(38): 8320-8328.
- Singh N, Jenkins GJS, Asadi R, Doak SH (2010) Potential toxicity of superparamagnetic iron oxide nanoparticles (SPION). Nano Rev 1: 10.
- Ma Z, Guan Y, Liu HJ (2005) Synthesis and characterization of micronsized monodisperse superparamagnetic polymer particles with amino groups. Polym Sci Part A: Polym Chem 43(15): 3433-3439.
- Hyeon T, Lee SS, Park J, Chung Y, Na HB (2001) Synthesis of highly crystalline and monodisperse maghemite nanocrystallites without a size-selection process. J Am Chem Soc 123(51): 12798-12801.
- Kalderon D, Roberts BL, Richardson WD, Smith AE (1984) A short amino acid sequence able to specify nuclear location. Cell 39(3pt 2): 499-509.
- Vives E, Brodin P, Lebleu B (1997) A truncated HIV-1 Tat protein basic domain rapidly translocates through the plasma membrane and accumulates in the cell nucleus. J Biol Chem 272(25): 16010-16017.
- Agemy L, Friedmann-Morvinski D, Kotamraju VR, Roth L, Sugahara KN, et al. (2011) Targeted nanoparticle enhanced proapoptotic peptide as potential therapy for glioblastoma. Proc Natl Acad Sci USA 108(42): 17450-17455.
- Byk G, Partouche S, Weiss A, Margel S, Khandadash R (2010) Fully synthetic phage-like system for screening mixtures of small molecules in live cells. J Comb Chem 12(3): 332-345.
- Khandadash R, Partouche S, Weiss A, Margel S, Byk G (2011) A fully synthetic “phage-like” system II: synthesis and live cell screening of combinatorial libraries of peptides on sub-cellular sized microspheres. Open Opt J 5: 17-27.