Sensor-based and Robot Sorting Processes and their Role in Achieving European Recycling Goals - A Review
Friedrich Karl*, Koinig Gerald, Fritz Theresa, Pomberger Roland and Vollprecht Daniel
Chair of Waste Processing Technology and Waste Management, Department of Environmental and Energy Process Engineering, Montanuniversitaet Leoben, Austria
Submission:January 18, 2022; Published: February 14, 2022
*Corresponding author: Karl Friedrich, Chair of Waste Processing Technology and Waste Management, Department of Environmental and Energy Process Engineering, Montanuniversitaet Leoben, Franz Josef-Straße 18, 8700 Leoben, Austria.
How to cite this article: Friedrich K, Koinig G, Fritz T, Pomberger R, Vollprecht D. Sensor-based and Robot Sorting Processes and their Role in Achieving European Recycling Goals - A Review. Academ J Polym Sci. 2022; 5(4): 555668. DOI: 10.19080/AJOP.2022.05.555668
Abstract
A circular economy is the stated aim of current technological and political developments in the waste management sector. Achieving the goal of a circular economy requires significant improvements in waste treatment technologies. For this reason, this paper summarises the relevant technologies, detailing the developments in the significant sensor-based sorting technologies. This review analyses the key spectral analysis methods like Near-Infrared Spectroscopy, Visual Spectroscopy, X-ray transmission, X-ray fluorescence analysis and Laser-Induced Breakdown Spectroscopy. This study further contains a detailed analysis of the standard sensor-based sorting construction types chute sorter, belt sorter and robot-aided sorting. Further insights in the branch of sensor-based sorting are permitted by describing the key players and stakeholders in sensor-based sorting, detailing the area of expertise and current fields of study for primary sensor and sorting machine suppliers. A convenient lookup table detailing the capabilities of these significant suppliers is provided. The last chapter summarises relevant trends and developments in digitalisation and Industry 4.0 in the waste and recycling sector, elaborating on relevant technology like digital waste management, sorting robots in waste management, smart villages and recyclable materials scanners. The reviewed data portrays the waste management industry’s substantial developments. While new technologies, like machine learning, convolutional neural networks and robot sorting, are increasingly implemented, a substantial discrepancy exists between technological capabilities and the current State-of-the-Art.
Keywords: Sensor-based Sorting; Robot Sorting; Recycling Goals; Digitalisation; Circular Economy
Introduction
This study aims to research developments in sensor-based sorting and robotics and their effects on waste management. The implementation and further development of sensor-based sorting and robotics has great potential to change waste management over the long term. In addition, research is performed on the technologies currently available on the market to determine their future potential. Furthermore, possible solutions are derived from achieving the circular economy package’s new European resource efficiency targets. Developments in waste technology and management are based on solving technical problems within the given legal framework. In the following, the essential regulations guide the waste management sector, cause trends and significantly influence their developments by changing parameters.
Circular Economy Package
The Circular Economy Action Plan of the European Union (EU) was introduced in December 2015 and it is intended to lead to a more resource-efficient future. In Europe, there has been a continuous growth of recycled materials to total raw materials from 2008 to 2016. However, secondary raw materials only account for 12 % of the total demand for raw materials in the EU, which provides a broad basis for innovation in waste management. A new legal basis came into force in July 2018, requiring, among other things, recycling rates of 70 % for packaging waste by 2030 and 65 % for municipal waste by 2035. In addition, the landfilling of municipal waste hass to be reduced to 10 % [1]. Furthermore, the harmonisation of definitions and calculation methods for recycling rates and new requirements for the separate collection of the waste types defined in the Waste Framework Directive (WFD) are included. These new regulations strengthen the market for secondary raw materials and create a uniform system to ensure their quality and make them comparable. The basis for this is always the most efficient waste separation and sorting possible [1]. Central objective is increasing the recycling quota by overcoming the plethora of challenges opposing this development [2].
Plastics strategy
The strategy for plastics, adopted in 2018, states that from 2020 onwards, all plastic packaging on the EU market must be recyclable and the consumption of single-use plastics should be reduced. The EU states that about 150,000 to 500,000 tonnes of plastic waste ends up in the sea every year. In order to prevent this, the plastics strategy aims at setting the path towards a circular economy of plastics. An important factor is the cost efficiency of recycling plastic waste, which can be achieved through changes in production and the design of packaging and products. Close cooperation between packaging manufacturers and the recycling industry as well as communication between the waste management and chemical industries is required to find a broader field of applications for recycled packaging waste. The EU expects a fourfold increase in demand for recycled plastics when the Plastics Strategy is fully implemented, which reduces the dependence on imported fossil raw materials. The resulting carbon dioxide (CO2) savings are expected to help meet the targets of the Paris Agreement [3].
For the waste management sector, this means opportunities for innovation, growth and new business models based on the circular economy. The expected increase in the market value of recycled plastics is based on evaluations of the automotive and construction industries, and economic incentives from the EU are also currently under discussion. Furthermore, increasing volume and better separate collection are expected to make recycling more lucrative. In order to achieve these goals, investments in infrastructure and innovation, which the EU estimates at 8,4 to 16,6 billion Euros, are needed. The strategy of plastics as an ambitious vision can become a job provider if the main actors take concrete actions towards a circular economy (“Towards a Circular Economy”) [3].
Single-Use Plastics Directive
The directive on reducing the impact of certain plastic products on the environment was designated as part of the Plastics Strategy only eight months after it was presented and came into force on 2 July 2019. The directive is based on a census that traced the pollution of European beaches to 15 products. According to litter counts, the main component of marine litter is plastics, at around 80-85 %, and these consist of 50 % single-use plastic and 27 % fishing gear. The single-use plastic problem can also be illustrated very well by measured numerical data. Between 1950 and 2015, more than one tonne of plastic was produced per capita of the world’s population, of which not even ten per cent was recycled. Half of the plastic ever produced worldwide has been produced since 2000. The market restrictions imposed by this directive mainly affect single-use plastic items. Unlike biobased and biodegradable plastics, this directive does not cover microplastics, glass and metal beverage containers. The aim is to reverse the trend, as consumption is expected to increase from single use items to more sustainable alternative items. The member states should set as ambitious measures as possible to comply with the waste hierarchy. It is also essential to consider the product life cycle and a harmonised standard in product design, which the waste management industry has demanded for a long time. In addition to increased producer responsibility, consumer decisions are also to be steered in a more sustainable direction to achieve a measurable quantitative reduction in the consumption of single-use plastics in the EU by 2026 compared to 2022. The directive focuses on marketing restrictions, product requirements, labelling requirements, extended producer responsibility, separate collection and consumer awareness measures. Further specifications concern the coordination of measures, guidelines on single-use plastic articles, information systems and reporting and finally, sanctions as well as evaluation and review. In principle, the directive’s contents must be complied with by 3 July 2021, although individual articles will not come into force until later. Market restrictions on products will come into force by the end of 2024 and the increased recycled content in beverage bottles by 2030 [4,5].
Sensor-based sorting as a key player
Developing a sustainable circular economy would be unthinkable without sensor-based sorting technology, especially if the ambitious EU targets shall be achieved. For example, solutions already exist for almost all industrial waste sorting tasks in polymer materials, which the actors in the recycling chain have also adopted [6].
Sensor-based sorting: Sensor technologies
Sensor-based sorting technology can automatically sort materials according to various material properties to divide the material flow into different product groups [6]. The upswing in sensor-based sorting is due to the rapid development in noncontact sorting technology, which has opened up new areas of application in recent years. This development, which continues to be dynamic, leads more efficient devices and thus to new areas of application in waste management [7]. Due to the increasingly complex requirements for the quality of the end products, the more valuable fractions and higher recovery of these fractions’ materials, sensors with different measuring principles are being combined more frequently to meet the prevailing trends in this direction [8]. The complexity of the technical design and the number of sensors is decisive for the possible applications. Especially in dry sorting, this technology has led to redesigns of processing methods and new application possibilities. Comparisons with the still widespread manual sorting showed that human eyesight was often insufficient to identify the properties of waste components and that machine systems were more efficient in this respect [9].
The learning ability of modern sensor-based systems, which is achieved through software-controlled data processing, is seen as an unique advantage, especially in changing the feed material’s composition or quality requirements. The decoupling of the sorting criterion from the actual separation process reduces the risk of false outputs due to mutual interference and the carry-over of different components. The units are very compact and can also be used in mobile plants. In addition, materials can be separated, which would otherwise be impossible to separate, such as minerals of the same density and magnetic properties in the coarse range. In addition, multiple sorting criteria like e.g. wettability or conductivity can be used in one process stage to achieve better separation efficiency. Due to considerable savings in water, energy and reagents compared to other separation methods, non-contact sorting can make the recovery of previously uneconomic deposits economical. Possible pre-enrichment by sensor technology also helps, which saves resources and, since it can already be used onsite, also space and transport costs [9,10].
However, good separation results can only be achieved by suitable preconditioning of the feed material. Pre-classification to the narrowest possible particle size range and separation of fine and coarse material that cannot be sorted is essential for achieving a certain separation accuracy, depending on the performance of the sorting system. The material mixture to be processed must be pre-treated so that the concentration of the material to be separated is as high as possible. At the same time, disturbing components such as easy dispersible particles with low densities are separated beforehand. Particularly important in preparing the feed material is separating the particles to create at least a monolayer. This means that the individual particles do neither touch nor overlap each other. Some sensor types require clean surfaces, which are generally produced by a washing process. This requirement leads to a certain amount of water consumption in the dry technique, which is less than wet separation methods. In addition to very light, flyable materials, composites and agglomerates also present particular challenges [9,10]. In principle, it is possible to use all non-contact physical measurement methods as separation methods. Factors such as resolution, measuring speed and environmental influences determine the possible applications, and so there is still potential for optimisation even with the sensor types already in use [10].
Sensors are differentiated according to whether they can detect superficial properties or “look inside” the material. The essential types belong to the former group and include the optical (colour) line scan cameras, which measure colour, brightness, transparency, reflection and shape. Fluorescent materials can also be detected after UV excitation [10]. 3D sensors, which function via laser triangulation, can consider the shape and structure of the material [6]. The wavelengths of the sensors, which are installed for sensor-based sorting, explain different fields of application. The terahertz range in the electromagnetic spectrum, as shown in Figure 1, is a part that is not yet fully exploited [11]. The sensors’ non-contact detection of object properties and characteristics consists of an object feeder, a separation system, and intelligent sensor technology consisting of an emitter, a detector, an evaluation and a discharge unit [12]. Table 1 presents an overview of the typical sensor types installed in waste management [13]. Near-infrared spectroscopy (NIR) for detecting material properties works via a light source placed above the conveyor belt that irradiates the material with infrared light. The irradiated molecules are excited to vibrate by specific wavelengths corresponding to the resonance frequency and reflect the other wavelengths diffusely. The respective spectrum is compared with a database and each one is assigned a material class as shown in Figure 2; this is called classification. The absorption lines important for plastic recognition are between 1,200 and 2,000 nm [14]. Spectroscopy works analogously to NIR spectroscopy in the visual (VIS) frequency range for colour sorting. Digital images are assigned different numerical values per pixel, exactly one for grey-scale images and three numerical values per pixel (red, green, blue) for colour images. In contrast to these red-green-blue (RGB) cameras, several hundred numerical values are assigned to a pixel in hyperspectral imaging (HSI) [15].



The spectral decomposition of the signal happens before the detector and results in a complete spectrum for each pixel. However, the passband wavebands of an RGB colour camera are much wider for the three colours red, green and blue. Combining both principles, the entire wavelength range from 400 to 1,000 nm can be covered, in that the HSI camera can represent parts of the VIS and the NIR range, and the RGB camera can cover the VIS range from 400 nm to 700 nm, at least in three bands. Figure 3 shows the transmittance curves of an RGB colour camera equipped with a filter for wavelengths above 650 nm and the HSI camera equipped with a passband of a bandpass from 600 to 1,000 nm to avoid ambiguous information [15]. Typical HSI cameras can operate between 250 to a maximum of 2500; an example for waste management imaging is shown in Figure 4 [15,16]. Hard plastics, paper, films, wood, biomass or fuels are separated with this method. In order to achieve the quality requirements for higher-quality recycling, multi-stage sorting is more frequently used than positive and negative sorting combinations. In positive sorting, the recyclable material is enriched in the discharged product, and in negative sorting, interfering components are separated. By switching between these two types of sorting, it is possible to react to the waste sector’s often highly fluctuating input compositions. NIR or VIS spectroscopy are increasingly used in commercial waste, electrical and electronic scrap, bulky waste, biowaste and mineral waste. The secondary raw materials industry is a significant development driver, demanding innovations with more complex sorting requirements and higher quality standards [8,9].


The second large group is electromagnetic sensors. These sensors are located transversely to the belt’s direction under the conveyor belt. Each generates a high-frequency electromagnetic field through a coil, which is changed by introducing conductive materials. The coil induces eddy currents in the material, which extract energy from the emitting field. The energy is detected by the electromagnetic sensor and leads to metal detection. For this reason, electromagnetic sensors are often used in shredder plants and electronic scrap processing. After using classic metal separators such as magnetic separators and eddy current separators, up to 10% of metals can still be contained in the material stream. These can be recovered with additional sensors. It is also helpful for sorting construction waste or incineration ashes, especially with digital image processing. In addition to metal recovery, purification of metallic fractions is also possible, for example, in PET bottle sorting [8].
The third large group of sensor types is relevant for recycling work with X-ray radiation. Here, a distinction is made between X-ray transmission and X-ray fluorescence.
X-ray transmission (XRT) divides the material according to density differences by measuring the degree of absorption of the X-ray radiation. The degree of absorption depends on the thickness and density of the material. The influences of the material size and thickness are compensated to determine the material-specific absorption of the individual parts with the help of software. For this purpose, the X-ray source is placed below the material flow and the scanner area for determining the residual radiation is placed above. Based on the information from the scanner and the sorting specifications, two products result [6].
X-ray fluorescence analysis (XRF) divides the material stream based on its atomic composition. The scanner unit and the X-ray source sit above the material stream, passing through a lowenergy X-ray field. The chemical elements are excited to emit element-specific energy by shell jumps of the electrons. The evaluation software outputs energy spectra that can be used to separate different product groups [6].
The use of laser technology is suitable for cleaning compost/ structural material by removing glass and plastics. Due to the ‘scattering’ effect, which occurs depending on the degree of hardness and water content, laser technology is particularly applicable in this area. Depending on the wavelength range of the laser, different properties such as colour, structure or, for example, fluorescence can be detected together or separately [8].
LIBS is shorthand for Laser-Induced Breakdown Spectroscopy, and this technology is used to determine the elemental composition of the specimen. LIBS uses high-focused light amplification by stimulated emission of radiation (laser) to remove the surface of the specimen [12].
It causes the electronic excitation of atoms, which form a plasma. As these fall decay back into their original state, they emit light of specific wavelengths. These wavelengths are characteristic of the element composition in question, forming a “fingerprint” used for qualitative and quantitative evaluation. The detection technology in collecting waste is less widespread and less researched than sorting waste. Reasons for this are the high decentralisation of waste accumulation and the influences of weather, vibrations, and dirt. An example of a waste stream that requires high purity for recycling is biowaste for composting plants. The German company Maier & Fabris has developed a metallic value or contaminant detection system based on eddy current induction directly on the collection vehicle. Further development is an automatic feedback system for citizens to inform them directly about the analysis result and, in the worst case, to block emptying at the collection vehicle. In addition to imaging techniques, research is currently being done on a detection method for odour, using ‘electronic noses’. Although significant progress can be seen, this technology is not yet employed commercially. However, data generation has new possibilities, such as weighing waste bins [12].
Sensor-based sorting: Construction type
Generally, a distinction can be made between the two systems of material feeding, namely feeding by chute and by belt. Both types are used in recycling and they differ according to their material feed. As shown in Figure 5, the chute machines are used mainly in the fine-grain range and with bulk materials that flow well. An oscillating conveyor trough (A) ensures uniform distribution over the entire width of the conveying chute and sufficient separation. The feed is then transferred to an inclined chute for further separation and acceleration. A detection device (B) inspects the feed material below the chute by a detection device (B) in free fall.
With the help of a computer, a real-time image of the material flow is classified according to various properties such as colour information, position and size. This image activates compressed air valves of a nozzle bar (C), which discharge the detected components (D). This type of construction is often combined with a colour line scan camera with an associated illumination unit and can distinguish colours in a vast spectrum [9]. With two or three sorting paths, the chute system is designed for the raw materials industry and the recycling industry to sort used glass, plastics, used electrical appliances, incineration ash, and construction and demolition waste [9].
As exemplified in Figure 6, belt sorters were developed for coarse and irregular feed material and can be used in combination with a NIR wavelength range detector. The feed material is again fed via an oscillating conveyor trough (A) and is pre-collected by continuously increasing conveyor speeds. Above the belt conveyor is an NIR sensor (B) which monitors the entire belt width and compares the characteristic spectrum of the objects with those in a computer database. Classification is also done according to size and position. The actual sorting is done using an air nozzle bar (C), which targets and separates the object (D) under investigation with one or more nozzles [9]. The belt sorting systems are used in the recycling industry to sort paper, plastics, RDF, and household and commercial waste with two sorting paths [17]. Various companies, also offer multiway sorting systems, which provide up to six sorting paths. Areas of application are packaging waste from household and commercial waste [17].
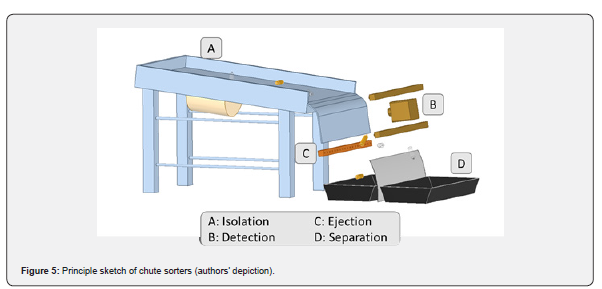

Robotics
The Robotic Industries Association (RIA) defines robots as follows (Inc 2020): “A robot is a reprogrammable, multifunctional manipulator designed to move material, parts, tools or specialised devices through variable programmed motions for the performance of a variety of tasks. Recently, however, the industry’s current working definition of a robot has come to be understood as any piece of equipment that has three or more degrees of movement or freedom.” [18]. Many industries have been using robotics and automated work processes for many years to take over physically demanding tasks from humans and make processes more efficient and more manageable. Especially in the industrial sector, collaborative robots, i.e. robots built to work with and alongside humans, are becoming increasingly important [19]. Human-robot collaborations refer to humans and machines working simultaneously on the same object, and cooperation refers to working reciprocally. Without the protective concepts such as nets or grids, it requires a unique design of the robot arms, which do not have sharp edges and rigid material. This concept combines the hand-eye coordination, force dosage and independent problem-solving ability of humans with the advantages of robotics such as freedom from fatigue, path fidelity and precision [20]. Table 2 shows a classification of common robot types including their functions [21].

Roughly categorised, industrial robots can take on tasks in the areas of production (robot carries tool), assembly and handling (robot carries gripping system), as well as checking and measuring (robot carries measuring device). They consist of arm parts connected by joints and can vary in size and number depending on the type of application. The entire robot arm is referred to as the manipulator, the foremost part as the effector, to which a wide variety of tools and grippers can be attached. Most systems for waste management fall under mechanical separators using grippers (pneumatically, electrically or hydraulically controlled), use suction pads or vacuum cups. The former robotic systems sort, for example, construction waste and the latter are used for sorting packaging. An important part is the control system, through which connected sensors can also be used in some circumstances. The robotic system also includes safety devices if needed, for example, to protect labourers. The kinematics (spatial relationship between the workpiece or tool and the manufacturing device) determines the design of the robot, which influences the working area, the load-bearing capacity, speed and repeatability [22].
Industrial robots usually have six degrees of freedom, which allow them to grasp objects independently of their positioning. The term kinematics describes the movement axes resulting from the degrees of freedom. Two types of kinematics are distinguished: serial kinematics, where the robot arm gets its mobility from joints but is connected to the base at one point (drives in the joints are also moved), and parallel kinematics, where several arms are connected to a fixed drive and can be moved simultaneously. Depending on the task, fewer degrees of freedom can be achieved using rotational, linear and translational joints, leading to the goal. The joints determine the operating range, shown in Figure 7 [22,23]. According to the kinematics, the following classification of industrial robots in Figure 8 is suggested.
According to the IFR - International Federation of Robotics statistics, an average of 106 new robots were installed per 10,000 employees in Europe in 2017. The record year 2017 saw a 30 % increase worldwide compared to the previous year [25]. One reason for the increased use of robotics is undoubtedly the rapid development in artificial intelligence, i.e. the ability of robots to perform so-called ‘deep learning’. Deep learning uses a particular type of information processing that functions via artificial neural networks. Using artificial neural networks and large amounts of data, machines can imitate the human brain in its decision-making processes and thus independently improve their abilities without human assistance. The more data is available and combined with the progress already made, the more complex the problems and the machine’s approaches to solving them. The use of robotics has limitations in that material can be heterogeneous, dirty and have different properties such as structure, size and shape. Limitations of the technique include non-optimised material flow, position changes between detection and gripping of the robot, poor presorting, sensor failures, and limitations of the robotic arm such as size, reaction speed and the number of parts selected per hour [19].
Challenges for the use of robotic systems are also currently still unclear legal obligations in the event of damage. In some cases, existing laws, such as waste management laws, further hinder the progress of digitalisation. Lack of acceptance, for example, due to quality problems, is not to be expected because of the rapidly developing technology [26]. The fear of job losses can be calmed by the fact that robotics is accompanied by a reduction of stresses and dangers in manufacturing and that new professions are constantly being created in automation and data processing. At present, it is mainly menial labour, or tasks that are monotonous, heavy or hazardous to health that have been lost [22].

Materials and Method
In order to achieve the objectives of this study, several methods described below were used to provide the most comprehensive possible picture of waste management and its future developments. The scope of this study ranges from the description of the legal and technological framework conditions to a comprehensive analysis of future technologies and trend developments.
projects in waste management
After extensive research on the most crucial technology, sensor-based and robot sorting suppliers are found and divided into the categories sensor suppliers, sorting machine manufacturers, and sorting robot manufacturers. In addition to the descriptions of the interest groups, some current projects in the field of sensor-based sorting and robotics are presented.
Deriving the effects of trend developments
Literature research on future developments in waste management serves as the basis for deriving the trends. After the subsequent evaluation, trend developments are deduced.
Results and Discussion
At first, the technology supplier’s market study results are reflected, and it is described which technology suppliers make a significant contribution to today’s developments in sensor-based sorting and robotics and the essential products in their portfolio.
Sensor suppliers
In the following, two companies are presented that have contributed to the numerous advances in sorting technology in the field of waste management and recycling (list in alphabetical order):
EVK DI Kerschhaggl GmbH
In addition to applications in the pharmaceutical industry, food processing and mining, this company based in Raaba near Graz also offers sensors for the recycling industry. Sensors are offered for polyethene terephthalate (PET) separation, RDF sorting, bulk material separation in heterogeneous waste and material flows, plastic flake sorting with hyperspectral imaging systems and conductivity imaging technologies. The EVK product portfolio includes colour, hyperspectral and inductive sensor systems. Hyperspectral imaging systems are intelligent camera systems of the ‘Helios’ product range that use spectral ranges adapted to the application (VIS, VIS/NIR, NIR and short-wave infrared “Short Wavelength Infrared” (SWIR)). These systems classify objects according to their chemical composition. EVK offers the possibility of combining this technology with inductive sensors or colour camera systems for sorting, inspection or monitoring purposes [27].
SLOC GmbH
The company has gained a foothold in waste management through cooperation with Saubermacher Dienstleistungs AG in level sensors. In addition to the initial level sensors, information on the location, movement patterns, lid positions, container/ bin openings and fire warnings are also possible in the context of a smart waste bin and rubble bin. The sensors are equipped with computer, power and storage systems independent of the manufacturer. The product portfolio also offers solutions for intralogistics in which forklifts are digitised and smart load carriers are made possible. Lifting height, usage profile, amount counts and load status are information that can be called up [28].
Sorting machine manufacturers
In the following chapter, companies acting worldwide in the manufacture of sensor-based sorting machines are presented and their sensor technologies for waste management sorting tasks are described. Meanwhile, it is reserved for a few established companies to dominate the world market for sensor technologies in the circular economy. Some offer ‘complete packages’ as system planners, whereas the individual components do not necessarily come from the same company. The list below does not claim to be exhaustive as there are other manufacturers on the market. The excluded manufacturers were not mentioned since they only offer a few units (e.g. LIBS) for waste management. Table 3 shows the sensor technologies of the various companies in 2018 [13].

Binder+Co
The machine manufacturer located in Gleisdorf, Austria, offers various processing units and sensor-based sorting machines. These include the CLARITY product line, which sorts recyclable from non-recyclable waste, and the MINEXX line, which is primarily used to process raw materials [29]. Sensor fusion allows sorting by material type and colour simultaneously through a link with specially developed software. In this way, more individual tasks can be solved than simply by combining different sensors [17].
Pellenc
Pellenc Selective Technologies (ST) is part of the Pellenc group. The optical sorters for household and commercial waste are manufactured at the company’s headquarters in Pertuis, France, and sold under the product name MISTRAL+. These systems use NIR, VIS and induction sensors [30].
REDWAVE
Another company that offers sensor-based sorting machines is REDWAVE, a division of BT Wolfgang Binder, located in Gleisdorf, Austria. In addition to sorting plastics, glass and paper, the sorting of minerals is also offered. As a company, REDWAVE is active as a machine supplier and provides system planning [31].
Sesotec
The development and production of the sorting systems of the company founded in 1976 still takes place in Schönberg, Germany, although it now operates globally with seven subsidiaries. Modular sorting systems for plastics, electronic waste, glass, household waste and metal are offered, which combine different sensors, detectors and separators in one device depending on the application. Up to three combined sensors can be used in the recycling systems: a high-resolution line scan camera, nearinfrared sensors and inductive metal detectors. The units are offered with a conveyor belt or a chute [32].
Steinert
The subsidiary Steinert Unisort bundles the resources for the sorting technologies for the waste management of the Steinert Group. The Steinert Group has been based in Köln, Germany, since it was founded in 1889, although there are subsidiaries in the USA, Australia and Latin America [33]. The product range for recycling with NIR is called ‘Unisort’. There is the option of a combination system of up to four sensors in one unit, called the Steinert KSS. The Steinert KSS contains 3D, colour and induction recognition. The fourth installed sensor is either a near-infrared, an X-ray transmission or an X-ray fluorescence sensor. Typical application areas would be separating heavy metal concentrates into copper, brass and grey metals [34].
TOMRA Systems
The company, based in Mülheim-Kärlich, Germany, offers sensor-based sorting solutions for various industries. Formerly TOMRA Sorting was called TiTech, till it was integrated in the Norwegian company TOMRA Systems in 2004. The AUTOSORT product range, which covers almost all waste streams, uses NIR, VIS or induction sensors or a combination of these. Furthermore, besides waste sorting machines and waste collection automats, TOMRA Sorting offers sorting systems for different applications in food or mining [35].”
Sorting robot manufacturers
The future of robotics in waste management has not yet been defined and offers room for innovative ideas. The following is a brief overview of the leading robotics manufacturers in the waste management sector and their current products.
Apple Inc.
Apple revealed their first dismantling robot in 2016. Apple claimed that this robot, called ‘Liam’, could dismantle 1.2 million iPhones 6 per year in eleven seconds each. The further development of ‘Daisy’, which replaced ‘Liam’ in 2018, can dismantle 200 iPhones per hour and differentiate between nine models [36,37]. Although there was a lot of media attention, it must be mentioned that Apple knows the location of recyclables in their devices and how they can be dismantled. This knowledge is usually absent in the everyday waste management business, which struggles with heterogeneity and variable degrees of pollution. The first waste sorting robot entered the market in 2011 from ZenRobotics and uses optical systems [37]. Since then, there have been attempts to use haptic because the sense of touch gives the operator much additional information. Therefore, a robot called ‘RoCycle’ was equipped with capacitive sensors by the Artificial Intelligence (AI) Lab at the Massachusetts Institute of Technology (MIT). It measures size and stiffness by touch. It is not yet a real competition to optical systems because of its low throughput, but combining the sensor systems would be conceivable [38].
OP Teknik
The waste sorting system from OP Teknik specialises in the fully automatic separation of construction and industrial waste into metals, plastic, wood, construction waste, stones and paper. With six robots used, as recommended by the manufacturer, up to 14,400 picks per hour are possible, selected by sensors and cameras in real-time according to material type, colour, size and shape. A single-arm can handle 2,400 picks per hour. For comparison, various manufacturers stated that a person could manage 20 to 40 picks per minute, correspondingly 1,200 to 2,400 picks per hour [39,19].
ZenRobotics
Founded in 2007 and based in Helsinki, Finland, the company was the first to focus on robotics sorting by launching its Heavy Picker in 2009. The robot system with up to three arms contains various detection units such as NIR, VIS and 3D sensors, metal detectors and an RGB camera. The Heavy Picker is designed for heavy and unwieldy objects weighing up to 30 kg. Therefore, it can simultaneously separate up to four different fractions with one arm without extensive pre-sorting or shredding. The various material flows for which it can be used are: commercial and industrial waste, construction and demolition waste, wood, inert materials, plastics, metals (scrap) and different coloured “plastic bags” collected from household waste. The Heavy Picker manages up to 2,000 picks per hour on a conveyor belt controlled by the robot. The AI software that ZenRobotics combines with their products is called Zenbrain [19,40].
The ZenRobotics Fast Picker has a maximum speed of 4,000 picks per hour and consists of an arm with a gripper that works via suction and a sensor unit for the software. This robot is designed for light materials such as packaging waste, dry mixed recyclable materials and household waste with a maximum weight of 1 kg [19,40].
AMP Robotics
The AMP Robotics company from Colorado achieves up to 3,600 picks per hour with its sorting system Cortex. The Cortex system introduced in 2017 uses VIS sensors and machine learning to sort mainly packaging waste. Sorting plants that use this system are mainly found in the USA. Figure 9 shows the basic functional principle: The vision system records data processed using AIbased learning and then sorted by the robot arm [19,41].

Sadako in cooperation with BHS
Since it was founded in 2012, the Spanish company Sadako has focused on artificial intelligence and robotics. Together with BHS - Bulk Handling Systems, their waste sorting system, Max- AI, was used for the first time in California in 2017. Max-AI uses deep learning and an optical system to act as quality control. Using suction mechanisms, up to six different fractions can be recognised simultaneously and sorted with the gripper arm. According to the manufacturer, the system can reach up to 3,900 picks per hour [19,42].
Bollegraaf Recycling Solutions
waste sorting systems for 55 years and has installed over 3,500 recycling systems in Europe, North America, Mexico and Canada. In 2013, the artificial intelligence-provided waste sorting robot RoBB-AQC was presented, separating recyclable materials in the final sorting step. The system is equipped with NIR sensors, an RGB camera and laser units for height detection and separates the detected materials automatically with a suction head. Up to four materials can be sorted out simultaneously per unit at a very high rate of 12,000 picks per hour if an installation with four vacuum grippers is available. However, the materials are mainly paper/ cardboard and various plastics made from mixed waste [19,43]. The Bollegraaf Cogni was presented in 2018 which uses the same technologies as RoBB-AQC and is also mounted on a portable overbelt construction. The suction head is on a delta robotic arm [19,43].
Machinex
The sorting robot from the Canadian company Machinex called SamurAI has been available since 2018 and operates with artificial intelligence from AMP. With one of its four suction heads on four robot arms, it can lift up to 6 kg and manage up to 4,000 picks per hour. SamurAI sorts plastics positively and negatively for quality control or separates from mixed waste. The system is used in nine plants in the USA and Canada and is mainly used to sort plastics. The manufacturer offers software called ‘MACH Vision’. It can create databases for material identification in advance, receive software updates and also use the ‘MACH Cloud’, which can obtain optimisations from other systems [19,44].
Relevant Trends and Developments
This chapter describes the influence of digitalisation and Industry 4.0 on the waste and recycling industry and elaborates on robotics and the sensors used in this industry.
Digital Waste Management
There are various definitions of the term digital transformation. In sum, it can be said that the networking of individual stakeholders (companies, customers, products, etc.) succeeds through using the latest technologies (computers, internet, etc.) and the processing, collection and analysis of information. The so-called 4th Industrial Revolution - better known as Industry 4.0 - thus involves using IT and automation to pass on real-time information to all actors in the value chain and network them [45].
These developments do not stop at the waste management industry. They offer great opportunities, as 63 % of the companies surveyed in a study by the Montanuniversitaet Leoben in cooperation with HTL Leoben confirm. Of the 400 companies surveyed in the green tech sector in German-speaking countries, 75 % said they were involved in digital transformation and 84 % of the companies that were not yet involved said they planned to do so in the future. Moreover, the global circular economy and waste management market volume is expected to rise from around 100 billion euros in 2013 to 170 billion euros by 2025, further encouraging becoming involved in the digital transformation [26,46]. This conclusion is supported by another recently published survey in which 83 % of all surveyed companies announce that they have implemented a company strategy for managing data and 75 % make efforts to ensure high qualities in their transaction data [47].
At the same time, companies in the green tech sector must face new challenges summarised by Roland Berger in 2016 for the German market in five areas. One challenge is securing customer access, as traditional trade is losing importance and sales platforms are becoming increasingly important for customer contact. In order not to lose touch, it is recommended for companies to initiate their platforms and to integrate valueadded partners for system solutions [48].
An example is the ‘Daheim’ app of Saubermacher Dienstleistungs AG, which is available individually designed for 230 municipalities. It implements information and reminder functions for collection calender, which is a Austrian calender that states on which days specific waste streams like light-weight packaging, paper or biological waste are collected by the waste collection system. Furthermore, it is a free communication platform for associations, schools and it offers e-car rental or swap [49].
In order to keep up, the need to increase flexibility and agility to follow the fast innovation cycles is mentioned. One characteristic of agility is to involve customers in the early phases of new developments and to obtain feedback. A further challenge is the development of digital competence. Digital transformation is a cross-sectional undertaking in many areas and does not stop at established processes and structures. In order to exploit the full potential of existing skills, interdisciplinary teams and cloud solutions for rapid information exchange are advantageous. Another recommendation is to adapt financing along the entire value chain. The digital economy relies on intangible assets such as employee know-how, digital strategies and data, whose monetary value is hard to estimate. It is essential to design individual solutions and evaluate the feasibility of renting, leasing, and pay-per-use options. The final challenge of digital transformation is developing the digital mission statement. That means not looking at the challenges individually but developing a comprehensive digitalisation strategy. The digitalisation strategy should be constant evaluation and adaptation to remain proactive [48]. Disruptive innovations in the waste and circular economy are currently taking place and will take place in the future in four identified areas. Collection and logistics face a revolution through ‘smart waste bins’ and intelligent route optimisation. Generally speaking, the customer is coming into focus, with more and more personalisation in terms of collection cycles, for example, which should increase recycling rates [50].
Sensor technology in waste management
With the increased demand for recycled material and increased demands on the quality and purity of this, the pressure on waste management to innovate towards real-time quality control is growing. The goal in the future will undoubtedly be digitised waste treatment, with individual treatment plants communicating with each other and various sensors providing real-time data. For example, to run conveyor belts at the right speed and in turn, adjust pre-treatment equipment such as shredders. Robotics will play a significant role in sorting in combination with real-time statistical modelling, improved object recognition and perpetual optimisation for tasks too dangerous or strenuous for human workers [26].
Quality plays a significant role in recycling plastics for energy use as RDF. Up to now, controls have mainly been carried out manually or by automated sampling. This approach became increasingly impractical due to the delay in the results, as the product had already been manufactured. Various sensors (e.g. NIR sensors) can remedy this situation and determine parameters in the waste stream such as degree of contamination, moisture content, etc. If these parameters are compared with the material group-specific properties in a database or additional parameters such as calorific value, chlorine or ash content are collected, the production line can be adapted in real-time. This fast influence offers the possibility of intervening in the process and optimising the plant to market-specific requirements [19]. In addition to ensure the quality of the RDF, increasing focus is being placed on the recovery of metals to identify and quantify valuable alloying elements [13].
Sorting robots in waste management
In Germany, only 30 % of waste management companies state that they are ready for digital transformation, following the global trend towards green technologies and digitisation [48]. Digitisation and automated processes should help make processes run faster and more precisely by exchanging human work for machine work. In addition, robotics is widely used to reduce human effort. For waste management, only robots for industrial use are considered. Robots differ significantly in their properties, such as speed, gripping system, and the size of their working area or range of the gripper [19]. In the field of waste sorting, collaborative robots (so-called “cobots”) would be conceivable. However, compared to other branches of industry, such as the automotive industry, their use has not yet arrived in waste management. Mechanically separating structures such as fences, light barriers, or laser networks can separate work areas. The latest concepts are based on cooperation and collaboration between man and machine without such restrictions, so robots can directly support employees [20].
With the introduction of Industry 4.0 and the rapidly developing digitisation, more and more applications of robotics technology are emerging. The learning ability of robotic systems means that sorting can be carried out more efficiently. One application of this learning ability is the use of the case-dependent speed of the conveyor belt. Regulating the speed of conveyor belts is a big issue in waste management as the heterogeneity of waste in type, size, and shape has presented a challenge for automated systems. In addition, waste streams have the problem of surface pollution, which impedes detection by sensors. The task for sorting robots includes the need to grasp objects of various shapes and sizes that occur in randomly distributed locations and quantities in the waste stream. Error-free work is also made more difficult by the change in position of objects due to vibrations of the conveyor belt, centrifugal forces or drafts. The computed inverse kinematic of the robotic arm has become wrong since the presumed position of the object has been altered. The failure to grab the object results in misthrows or loss of valuables, which poses the need for continuous monitoring and tracking of objects to be ejected [19].
If the software is connected to the appropriate hardware and artificial intelligence is stored, a robotic system can perform several operations simultaneously and thus perform different sorting tasks. Of course, new waste streams to be sorted out can also be teached in, which makes this technology fundamentally promising, because of the possibility of separating a wide variety of fractions. Figure 10 shows the detection of different materials by the AMP sorting robot Cortex. Robots are used to replace manual sorting or to sort for areas that were previously not sortable [19]. Furthermore, these technologies allow an automatic quality recording and increase sorting efficiencies (e.g. plastics), if necessary. Manual sorting is limited by weight, size and the extension of work environment that robotics is not [19].

The developers do not see robotics as the only future solution for waste treatment and sorting, but especially in packaging and residual waste combined with other technologies such as optical sensors with pneumatic separation. Automated systems are often seen as a quality guarantee at the discharge of a plant. Another topic of utmost importance during the discourse about digitalisation is the protection against cybercrime. The stored software for detecting objects and the associated algorithms are essential and must be adequately protected against manipulation and exploitation. Adequately protecting intellectual property and shielding networks against attacks demands financial resources and know-how [19].
Smart Waste
The circular economy targets stated by the EU and 90 % of the German population agree that waste separation significantly impacts environmental protection. From the consumer’s point of view, the producers or manufacturers of goods substantially influence establishing a well-functioning recycling system. Assuming that these findings can be transferred to Austria, this could explain why the proportions of recyclable materials in the residual waste bin in Styria have hardly changed in the last 20 years. In order to meet the recycling quotas of the EU, citizens must be involved additionally to the expansion of the sorting technology in plants [52,53]. The smart garbage bin, for example, with level measuring sensors from SLOC leads to less traffic, traffic jams, noise and CO2 emissions through dynamic route planning. Austria Glas Recycling expects a potential saving of up to 30 % of the costs and the effort of the collection through the high-tech sensors in public glass containers after the pilot project with Saubermacher in December 2018 in the municipality of Horn in Lower Austria [54]. This example is intended to show that the detection options using sensors, in addition to level measurement, can determine location, movement pattern, several lid openings and a temperature increase of a barrel and thus enable a needbased collection tour planning in addition to increasing comfort for the citizens [12,53].
Recyclable materials scanner
In 2018, the Saubermacher Dienstleistungs AG presented the ‘recyclable material scanner’, a multisensor, multi-spectral image recording system that detects the empty contents of a residual waste bin on the collection vehicle. The generated visual output is displayed in Figure 11. The system evaluates the collected waste in real colours, 3D, and various spectral channels to classify the material. A waste bag opener is used to further increase the visibility of the waste for the convolutional neural network. Test runs have shown that the announcement of the use of this procedure led to a significant reduction in missed throws. Before this announcement, incorrect throws were detected in 65 % of the garbage cans; after the project was publicised, the rate immediately fell to 38 % and could be further reduced through direct feedback. The supplementary resource scanner portal can display and evaluate the detection results and can thus be used as a feedback portal for citizens. The citizens communicate with the disposal company and vice versa via SMS or Saubermacher’s app ‘Daheim’. The proportion of incorrect throws in the municipalities’ residual waste could be reduced by up to 80 %; on average, incorrect throws were halved. The technologies developed in Austria are to be used in another larger region in 2020 [55,56,57].

Smart Villages
The recyclable material scanner and the intelligent waste bin are part of the “Smart Village” project. Energie Steiermark and Saubermacher Dienstleistungs AG included around 150 households in the communities of Riegersburg and Feldkirchen. In addition to the measurements necessary for generating key figures to quantify correct waste separation, street lights were equipped with sensors and vehicles of the road service in winter with GPS route recording and ice sensors. The project was presented in mid-2018, and the first positive results were presented in July 2019, which suggest an expansion of smart technologies in the municipal waste sector [56,57].
Conclusion
The presented data depicts the waste management industry’s rapid developments. While new technologies, like machine learning and convolutional neural networks and robot sorting, are increasingly implemented, a substantial discrepancy exists between technological capabilities and the current State-of-the- Art. Stakeholders in the industry expressed their willingness to adapt their current approaches and implement emerging technologies into their current approaches; these developments take time. Further investments must be made to acquire the knowledge, technology, and human resources needed for such a transmission. These investments need a dependable political and economic foundation to be made, and further political guidelines will be needed to ensure the sustainability of these investments. The increasing attention lawmakers and political institutes currently give to the waste management industry are a welcome enticement to facilitate the implementation of improved sorting technologies in the sector.
When combined with these new emerging technologies, the existing technologies mentioned in this study applied to sensorbased sorting can substantially impact the feasibility of reaching the goal of a circular economy. The active participation in the studies mentioned in this survey reflects the consumers’ and manufacturers’ interest in enhancing current waste management techniques and implementing and adapting to technologies like robotic sorting and applying neural networks for the classification in waste collection. These changes were shown to increase the efficiency of the collection of post-consumer waste by announcement alone. However, while these results motivate further research and these technologies see widespread employment in the automotive and pharmaceutical industry, the operating conditions in this field differ significantly from those in waste management. More comprehensive employment of technologies like robot sorting, live in-line sensor-based measurements of manufacturing and machine learning approaches need to be evaluated in their adaptability to the inherent problems their application in waste management entails.
The employment of these new technologies will need coherent legal and political guidelines. This lack of coherent legal guidelines extends not only to the sector of waste management but to the industrial application of robotics, data science and machine learning in general. Without legal guidelines regulating the liability issues arising from human labourers sharing working space with machines and defining safety regulations adapted to this new development, further growth of this technology will be stunted. It is further to be expected that the emergence of these new technologies will significantly impact the existing labour market, as the need for menial labour is decreasing, and arduous and dangerous jobs may soon be undertaken by machinery. The labour market’s needs will increasingly shift to skilled technicians, able to maintain, program and control the machines substituting the human workforce. Since digitisation and digitalisation are comparable new topics in the waste industry, there is great potential for improvements. The rising interest in this topic is also reflected by the increased market volume of products affiliated with green production and the circular economy, prompting all significant stakeholders in the manufacturing of sorting systems to become involved in applying these emerging technologies in their product portfolio to be on the leading edge of these developments.
Author Contributions
Conceptualization, Karl Friedrich and Daniel Vollprecht; methodology, Karl Friedrich; validation, Karl Friedrich, Theresa Fritz and Gerald Koinig; formal analysis, Karl Friedrich and Gerald Koinig; investigation, Karl Friedrich, Theresa Fritz and Gerald Koinig; data curation, Theresa Fritz and Karl Friedrich; writing - original draft preparation, Karl Friedrich, Theresa Fritz and Gerald Koinig; writing - review and editing, Karl Friedrich and Gerald Koinig; visualization, Karl Friedrich, Theresa Fritz and Gerald Koinig; supervision, Daniel Vollprecht and Roland Pomberger; project administration, Roland Pomberger. All authors have read and agreed to the published version of the manuscript.
Conflicts of Interest
The authors declare no conflict of interest.
References
- European Commission (2019) Implementation of the Circular Economy Action Plan; Report: COM(2019) 190 final.
- Friedrich K, Koinig G, Tschiggerl K, Pomberger R, Vollprecht D (2021a) Challenges to Increase Plastic Sorting Efficiency. International Journal on Engineering Technologies and Informatics 2(4): 114-118.
- European Commission (2018) A European Strategy for Plastics in a Circular Economy; Report: COM(2018) 28 final.
- European Parliament and Council (2019) Directive (EU) 2019/904 on the reduction of the impact of certain plastic products on the environment, L 155/1.
- Global 2000 (2019) Single-Use Plastics Directive - die Einwegplastik-Richtlinie der EU (Single-Use Plastics Directive - the Single-Use Plastics Directive in the EU). (last access: 11 November 2021).
- Beel H (2017) Sortierung von schwarzen Kunststoffen nach ihrer Polymerklasse mit Hyperspectral-Imaging-Technologie (Sorting of black plastics to their polymer types with hyper-spectral-imaging-technology), Berliner Recycling und Rohstoffkonferenz 2017 (Berlin Recycling and Resources Conference 2017), In: Recycling und Rohstoffe Band 10, Berlin, Germany, pp. 175-191.
- Pomberger R, Küppers B (2017) Entwicklungen in der sensorgestützten Sortiertechnik (Developments in sensor-based sorting), Österreichische Abfallwirtschaftstagung 2017 - Die Digitalisierung der Abfallwirtschaft (Austrian Waste Management Congress 2017 – Digitalisation of Waste Management), In Proceedings of the Austrian Waste Management Congress 2017, ÖWAV: Vienna, Austria, Module 9.
- Uepping R (2013) Sensorgestützte Sortiertechnik (Sensor-based Sorting Technology), Berliner Recycling und Rohstoffkonferenz 2013 (Berlin Recycling and Resources Conference 2013), In Recycling und Rohstoffe Band 6, Berlin, TK Verlag: Neuruppin, Germany, pp. 371-383.
- Pretz T, Julius J (2008) Stand der Technik und Entwicklung bei der berührungslosen Sortierung von Abfällen (State-of-the-Art and development in contactless sorting of waste). Österr Wasser- und Abfallwirtschaft 60, ÖWAV: Vienna, Austria, pp 105-112.
- Wotruba H (2008) Stand der Technik der sensorgestützten Sortierung (State-of-the-Art in sensor-based sorting). Berg- und Hüttenmännische Monatshefte, Volume 153, Heft 6, Springer-Verlag: Heidelberg, Germany, pp. 221-224.
- Lydit (2021) Terahertz, the last frontier of photonics. (last access: 11 November 2021).
- König, M (2019) Innovative Detektionstechnik und gezielte Kommunikationsstrategie zur Beeinflussung des Wegwerfverhaltens in der österreichischen Abfall- und Ressourcenwirtschaft (Innovative detection technology and targeted communication strategy to influence the throw-away behavior in the Austrian waste and resource management), Master’s Thesis, FH Wien der WKW, Vienna, Austria.
- Flamme S, Hams S, Zorn M (2018) Sensortechnologien in der Kreislaufwirtschaft (Sensor technologies in Circular Economy), Recy- & Depotech 2018, In Proceedings of the 14th Recy- & DepoTech Conference, 07 November - 09 November 2018, Leoben, AVAW Eigenverlag: Leoben, Austria, pp. 787-792.
- Küppers B, Möllnitz S (2018) Versuchsstand für sensorgestützte Erkennung und Sortierung (Experimental sensor-based sorting setup). DGAW-Wissenschaftskongress„ Abfall- und Ressourcenwirtschaft“ (8th DGAW Science Congress „Waste and resource economy“), In Proceedings of the 8th DGAW Science Congress „Waste and resource economy“,Vienna, innsbruck university press: Innsbruck, Austria, pp. 211-215.
- Aderhold J, Brüning R, Dittrich PG, Grählert W, Hubold M, et al. (2019) Grundlagen und Methoden des HSI (Basics and methods of HSI), Chapter 2 in Leitfaden zur hyperspektralen Bildverarbeitung (Hyperspectral Imaging Guide), Volume 19, Fraunhofer-Verlag: Stuttgart, Germany, 2019, pp. 15-34,
- Luxflux (2018) Die nächste Stufe der industriellen Bildverarbeitung (The next step in industrial image processing). (last access: 11 November 2021).
- Binder+Co (2021a) Sensorbasierte Sortiertechnologie mit höchster Präzision (Sensor-based sorting technology with highest precision). (last access: 11 November 2021).
- Inc (2020) Robotics. (last access: 11 November 2021).
- Sarc R, Curtis A, Kandlbauer L, Khodier K, Lorber KE, et al. (2019) Digitalisation and intelligent robotics in value chain of circular economy-oriented waste management - A review. Waste Management 95: pp. 476-492.
- TÜV AUSTRIA Group, Fraunhofer Austria Research GmbH (2016) Sicherheit in der Mensch-Roboter Kollaboration: Grundlagen, Herausforderungen, Ausblick (Safety in human-robot collaboration: basics, challenges, outlook), White Paper. (last access: 11 November 2021).
- Stark G (2009) Robotik mit MATLAB (Robotics with MATLAB). Carl-Hanser-Verlag: München, Germany, pp. 16-17.
- Dokulil E (2001) PC News 72, April 2001, PCNEWS-Eigenverlag: Vienna, Austria, pp. 58-64.
- Gerke W (2015) Technische Assistenzsysteme: Vom Industrieroboter zum Roboterassistenten, De Gruyter Oldenbourg: Berlin, Germany, pp. 147-148.
- Induux (2021) Industrieroboter - Typen Aufbau Anwendungen Auswahl (Industrial robots – Types, design, applications, selection). (last access: 11 November 2021).
- IFR - International Federation of Robotics (2018) Welcome to the IFR Press Conference. World Robot Summit, Presentation of the IFR Press Conference, Tokyo, Japan. (last access: 18 January 2022).
- Green Tech Cluster (2018) Digitale Abfallwirtschaft: Mehrwert entlang der gesamten Wertschöpfungskette (Digital Waste Management: Added value along the whole value chain), Green Tech Radar. (last access: 18 January 2022).
- EVK (2021) Products. (last access: 18 January 2022).
- SLOC (2021) SLOC Product Portfolio. (last access: 18 January 2022).
- Binder+Co (2021b) Products - Sensor-based Sorting.
- Pellenc (2021) Products. (last access: 18 January 2022).
- REDWAVE (2021) REDWAVE solutions. (last access: 18 January 2022).
- Sesotec (2021) Sorting systems. (last access: 18 January 2022).
- Steinert (2021a) Steinert - Our locations. (last access: 18 January 2022).
- Steinert (2021b) Steinert - Sensor Sorting. (last access: 18 January 2022).
- TOMRA (2021) TOMRA – Recycling. (last access: 18 January 2022).
- Apple (2018) Apple adds Earth Day donations to trade-in and recycling program, Press Release. (last access: 18 January 2022).
- EU Recycling (2018) Abfallsortierung der Zukunft: durch Mensch oder Roboter? (Waste sorting in the future: By hand or by robot?), EU-Recycling, p. 10. (last access: 18 January 2022).
- Heaven D (2019) Recycling-Roboter sortiert durch Tasten (Recycling robots sorts by touch), MIT Technology Review. (last access: 18 January 2022).
- OP Teknik (2021) SELMA robot sorting. (last access: 18 January 2022).
- ZenRobotics (2021) Robotic Waste Recycling Solutions. (last access: 18 January 2022).
- AMP Robotics (2021a) AMP Cortex – Intelligent Robots for Recycling. (last access: 18 January 2022)
- BHS (2021) MAX AI. (last access: 18 January 2022).
- Bollegraaf (2021) Robotic Sorting. (last access: 18 January 2022).
- Machinex (2018) SamurAI. (last access: 18 January 2022).
- Erhart W (2017) Digitale Geschäftsmodelle und schnelle Innovationszyklen in der traditionellen Industrie; Am Beispiel: Konzeptionierung und Implementierung eines digitalen, mehrseitigen Geschäftsmodells in der Verwertungs- und Entsorgungsbranche (Digital business models and fast innovation cycles in traditional industries; Example: Conception and implementation of a digital, versatile business model in the recycling and disposal industry). Master’s Thesis, Campus 02 - University of Applied Sciences for Economy, Graz, Austria.
- Sarc R, Hermann R (2018) Unternehmensbefragung zum Thema Abfallwirtschaft 4.0 (Company survey on waste management 4.0), Recy- & Depotech 2018, In Proceedings of the 14th Recy- & DepoTech Conference, 07 November - 09 November 2018, Leoben, AVAW Eigenverlag: Leoben, Austria, pp. 805-812.
- Friedrich K, Fritz T, Koinig G, Pomberger R, Vollprecht D (2021b) Assessment of Technological Developments in Data Analytics for Sensor-Based and Robot Sorting Plants Based on Maturity Levels to Improve Austrian Waste Sorting Plants. Sustainability 13(16): 9472.
- Berger R (2016) Die Digitalisierung in der GreenTech-Branche; Handlungsbedarf für Unternehmen der Umwelttechnik und Ressourceneffizienz in Deutschland (Digitalisation in the Green Tech Branche; Need for action for companies in environmental technology and resource efficiency in Germany), Bundesministerium für Umwelt, Naturschutz, Bau und Reaktorsicherheit.
- Saubermacher (2019) Daheim App. (last access: 18 January 2022).
- Klemmer O (2019) Digitalisation potential analysis for sustainable waste management, Master's Thesis, TU Vienna and Diplomatic Academy of Vienna, Vienna, Austria.
- Continuous Improvement Fund (2021) Robot combines Hyperspectral vision with Artificial Intelligence for residential recycling. (last access: 18 January 2022).
- Verbraucherzentrale Bundesverband (2015) Ergebnisse der bevölkerungsrepräsentativen Umfrage zum Thema "Wertstofftonne" (Results of the population representative survey on the subject of "valuable material bin"), forsa., Berlin, Germany. (last access: 18 January 2022).
- Mittermayr R, Klünser S (2019) Smart Waste - Wie Digitalisierung und IoT die Welt des Abfalls verändern können (Smart Waste - How digitalisation and IoT can change the world of waste), Berliner Recycling- und Rohstoffkonferenz 2019 (Berlin Recycling and Resources Conference 2019), In Recycling und Rohstoffe Band, Berlin, TK-Verlag: Neuruppin, Germany, pp. 15-24.
- Green Tech Cluster (2019) Erfolgreiche Smart-Waste Projekte (Successful smart-waste projects). (last access: 18 January 2022)
- FG Entsorgungs- und Ressourcenmanagement, WKO Steiermark (2019) Rohstoff, Smart Waste: Erfolgreiche Mülltrennung mit Künstlicher Intelligenz (Raw material, Smart waste: Successful waste separation with artificial intelligence), p. 27. (last access: 18 January 2022).
- ORF Steiermark (2018) Gemeinden starten Pilotprojekt "Smart Village" (Municipalities start pilot project "Smart Village").(last access: 18 January 2022).
- Piron R (2019) Erste Smart Village - Projekte zeigen Erfolge (The first Smart Village projects are showing success), KOMMUNAL. (last access: 18 January 2022).
- Saubermacher Dienstleistungs AG – Ecoport (2018) Der Wertstoffscanner von Saubermacher (The recyclable material scanner from Saubermacher), Video: Der Wertstoffscanner von Saubermacher. (last access: 18 January 2022).