Electro-Thermal Modeling, Aging and Lifetime Estimation of Power Electronic MOSFETs
Cristina Morel* and Nassim Rizoug
Ecole Supérieure des Techniques Aéronautiques et de Construction Automobile, ESTACA’Lab Paris-Saclay, France
Submission: June 26, 2023; Published: July 07, 2023
*Corresponding Author: Cristina Morel, Ecole Supérieure des Techniques Aéronautiques et de Construction Automobile, ESTACA’Lab Paris-Saclay, France
How to cite this article: Cristina M, Nassim R. Electro-Thermal Modeling, Aging and Lifetime Estimation of Power Electronic MOSFETs. Civil Eng Res J. 2023; 14(1): 555879. DOI 10.19080/CERJ.2023.14.555879
Abstract
Power devices are used in various industrial applications, including energy transfer (grid, wind), industrial applications (rail, photovoltaic systems) and civil equipments (electric vehicles, battery charges, home appliance). Power switches reliability may be affected by their high switching frequency, causing strong thermal fluctuations. Therefore, an electro-thermal analysis is essential to improve their reliability and to ensure continuous operation. The device aging phenomenon is taken in consideration for lifetime estimation.
Keywords: Power device; Thermal stress; Junction temperature; Electro-thermal model; Lifetime; Aging
Introduction
Power semiconductor devices, a key part of power converters, switch mode power supplies, integrated power electronics modules and inverters, are their most fragile components. Switching power components are usually distributed regarding their power, application, and frequency operation range, as in Figure 1. Active semiconductor devices, as Si, SiC and GaN MOSFETs, are commonly used because of their high switching speeds. They are also characterized by high temperature and thermal fluctuations, with increasing power density requirements.
An important cause of aging and failures of these devices is their thermal stress ; its reduction in a power device improves its lifetime and reliability. The electro and thermal models are linked together : one communicates its power dissipation Ploss to thermal model and then receives the junction temperature Tj. In order to improve the efficiency and safety and to estimate the remaining lifetime, [1] combines three models : the electro-thermal, the lifetime analytic and the aging models. The operative mode is shown in Figure 2.
In order to generate the complete electrical circuit, the effects of many parasitic parameters (resistor, inductance and capacitor) must be taken into account . In [2], any significant change to simplify the circuit has an impact on the accuracy of power loss. Indeed, power losses in any MOSFET semi-conductor are the sum of the conduction power losses and the switching power turn-on and turn-off losses [3]. These factors contribute to the increase of junction temperature. Conduction power losses depend on the drain-source current, on-state resistance Rds-on, duty cycle and junction temperature. There exists a strong correlation between Tj and Rds-on [4], even if this electrical parameter is assumed constant with reference to the temperature in part of the literature. A typical load mission profile of the electrical model reflects the de-vice operation environment, which generates the correspon-ding loss profile.
The thermal model reproduces the effect of heat propagation inside the device. The model is derived from the thermal impedance of the device datasheet and generates the junction temperature Tj. This temperature is one of the most important parameters impacting the behavior, performance and reliability of the device [5]. Thermal modeling methods include numerical and analytical approaches and the thermal resistor-capacitor network. The finite element method of the numerical approach provides an exact temperature distribution of any device geometry. This method is not applicable for a long-time load because of the very long-time compilation. In [6], the analytical approach provides a Fourier series solution by solving the heat diffusion equations. The most common thermal resistor-capacitor networks used for modeling thermal behavior are Foster and Cauer models [2]. The Cauer model represents the real physical behavior of materials device. Instead, a Foster network is an equivalent resistorcapacitor circuit.
For a single heat source (i.e. a single device), the junction temperature is only determined by its own power losses. Unfortunately, in many studies, the thermal cross-coupling effect is not included. For a multi-chip module, each heat source contributes to increase its own temperature, including thermal coupling between neighboring devices [7]. Then, the thermal model considering the thermal coupling is defined as a thermal impedance matrix. The typical test to induce more failures is the accelerated life-test, which consists in increasing the stress level. The most popular lifetime models are Coffin-Manson, Norris Landzberg and Bayerer’s models [4]. They depend on the stress level and are based on aging accelerated testing data. Thus, the estimated devices lifetime must include an aging process. A lifetime model depends on the following parameters : the number Nf of cycles before the device generates a fault under thermal stress, junction and mean temperatures. To count the thermal cycles, the half-cycle thermal [1], the maximum-edge and the rainflow counting algorithms can be used to estimate the accumulated stress and aging of the devices.
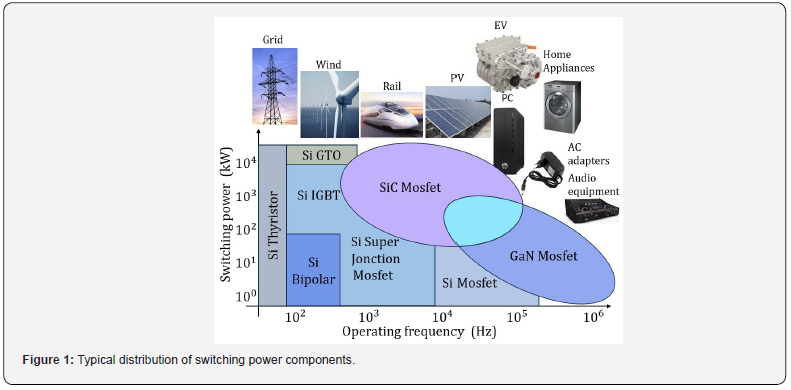
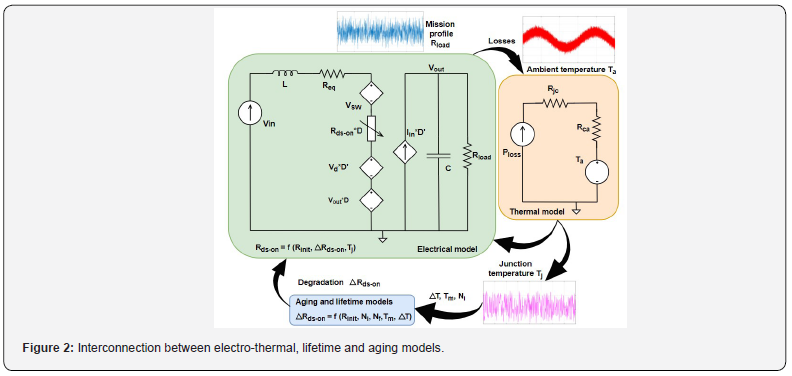
Coffin-Manson model is based on the assumption that the failures are accelerated by the stress difference and not by the rapidity of this difference. But, Coffin-Manson model does not distinguish test temperature profiles with the same ΔT. To determine the number of cycles-to-failure, the Norris-Landzberg model takes in account the temperature change. The failure root causes in power semiconductor MOSFET include junction temperature swing and mean junction temperature modelling with Coffin-Manson-Arrhenius and Monkman-Grant. Therefore, the mission profile must use these temperatures through the power loss, electrical and thermal modeling. Three aging models exist in the literature : the exponential degradation model developed by [8], the modified exponen-tial model proposed by [1] and the stress related model [9]. From thermal and aging point of view, the dominant affected parameter of MOSFET devices is Rdson , which evolution enables the evaluation of the device lifetime. The life-cycle of power semiconductors devices mush be provided by a re-liability over a period of many years.
Conclusion
Electro-thermal models, together with lifetime and aging models are used to evaluate stress accumulation and degradation evolution. The accumulated stress on device accelerates the degradation process for a long mission profile load. Thus, it is important to include the aging effect into the estimated lifetime model.
References
- Cheng T, Lu D, Siwakoti Y (2013) A Mosfet Spice model with integrated electro-thermal averaged modeling, aging, and lifetime estimation. IEEE Access 9.
- Raciti A, Cristaldi D, Greco G, Vinci G, Bazzano G (2015) Electrothermal PSpice modeling and simulation of power modules. IEEE Trans. on Ind. Electronics 62: 6260-6271.
- Urkizu J, Mazura M, Alacano A, Aizpuru I, Chakraborty S, et al. (2019) Electric vehicle inverter electro-thermal models oriented to simulation speed and accuracy multi-objective targets. Energies 12: 3608.
- Chen H, Lin S, Liu Y (2021) Transient electro-thermal coupled modeling of three-phase power MOSFET inverter during load cycles. Materials 14(18): 5427.
- Kishor Y, Patel R (2022) Thermal modeling and reliability analysis of recently introduced high gain converters for PV application. Cleaner Energy Systems 3: 100016.
- Nejadpak A, Mohammed O (2013) A physic-based dynamic electro-thermal model of silicon carbide power IGBT devices. IEEE Applied Power Electronics Conference, pp. 201-206.
- Karami M, Li T, Tallam R, Cuzner R (2021) Thermal Characterization of SiC Modules for Variable Frequency Drives. IEEE Open Journal of Power Electronics 2: 336-345.
- Dusmez S, Akin B (2015) An accelerated thermal aging platform to monitor fault precursor on-state resistance. Proc. IEEE Int. Electric Mach. Drives Confernce (IEMDC), ppp. 1352-1358.
- Testa A, De Caro S, Russo S (2012) A reliability model for power MOSFETs working in avalanche mode based on an experimental temperature distribution analysis. IEEE Trans. Power Electron 27: 3093-3100.