Applicability and Limitations of Thermoplastic Composites for Hydrogen Pressure Vessels
Zoltan Major*
Institute of Polymer Product Engineering, Johannes Kepler University Linz, Austria
Submission: January 23, 2023; Published: January 30, 2023
*Corresponding author: Zoltan Major, Institute of Polymer Product Engineering, Johannes Kepler University Linz, Austria
How to cite this article: Zoltan M. Applicability and Limitations of Thermoplastic Composites for Hydrogen Pressure Vessels. Academ J Polym Sci. 2023; 5(5): 555675. DOI: 10.19080/AJOP.2023.05.555675
Keywords: Thermoplastic; Composites; Hydrogen; Pressure Vessels; Thermoset
Opinion
High potential of thermoplastic composites (TPCs) for demanding applications is expected in many publications and technical documents for aircraft, automotive and energy sector [1-3]. The driving forces behind the increasing application of thermoplastic composites are expectations about (i) the easier/better recyclability, (ii) significantly higher productivity and cost efficiency in both low/medium (aircraft) and high batch production (automotive applications, storage tanks) and (iii) the improved toughness of TPCs due to the ductility of the various thermoplastic polymer matrices compared with conventional thermoset matrix materials [2-4]. All above three aspects should be analyzed in detail using realistic scenarios and proper data for a successful overall product and process development procedure for thermoplastic composites. In addition to the conventional engineering approach for the product and process design a novel ecological analysis based on a realistic, multi-scenario and quantitative life cycle analysis is also essential. Without the cooperation of engineering and ecological life cycle analysis experts there is a high potential for a dead-end street story of thermoplastic composites. Furthermore, the complex material behavior of TPCs influences the design and the sizing of the components. Hence, for a reliable dimensioning, proper material properties have to be transformed into corresponding material models in order to perform proper simulations for reliably determining stress-strain distributions in components. The identification of critical loading path, potential damage regions and local stress/strain values is essential for reliable component design. These stress/strain values are than compared with proper strength parameters (intra and interlaminar) using appropriate strength hypotheses for composites which considers the anisotropy [5,6].
The experimental determination of proper mechanical properties over a wide temperature and loading rate range is a challenging task. The low (below -20 °C) and high temperatures (above 200 °C) pose complexities both from measurement and test technique point of view even at moderate loading rates. Especially, the experimental characterization at high loading rate requires a number of additional considerations for both the test set-up to the data reduction. Furthermore, as thermoplastic composites are often exposed to cyclic loading conditions, the proper characterization of the fatigue behavior and the estimation procedure for life time plays an important role. There is no perfect agreement in the literature about the test methods and data reduction procedure necessary. However, due to the limited expressiveness of laboratory specimens data, appropriate component test are also needed. To reduce the testing duration times and to increase the reliability, accelerated or highly accelerated life time estimation protocols (ALT and HALT) should also employed. A potential application for thermoplastic composite materials towards green economy is pressure vessels for hydrogen. Hydrogen could be a key cornerstone of the green transformation of the global economy, and a major lever to diversify energy supplies and accelerate the clean energy transition. The various methods for green hydrogen production from renewable resources become high attention both in the scientific research and in the industrial development. Green hydrogen is produced through water electrolysis process by employing renewable electricity of solar, wind, hydro and geothermal energy.
Hydrogen is already being used in various applications in the transport, electricity and heat sectors. Numerous current research and demonstration projects clear that hydrogen can be used as a fuel, as a fuel and as a storage medium for renewable electricity [5,7]. In addition to the higher efficiency compared to the hydrogen combustion engine, use in the fuel cell has the advantage that there are no other emissions apart from water. There is a worldwide standard for refueling and storing hydrogen as compressed gas, which usually provides for 350 bar systems for buses, heavy goods vehicles and rail vehicles. 700 bar systems are mainly used in passenger cars and applications in which a higher energy density must be achieved at the storage system level due to the limited installation space. Storage technologies for the energy utilization of hydrogen are classified into physicalbased and material-based storage forms. The physical storage can be divided into compressed gas, cryo-compressed and liquid gas storage techniques.
The following 4 types of high-pressure vessels are classified for above storage techniques:
i. Type I: pressure vessel made of metal
ii. Type II: pressure vessel made of a thick metallic liner hoop wrapped with a fiber-resin composite.
iii. Type III: pressure vessel made of a metallic liner fullywrapped with a fiber-resin composite.
iv. Type IV: pressure vessel made of polymeric liner fullywrapped with a fiber-resin composite.
The pressure vessels are generally cylinders, but composite vessels can also be polymorph or toroid [3,5]. Recently, in spite of the higher material and production costs Type IV pressure vessels become more attention. The figure shows a simplified classification of the sub-types of grade IV pressure vessels (Figure 1). In addition to the conventional thermoset resin matrix materials (epoxy or polyester), several thermoplastic polymers (polypropylene, polyamide, polyether ether ketone) have been used as matrix for thermoplastic composites. Due to the high level of mechanical loads the application of high-stiffness carbon fibers is requested. These pressure vessels are exposed to a complex combination of mechanical and thermal loads during the operations. The periodic filling and refilling of the vessels at increasing and decreasing temperatures generates in and out-ofphase thermomechanical loading and reloading cycles in the wall and in the connector part of the pressure vessel. Furthermore, due to the small size of the H2 molecule, the diffusion and thus the permeation are enhanced. The permeation is specific of type IV vessels and is an inherent phenomenon for all gases in contact with polymers. It is the result of the H2 gas dissolution and diffusion in the polymer matrix.
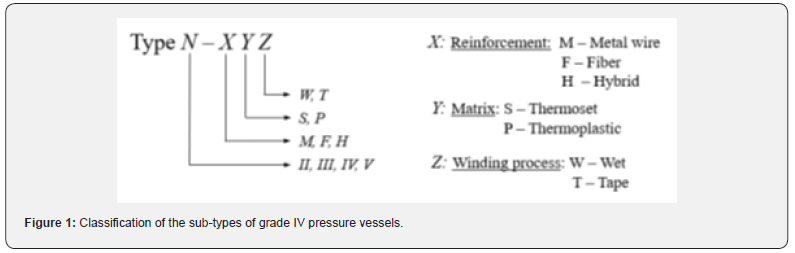
Laser-assisted tape winding (LATW) is an automated manufacturing process to produce fiber-reinforced thermoplastic composites with high strength-to-weight ratio [2,3]. The final product quality and performance are highly dependent on the quality of the fiber reinforced tapes, the process temperature together with the speed, consolidation force, and surface preparation. While the intralaminar strength and fatigue strength depends on the tape quality the interlaminar strength and fatigue strength highly depend on the quality of the consolidation. The intensive application of hydrogen to the green transformation of the global economy make sense if the majority (all) parts of the supply chain are fully recyclable in a circular technology process. Hybrid or composite materials reveal many advantages for performance and functionality of various components but are not advantageous from recycling point of view. Although, generally polymeric materials can simultaneously fulfill both structure mechanical and functional requirements, it is not easy to find polymer system with outstanding mechanical properties (stiffness and strength) and permeation resistance. Future development should focus on the integration of both functions in one material system.
References
- Jin Z, Han Z, Chang C, Sun S, Hongya Fu (2022) Review of methods for enhancing interlaminar mechanical properties of fiber-reinforced thermoplastic composites: Interfacial modification, nano-filling and forming technology. Composites Science and Technology 228: 109660.
- Boon YD, Joshi SC, Bhudolia SK (2021) Review: Filament Winding and Automated Fiber Placement with In Situ Consolidation for Fiber Reinforced Thermoplastic Polymer Composites. Polymers 13(12): 1951.
- Hosseini SMA, Schäkel M, Baran I, et al. (2018) A new global kinematic-optical-thermal process model for laser-assisted tape winding with an application to helical-wound pressure vessel. Materials & Design 193: 108854.
- Ma LL, Liu F, Liu D, Liu Y (2021) Review of Strain Rate Effects of Fiber-Reinforced Polymer Composites. Polymers 13(17): 2839.
- Alves MP, Gul W, Cimini Junior CA, Ha SK (2022) A Review on Industrial Perspectives and Challenges on Material, Manufacturing, Design and Development of Compressed Hydrogen Storage Tanks for the Transportation Sector. Energies 15(14): 5152.
- Takemoto S, Yoshikawa N (2022) Strength evaluation of CFRP structure of high pressure hydrogen tank based on mesoscale analysis. Materials Today Communications 32: 103966.
- Barral K, Barthélémy H (2006) WHEC 16 / 13-16, Lyon France.